ABSTRACT
(1) Background: BET bromodomain proteins regulate transcription by binding acetylated histones and attracting key factors for, e.g., transcriptional elongation. BET inhibitors have been developed to block pathogenic processes such as cancer and inflammation. Despite having potent biological activities, BET inhibitors have still not made a breakthrough in clinical use for treating cancer. Multiple resistance mechanisms have been proposed but thus far no attempts to block this in glioma has been made. (2) Methods: Here, we have conducted a pharmacological synergy screen in glioma cells to search for possible combination treatments augmenting the apoptotic response to BET inhibitors. We first used HMBA, a compound that was developed as a differentiation therapy four decades ago but more recently was shown to primarily inhibit BET bromodomain proteins. Data was also generated using other BET inhibitors. (3) Results: In the synergy screen, we discovered that several MEK inhibitors can enhance apoptosis in response to HMBA in rat and human glioma cells in vitro as well as in vivo xenografts. The combination is not unique to HMBA but also other BET inhibitors such as JQ1 and I-BET-762 can synergize with MEK inhibitors. (4) Conclusions: Our findings validate a combination therapy previously demonstrated to exhibit anti-cancer activities in multiple other tumour types but which appears to have been lost in translation to the clinic.
Introduction
Before the discovery of oncogenes, the concept of cancer cell differentiation therapy was explored therapeutically, in part based on early observations that dimethylsulfoxide (DMSO) can cause differentiation of Friend virus-induced mouse erythroleukemia (MEL) cells into haemoglobin producing red blood cells [Citation1]. Efforts to produce more potent cancer differentiation compounds generated two molecules that were tested in the clinic, hexamethylene bisacetamide (HMBA) and suberoylanilide hydroxamic acid (SAHA, later renamed to vorinostat) [Citation2,Citation3]. Whereas SAHA was found to inhibit histone deacetylases (HDACs) 1–3 and made it to clinical approval for cutaneous T-cell leukaemia, HMBA neither inhibits HDACs nor received clinical approval, and its target was unknown for 40 years [Citation4,Citation5]. Recently, however, we discovered that HMBA is a bromodomain and extra-terminal domain (BET) inhibitor, with highest binding affinity for bromodomain 2 (BD2) of BET proteins BRD2, BRD3, and BRD4 while also inhibiting the bromodomain of histone acetyltransferase P300 [Citation6]. The structure of HMBA largely resembles that of an acetylated lysine, explaining the mode of action.
Although HMBA was likely the first anti-cancer compound used in the clinic that inhibited BET bromodomain proteins, the concept of BET inhibitors (BETis) were largely popularized with the development of the low nanomolar BETis JQ1 and iBET-151 [Citation7,Citation8]. The mechanism of action of these compounds involves inhibition of transcriptional elongation [Citation9,Citation10]. Albeit that MYC transcription is frequently suppressed, all effects of BETis are not dependent on MYC suppression [Citation11,Citation12]. Most of the clinical studies using HMBA and other BETis have focused on haematological malignancies and less is known about the effect of this class of compound in solid tumours such as glioma. Haematological malignancies respond to BETis in vitro by cell cycle arrest, differentiation, and apoptosis, whereas glioma cells undergo cell cycle arrest and differentiation and to a lesser extent apoptosis [Citation13–15]. Importantly for glioma treatments, the clinical BET inhibitor OTX015 has been shown to pass the blood-brain-barrier [Citation16], and HMBA can cause dose-limiting toxicities in CNS, suggesting it is also brain-penetrant [Citation17–19].
If BETis are to work in the clinic against solid tumours including glioma, then the predominant effect of BETis should be cell death such as apoptosis or possibly senescence. So far, BETis have not convincingly shown cell death as single agents in solid tumours. Here we use the C6 rat glioma model system to study means to activate cell death in BETi-treated cells. We demonstrate that the MAPK pathway is critical for maintaining viability of HMBA-treated C6 cells and demonstrate synergy between HMBA and MEK inhibitors in vitro and in mouse xenograft experiments using C6 cells and human primary glioma sphere cultures.
Results
To study the effect of HMBA in glioma we used the rat glioma cell line C6. Treatment of these cells for 72 h blocked cell proliferation ()) but did not induce cell death, as assessed by flow cytometry of sub-G1 DNA content for apoptosis, or decrease in cell number from the treatment start (). We therefore conclude that C6 glioma cells primarily respond to HMBA by growth inhibition. We hypothesized that a signalling pathway targeted by drugs could be used by the cell to maintain viability upon HMBA treatment. We therefore screened a library of 226 compounds (Supplemental Table S1) either approved for clinical use or under various stages of clinical development. Comparing the effect of monotherapy of HMBA, with monotherapy of either library compound alone or with combination therapy of HMBA and library compound, we identified compounds that displayed synergistic effects together with HMBA, of which three were MEK inhibitors ()).
Figure 1. HMBA evokes primarily growth arrest in C6 glioma cells. a) Cell counts using trypan blue and b) DNA histograms of 7-AAD-stained nuclei quantifying the sub-G1 content together indicate that the primary response to HMBA-treatment in C6 glioma cells is growth arrest. c) Quantification of cells with less than diploid DNA content in b). d) Plot summarizing the results from the pharmacological screen of HMBA in combination with 226 different compounds. The three red dots indicate the three MEK inhibitors which all fall below the line of equal measured and expected
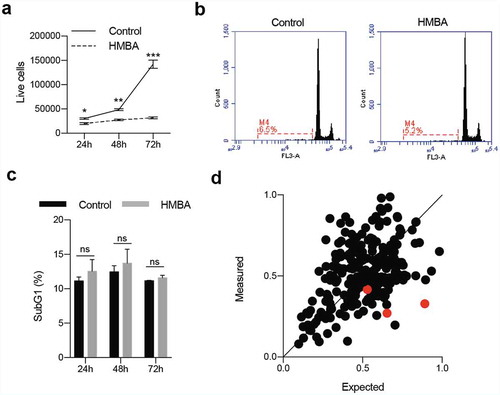
Figure 2. Combination of BET inhibitors and MEK inhibitors enhance cell death in C6 rat glioma cells. a) Clonogenic assay of C6 treated with HMBA, trametinib or the combination of both. b) Cell viability of single treatment or combination using Cell Titre Glo. The dotted indicates the expected value of an additive effect of the combination c) Percent of cells with less than diploid DNA content (sub-G1). The high rates in combination treatment could be suppressed with pan-caspase inhibitor Q-VD-OPH, suggesting apoptosis. d) and e) Viability and sub-G1 assessments of combinations of BET inhibitors HMBA, JQ1 and I-BET762 together with MEK inhibitors trametinib, TAK733, AZD8330 and binimetinib. Single asterisks or hash signs indicate significant values of p < 0.05, double signs are p < 0.01, triple signs are p < 0.001 and quadruple signs are p < 0.0001
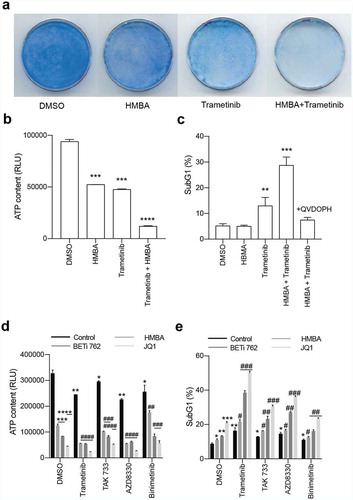
Currently, two MEK inhibitors are FDA approved for use in melanoma but none are used for the treatment of glioma. We repeated the results from the library screen using the FDA-approved MEK-inhibitor trametinib (GSK1120212) in a clonogenic assay ()). The lack of long-term growth and induction of cell death was revealed by an ATP/luciferase-based viability assay ()) and flow cytometric analysis of sub-G1 DNA content ()). This cell death was likely mediated by caspases since the sub-G1 content of the cells could be rescued by the pan-caspase inhibitor Q-VD-OPH. Furthermore, the synergistic effects of dual BET and MEK inhibition could be reproduced using other MEK inhibitors (TAK-733 and AZD8330, but not binimetinib) and BETi (JQ1 or iBET-762; and Supplemental Figure S1A-B).
Earlier studies had indicated that HMBA could more efficiently induce differentiation of a vincristine-resistant leukaemia cell line [Citation20]. This suggested that HMBA possibly could interfere with drug resistance pump such as p-glycoprotein (ABCB1 or MDR1) but such a link could not be established. On the other hand, trametinib had previously been shown to be a substrate of p-glycoprotein (p-gp) [Citation21] so we reasoned that p-gp could be involved in the synergy in C6 glioma cells. Indeed, as we have previously shown [Citation22], C6 cells were highly effective in pumping out the substrate rhodamine 123, and this activity was blocked by the ABCB1/ABCG2 inhibitor elacridar ()). Interestingly, also trametinib – but not the other MEK inhibitors tested – could inhibit pumping of rhodamine 123 but only occasionally at 1 µM and more prominently at >1 µM (Supplemental ). Blocking of pumps with elacridar reduced the concentration needed to inhibit ERK phosphorylation in C6 cells ()). However, the fact that elacridar neither synergized with trametinib nor with HMBA or JQ1 () made it unlikely that BETis synergize with MEK inhibitors because of regulation of p-gp or other drug pumps. Rather, as HMBA and JQ1 synergize with other MEK inhibitors () – which were not p-gp inhibitors (Supplemental ) – and also with the ERK inhibitor SCH772984 (), suggests that the MAPK pathway maintains the viability of BETi-treated C6 glioma cells.
Next, we investigated if HMBA and trametinib could block tumour growth in vivo. Immuno-compromised NOG mice were transplanted with C6 cells subcutaneously, and when tumours were palpable they were randomized to treatment either with normal food or food containing trametinib and/or normal drinking water or drinking water supplemented with HMBA. Tumours in mice treated with HMBA in drinking water or trametinib in the food grew significantly slower than tumours growing in control mice and in HMBA/trametinib-treated mice tumour growth was robustly suppressed resulting in four-fold longer survival ().
Figure 3. Inhibiting p-gp activity with elacridar affects trametinib activity but does not synergize with trametinib to kill C6 cells. a) Flow cytometry analysis for p-gp activity showing p-gp substrate Rhodamine 123 being pumped out of cells (DMSO, bottom panel whereas inhibiting p-gp with elacridar blocks this pumping (Elacridar, bottom panel). b) Blocking p-gp with elacridar enhanced the activity of trametinib as judged by lowered P-ERK on Western blot. Values of relative expression to actin and the control, assessed by densitometry, is below images. Uncropped images are in Supplemental Figure S2. c) Viability assay showing that inhibiting p-gp with elacridar does not enhance killing by trametinib. d) Viability assay of combination treatments with elacridar and HMBA or JQ1. e) and f) Viability and sub-G1 assessments of combinations of BET inhibitors HMBA and JQ1 together with ERK inhibitor SCH772984
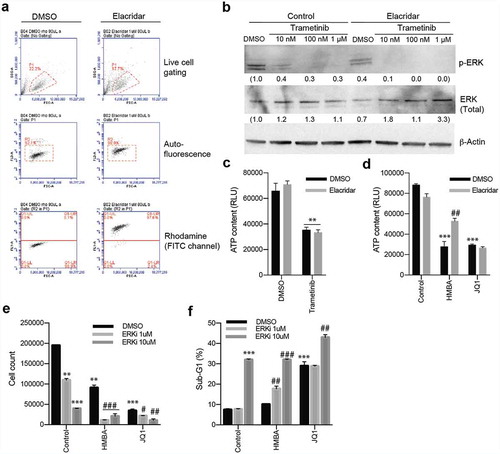
Figure 4. Treatments in vivo of C6 glioma with HMBA (2.5% HMBA in drinking water), trametinib (0.5 mg/kg mouse mixed in food) or combination. a) Tumour volumes over time with respective treatment. Significance of curve comparisons (asterisks) are made for vehicle and trametinib+HMBA treated mice. b) Survival curve indicating the elapsed time for tumours in the different treatment to reach ethical size limit, n = 4 in each group
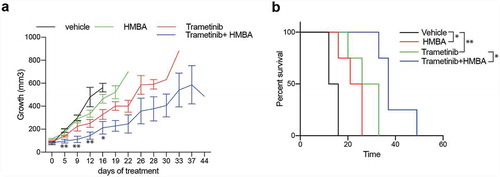
To gain insight into whether or not the described synergy effect of BET and MEK inhibition would also impact on human glioma we treated four patient-derived glioma sphere cultures with HMBA and trametinib. Three out of the four cell lines had some response to trametinib in short-term sphere culture but only one out of the four cell lines, NCH421K, was sensitive to the combination, suggesting that multiple pathways maintain the viability of human glioma cells treated with HMBA ()). However, long-term adherent culture of NCH644 and NCH690 revealed sensitivity to the combination ()). Nevertheless, treatment of mice bearing NCH421K tumours with HMBA water and trametinib food suppressed growth ()). Trametinib has been associated with induction of kinase activities in triple-negative breast cancer cells through enhancer remodelling [Citation23]. Presumably, this could help the cells survive MEK inhibition, which would be perturbed by BETi treatment if these kinases rely on BET protein-regulated processes for expression. In order to investigate if this also holds true in glioma, we performed phosphokinase arrays on two of the human glioma lines, NCH644 and NCH690. The analysis included 43 phosphorylation sites of known kinases. After 24 h treatment, there were minor effects on kinase activities in the two cell lines (Figure S3). The graphs display the ratios of trametinib vs vehicle of each cell line. CREB is a kinase that is deregulated in glioma, is phosphorylated by MEK and is essential for gliomagenesis [Citation24–27]. Reassuringly, ERK phosphorylation and phosphorylation of the ERK target CREB was inhibited in both cell lines, confirming the activity of the MEK inhibitor. Glioma line NCH690 exhibited a general downregulation of all kinase activities tested in the assay, in accordance with the overt sensitivity of this cell line to monotherapy with trametinib ()). The NCH644 line, on the other hand, was less affected by monotherapy with trametinib ()) and phosphorylation of for example c-Jun, FYN, and PRAS40 was induced by trametinib (Figure S4). Collectively, our data does not provide a consistent view on changes of the phospho-proteome in glioma cells treated with trametinib, besides inhibition of ERK phosphorylation.
Discussion
In the present study, we have identified means to enhance the efficacy of BETis in models of glioma. Several BETis have already entered clinical trials, e.g., HMBA, OTX015, BAY 1,238,097 and ABBV-075 [Citation28–30], but thus far the therapeutic effect of these inhibitors as monotherapies have been sparse. Our findings that MEK inhibitors, which are already available in clinical use, could synergize with BETis is therefore of clinical interest. Notably though, the synergistic effect of simultaneous targeting with BET and MEK inhibitors has also been observed in a broad set of tumour types [Citation23,Citation31–35]. The sensitivity has been correlated to certain mutational states, like Suz12 loss in malignant peripheral nerve sheath tumours [Citation31] which leads to an epigenetic switch from histone methylation to histone acetylation, rendering the tumours sensitive to BET inhibitor JQ1. Another study demonstrated that resistance to MEK inhibitors associated with BRD4-induced enhancer formation, which could be inhibited by JQ1 [Citation23]. Although the combination therapy can show effects in many tumour types, it is not certain that the mechanism will be identical in all affected tumour types since the transcriptional effects of BET inhibition are very pronounced.
MEK/BET combination inhibition can suppress MAPK and checkpoint inhibitor-resistant melanoma in animal models including those exhibiting NRAS mutations [Citation34]. Although BET and MEK inhibitors would be expected to have effects on normal lymphocytes as well, the combination had activity also in immune-competent mice and did not impair immune cells. However, in these experiments, checkpoint inhibitors were not given which could explain the general insensitivity of the non-dividing immune cells to BET/MEK combination treatment. Some of these concerns could be investigated in novel-humanized mouse models developed by us for, e.g., melanoma [Citation36,Citation37]. Unfortunately, we could not use these here since they require access to autologous or HLA-matched immune cells.
We have previously published data demonstrating that BETis act as what historically was referred to as cancer differentiating agents [Citation6,Citation11]. Tumour cell differentiation therapies held great promise during the 1980s and 1990s but did not render any clinically approved therapies for solid tumours. The vast literature, including our study, on combining BET inhibitors with MAPK inhibitors, could be a solution to enhancing the effects of previously tested differentiation therapies. Glioma patients have very few viable treatment options for advanced disease and therefore could participate in phase 1 studies on the combination between BETis and, e.g., trametinib. A possible challenge may be that trametinib appears to be a substrate of drug pumps [Citation21] but this has to be investigated in animal models where the tumour is grown in the brain and later be validated in the clinic. Additional experiments are also needed in the future to address mechanisms of resistance to BET and MEK that can be targeted or excluded by correct patient stratification strategies.
Materials and methods
Chemicals
HMBA and Rhodamine 123 were purchased from Sigma-Aldrich. A collection of 226 anti-cancer compounds under clinical development or in clinical use as well as AZD8330, I-BET-762, trametinib, TAK733, binimetinib, elacridar, and ERK inhibitor SCH772984 were all from Selleck Biochemicals. The (+)-enantiomer of JQ1 was purchased from Cayman chemicals.
Cell culture
The rat glioma cell line C6 was bought from Cell Line Service (CLS) and grown in RPMI-1640 supplemented with 10% FBS, GlutaMAX, and antibiotics. The human glioma sphere cultures NCH412K, NCH612, NCH644, and NCH690 were form CLS and were cultured according to the company’s recommendations in glioma sphere medium MG43 (CLS) as spheres or adherent cultures using laminin-coated plastic dishes. Viability after treatments was analysed with Cell Titer Glo (Promega), or Coomassie-staining of cells grown for clonogenic assay.
Mouse experiments
All animal experiments were performed in accordance with regional/local animal ethics committee approval (approval number 36/2014). C6 or NCH412K cells were injected subcutaneously onto the flanks of immunocompromised, non-obese severe combined immune-deficient interleukin-2 chain receptor γ knockout mice (NOG mice; Taconic, Denmark). Tumours were measured with caliper at regular time points and tumour volumes were calculated using the formula: tumour volume (mm3) = (length(mm)) × (width(mm))2/2. Treatments were started when the tumours were actively growing, judged by increasing volumes on repeated caliper measurements. Trametinib was mixed in the chow at 2.5 mg/kg giving an approximate dose of 0.5 mg/kg mouse per day. HMBA was given in drinking water as 2.5% HMBA, 0.33 g/L bicarbonate, 2% sucrose. Vehicle was given as 0.33 g/L bicarbonate, 2% sucrose. Mice were sacrificed and tumours were harvested before or when tumours reached ethical size limit.
Cell cycle analysis
One million cells per mL were lysed and stained for 30 minutes at 37°C in modified Vindelöv’s solution (20 mM Tris, 100 mM NaCl, 1 μg/mL 7-AAD, 20 μg/mL RNase, and 0.1% NP40 adjusted to pH 8.0) followed by the analysis of DNA content using the FL3 channel (linear mode and cell cycle) or FL3 channel (logarithmic mode and apoptosis) with a BD Accuri C6 flow cytometer.
Western blot
For western blot analysis of protein expression, cell pellets or tumour pieces were lysed in lysis buffer (50 mM HEPES pH 7.5, 150 mM NaCl, 1 mM EDTA, 2.5 mM EGTA, 0.1% Tween-20, 1 x HALT protease and phosphatase inhibitors (Thermo Scientific)) on ice. After sonication and clearing of lysates, protein was determined using Bio-Rad Protein Assay Dye reagent (Bio-Rad). A total of 50 μg of protein was resolved on 4–20% Mini-PROTEAN TGX gels (Bio-Rad) and transferred to nitrocellulose membrane (Protran, GE Healthcare Bio-Sciences). Membranes were stained with Ponceau S red dye to verify equal loading. All subsequent steps were carried out in TBS-Tween (10 mM Tris-HCl, pH 7.6, 150 mM NaCl, and 0.05% Tween-20) containing 5% bovine serum albumin for antibody incubations. Antibodies against total ERK and phosphorylated ERK were from Cell Signalling, beta-Actin was from Sigma. For phosphorylation site detection the Proteome profiler human phospho-kinase array kit (R&D Systems) was used according to manufacturer’s instructions. Lysates were prepared the same way as described above and 200 μg total protein was incubated with each membrane set. The signals were quantified using densitometry.
Figure 5. Human glioma cells are sensitive to combination of HMBA and trametinib. a) Viability assay of four human glioma cell lines treated for three days as spheres with HMBA and varying concentrations of trametinib. b) Clonogenic assay of cell lines NCH644 and NCH690 growing adherently on plastic showing potency of combination treatment. c) Tumour growth in vivo of NCH421K cells growing subcutaneously on NOG mice and treated with combination of HMBA+trametinib
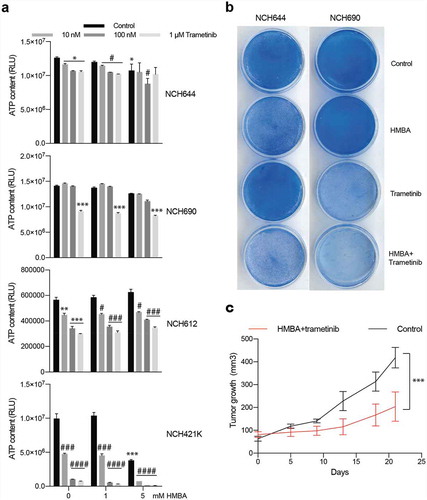
Analysis of pump activity
C6 cells were treated for 48 h with indicated inhibitor, after which they were further treated in the presence of 200 ng/mL Rhodamine 123 for 60 min. After incubation, the cells were washed with PBS and cultured for another 90 min in fresh medium with continued treatment but in the absence of Rhodamine 123. Elacridar was added (1uM) to block pumping of Rhodamine 123. Cells were harvested and resuspended in PBS and analysed with a BD Accuri C6 flow cytometer.
Statistical analysis
Graphs were generated using GraphPad Prism, error bars on tumour growth curves are shown as standard error of mean (SEM), and error bars on cell experiments are shown as standard deviation (SD). Statistical significance was assessed by Student’s T test and significant values compared to vehicle are indicated by asterisks whereas significant values compared to relevant monotherapy in combination experiments are indicated by hash signs. Single asterisks or hash signs are p < 0.05, double signs are p < 0.01, triple signs are p < 0.001 and quadruple signs are p < 0.0001. Survival curve analysis for in vivo experiments was performed using the log-rank (Mantel-Cox) test in Graph Pad Prism (GraphPad Software).
Conclusions
The present study confirms in an additional cancer type that targeting BET bromodomain protein and MEK is more effective than monotherapies of both inhibitors. We propose the initiation of a basket clinical trial for patients with solid tumours that have failed targeted therapies and/or immunotherapies.
Significance
Our findings validate a combination therapy previously demonstrated to exhibit anti-cancer activities in multiple other tumour types but which appears to have been lost in translation to the clinic.
Author contributions
The following were made: Conceptualization, J.A.N. and L.M.N.; methodology, E.F.B, D.V.H., and L.M.N.; data curation, E.F.B., J.A.N., L.M.N.; writing—original draft preparation, E.F.B., J.A.N., L.M.N.; writing—review and editing, E.F.B, D.V.H., J.A.N., L.M.N; supervision, J.A.N., and L.M.N.
Supplemental Material
Download Zip (1.2 MB)Acknowledgments
We dedicate this study to the late Dr Paul A Marks, who developed the HMBA molecule. We thank Sofia Stenqvist and Carina Karlsson for technical support.
Disclosure statement
No potential conflict of interest was reported by the author.
Supplementary material
Supplemental data for this article can be accessed here.
Additional information
Funding
References
- Friend C, Scher W, Holland JG, et al. Hemoglobin synthesis in murine virus-induced leukemic cells in vitro: stimulation of erythroid differentiation by dimethyl sulfoxide. Proc Natl Acad Sci U S A. 1971;68(2):378–382.
- Reuben RC, Wife RL, Breslow R, et al. A new group of potent inducers of differentiation in murine erythroleukemia cells. Proc Natl Acad Sci U S A. 1976;73(3):862–866.
- Richon VM, Webb Y, Merger R, et al. Second generation hybrid polar compounds are potent inducers of transformed cell differentiation. Proc Natl Acad Sci U S A. 1996;93(12):5705–5708.
- Marks PA, Breslow R. Dimethyl sulfoxide to vorinostat: development of this histone deacetylase inhibitor as an anticancer drug. Nat Biotechnol. 2007;25(1):84–90.
- Richon VM, Emiliani S, Verdin E, et al. A class of hybrid polar inducers of transformed cell differentiation inhibits histone deacetylases. Proc Natl Acad Sci U S A. 1998;95(6):3003–3007.
- Nilsson LM, Green LC, Veppil Muralidharan S, et al. Cancer differentiation agent hexamethylene bisacetamide was likely the first BET bromodomain inhibitor in clinical trials. Cancer Res. 2016. DOI:10.1158/0008-5472.CAN-15-2721
- Filippakopoulos P, Qi J, Picaud S, et al. Selective inhibition of BET bromodomains. Nature. 2010;468(7327):1067–1073..
- Nicodeme E, Jeffrey KL, Schaefer U, et al. Suppression of inflammation by a synthetic histone mimic. Nature. 2010;468(7327):1119–1123..
- Dawson MA, Prinjha RK, Dittmann A, et al. Inhibition of BET recruitment to chromatin as an effective treatment for MLL-fusion leukaemia. Nature. 2011;478(7370):529–533..
- Delmore JE, Issa G, Lemieux M, et al. BET bromodomain inhibition as a therapeutic strategy to target c-Myc. Cell. 2011;146(6):904–917..
- Bhadury J, Nilsson LM, Muralidharan SV, et al. BET and HDAC inhibitors induce similar genes and biological effects and synergize to kill in Myc-induced murine lymphoma. Proc Natl Acad Sci U S A. 2014;111(26):E2721–30.
- Lockwood WW, Zejnullahu K, Bradner JE, et al. Sensitivity of human lung adenocarcinoma cell lines to targeted inhibition of BET epigenetic signaling proteins. Proc Natl Acad Sci U S A. 2012;109(47):19408–19413.
- Shirsat NV, Kayal JJ, Shaikh S, et al. Growth inhibition and differentiation of C6 glioma cells on treatment with hmba. Cell Biol Int. 2001;25(7):621–627.
- Li XN, Du ZW, Huang Q. Modulation effects of hexamethylene bisacetamide on growth and differentiation of cultured human malignant glioma cells. J Neurosurg. 1996;84(5):831–838.
- Cheng Z, Gong Y, Ma Y, et al. Inhibition of BET bromodomain targets genetically diverse glioblastoma. Clin Cancer Res. 2013;19(7):1748–1759.
- Berenguer-Daize C, Astorgues-Xerri L, Odore E, et al. OTX015 (MK-8628), a novel BET inhibitor, displays in vitro and in vivo antitumor effects alone and in combination with conventional therapies in glioblastoma models. Int J Cancer. 2016;139(9):2047–2055.
- Rowinsky EK, Ettinger DS, Grochow LB, et al. Phase I and pharmacologic study of hexamethylene bisacetamide in patients with advanced cancer. J Clin Oncol. 1986;4(12):1835–1844.
- Ward FT, Kelley JA, Roth JS, et al. Phase I bioavailability and pharmacokinetic study of hexamethylene bisacetamide (NSC 95580) administered via nasogastric tube. Cancer Res. 1991;51(7):1803–1810.
- Young CW, Fanucchi MP, Declan Walsh T, et al. Phase I trial and clinical pharmacological evaluation of hexamethylene bisacetamide administration by ten-day continuous intravenous infusion at twenty-eight-day intervals. Cancer Res. 1988;48(24 Pt 1):7304–7309.
- Melloni E, Pontremoli S, Damiani G, et al. Vincristine-resistant erythroleukemia cell line has marked increased sensitivity to hexamethylenebisacetamide-induced differentiation. Proc Natl Acad Sci U S A. 1988;85(11):3835–3839.
- Vaidhyanathan S, Mittapalli RK, Sarkaria JN, et al. Factors influencing the CNS distribution of a novel MEK-1/2 inhibitor: implications for combination therapy for melanoma brain metastases. Drug Metab Dispos. 2014;42(8):1292–1300.
- Einarsdottir BO, Karlsson J, Söderberg EMV, et al. A patient-derived xenograft pre-clinical trial reveals treatment responses and a resistance mechanism to karonudib in metastatic melanoma. Cell Death Dis. 2018;9(8):810..
- Zawistowski JS, Bevill SM, Goulet DR, et al. Enhancer remodeling during adaptive bypass to mek inhibition is attenuated by pharmacologic targeting of the P-TEFb complex. Cancer Discov. 2017;7(3):302–321..
- Daniel P, Filiz G, Brown DV, et al. Selective CREB-dependent cyclin expression mediated by the PI3K and MAPK pathways supports glioma cell proliferation. Oncogenesis. 2014;3:e108.
- Daniel PM, Filiz G, Brown DV, et al. PI3K activation in neural stem cells drives tumorigenesis which can be ameliorated by targeting the cAMP response element binding protein. Neuro Oncol. 2018;20(10):1344–1355.
- Tan X, Wang S, Yang B, et al. The CREB-miR-9 negative feedback minicircuitry coordinates the migration and proliferation of glioma cells. PLoS One. 2012;7(11):e49570.
- Tan X, Wang S, Zhu L, et al. cAMP response element-binding protein promotes gliomagenesis by modulating the expression of oncogenic microRNA-23a. Proc Natl Acad Sci U S A. 2012;109(39):15805–15810.
- Amorim S, Stathis A, Gleeson M, et al. Bromodomain inhibitor OTX015 in patients with lymphoma or multiple myeloma: a dose-escalation, open-label, pharmacokinetic, phase 1 study. Lancet Haematol. 2016;3(4):e196–204..
- Piha-Paul SA, Sachdev JC, Barve M, et al. First-in-human study of mivebresib (ABBV-075), an oral pan-inhibitor of bromodomain and extra terminal proteins, in patients with relapsed/refractory solid tumors. Clin Cancer Res. 2019;25(21):6309–6319..
- Postel-Vinay S, Herbschleb K, Massard C, et al. First-in-human phase I study of the bromodomain and extraterminal motif inhibitor BAY 1238097: emerging pharmacokinetic/pharmacodynamic relationship and early termination due to unexpected toxicity. Eur J Cancer. 2019;109:103–110.
- De Raedt T, Beert E, Pasmant E, et al. PRC2 loss amplifies Ras-driven transcription and confers sensitivity to BRD4-based therapies. Nature. 2014;514(7521):247–251..
- Jing Y, Zhang Z, Ma P, et al. MAPK blockade for effective treatment of ovarian cancer. Oncotarget. 2016;7(3):2545–2554.
- Ma Y, Wang L, Neitzel LR, et al. The MAPK pathway regulates intrinsic resistance to BET inhibitors in colorectal cancer. Clin Cancer Res. 2017;23(8):2027–2037.
- Echevarria-Vargas IM, Reyes-Uribe PI, Guterres AN, et al. Co-targeting BET and MEK as salvage therapy for MAPK and checkpoint inhibitor-resistant melanoma. EMBO Mol Med. 2018;10(5). DOI:10.15252/emmm.201708446.
- Wyce A, Matteo JJ, Foley SW, et al. MEK inhibitors overcome resistance to BET inhibition across a number of solid and hematologic cancers. Oncogenesis. 2018;7(4):35..
- Jespersen H, Lindberg MF, Donia M, et al. Clinical responses to adoptive T-cell transfer can be modeled in an autologous immune-humanized mouse model. Nat Commun. 2017;8(1):707.
- Ny L, Rizzo LY, Belgrano V, et al. Supporting clinical decision making in advanced melanoma by preclinical testing in personalized immune-humanized xenograft mouse models. Ann Oncol. 2020;31(2):266–273.