ABSTRACT
Systemic Sclerosis (SSc) is an autoimmune connective tissue disease that leads to skin and lung fibrosis. The Wnt pathway is clearly elevated in SSc and is pro-fibrotic via activation of canonical Wnt signalling. sFRP-1 is a Wnt antagonist that acts as a negative regulator of Wnt signalling. We sought to measure the levels of serum sFRP-1 in early diffuse SSc patients compared to healthy controls and if this is regulated by microRNA27a-3p. Ten early diffuse SSc patients and healthy controls sera were taken and sFRP-1 quantified by ELISA. Skin biopsies were also taken in five SSc patients and controls. Fibroblasts were quantified for microRNA27-3p expression by Taqman qRT-PCR with an internal microRNA to normalize. 3ʹUTR luciferase assays were performed to confirm direct targets of microRNA27a-3p with microRNA overexpression. Fibroblasts were transfected with microRNA27a mimics or scramble controls and using ELISA sFRP-1 was quantified. Furthermore, Collagen, Axin-2, TIMP-1 and MMP-1 were measured. Serum sFRP-1 was significantly reduced in early diffuse SSc patients. We identified microRNA27a-3p-3p as regulating sFRP-1 in dermal fibroblasts. We found significantly elevated microRNA27a-3p in isolated dermal fibroblasts from SSc patients. We confirmed that sFRP-1 is a direct target of microRNA27a-3p through cloning of the 3ʹUTR into a luciferase vector. ECM genes were also upregulated by microRNA27a-3p-3p and the matrix-degrading enzyme MMP-1 was suppressed. Serum sFRP-1 is reduced in diffuse SSc patients and is regulated by microRNA27a-3p and this is a direct regulation. Modulation of microRNA27a-3p levels could mediate fibrosis regression.
Introduction
Systemic sclerosis (SSc) is a rare, idiopathic, autoimmune connective tissue disease in which there is inflammation and resultant skin fibrosis [Citation1,Citation2]. Among all of the autoimmune rheumatic disorders this has the highest all-cause mortality and is currently lacking treatment that directly modifies the fibrotic element [Citation3]. The progressive fibrosis is due to the deposition of collagen type I and other extracellular matrix proteins. A critical feature of the disease is the activation of resident skin fibroblasts to myofibroblasts that are activated and secrete high levels of extracellular matrix molecules. These myofibroblasts are key to fibrosis and their activation, we have shown, is under the control of epigenetics [Citation4,Citation5].
We previously showed elevated methyl CpG-binding protein 2 (MeCP2), and this mediates repression of the Wingless-related integration site (Wnt) inhibitor secreted frizzled Related Protien-1 (sFRP1) in SSc [Citation6]. Other groups have demonstrated that sFRP-1 is anti-fibrotic and is repressed in dermal fibroblasts by epigenetic mechanisms leading to enhanced production of extracellular matrix (ECM) [Citation6,Citation7]. Methylation within the promoter region of sFRP-1 is associated with repression and enhanced Wnt-mediated fibrosis [Citation7] and stabilization of the Wnt effector β-catenin in dermal fibroblasts leads to skin fibrosis [Citation8]. However, whether other epigenetic mechanisms exist that regulate sFRP-1 levels are not clear. MicroRNAs are small non-coding RNAs that regulate gene expression and therefore regulate various signalling pathways [Citation9]. They regulate the mRNA of their target genes by binding to the 3ʹUntranslated Region (3ʹUTR) with the microRNAs ‘seed region’ and result in silencing of the gene through cleavage or destabilization of the mRNA leading to suppression. Thus, although microRNAs are small they can have a large effect on cell function and are implicated in a variety of rheumatic diseases [Citation10]. In the case of SSc, multiple microRNAs have been found to be dysregulated and have direct effects on both pro-fibrotic and anti-fibrotic pathways [Citation5,Citation11]. This study aims to determine the serum levels of sFRP-1 and if sFRP-1 is regulated epigenetically in early diffuse SSc.
Methods
Ten early diffuse SSc patients with disease duration less than 2 years (from first non-Raynaud’s phenomenon symptom) serum was drawn aseptically; all patients gave full-informed consent. They all fulfilled the 2013 ACR/EULAR criteria for SSc [Citation12] and all gave informed consent and this study was ethically approved by Research Ethics committee Sunderland (UK) under approval number REC/13/NE/0089 and followed the declaration of Helsinki guidelines. All 10 patients were treatment naïve and not on any form of immunosuppression and anti-Scl70 antibody positive (). All SSc patients were female mean age 56 years old (SD 5.2). Healthy controls were all female mean age 55 years old (SD 6) with full written informed consent. Serum was isolated and stored at −80°C until sFRP-1 was analysed.
Table 1. Patient demographics
ELISA
Isolated sera were defrosted upon use and we used a commercial ELISA (Raybiotech, USA) this detects human sFRP-1. We followed the manufacturers’ instructions and used recombinant sFRP-1 to generate a standard curve.
For Matrix Metalloprotease-1 (MMP-1) ELISA healthy dermal fibroblasts were seeded in 6-well plates and transfected with microRNA27a-3p (75 nM) or matched scramble control using HIPerFect (Qiagen) and after 48 h media was collected and MMP-1 was measured using manufactures’ instructions (R&D systems, UK).
Cell culture
Dermal biopsies were taken from early diffuse patients affected skin on the arm using a 5 mm punch biopsy and cultured as previously described [Citation13]. Isolated dermal fibroblasts cultured from healthy controls were also taken as previously described [Citation13]. All cultures were maintained in Dulbecco’s Modified Eagle’s Medium (DMEM) + 10% foetal calf serum (FCS) and penicillin (100 U/ml) and streptomycin (100 µg/ml). In some experiments cells were serum starved overnight in DMEM only and then incubated with human recombinant TGF-β1 (5 ng/ml) (R&D systems, UK) or vehicle control (HCL 0.01% v/v) and cells were harvested after 24 h and small RNA was isolated. MicroRNA 27a-3p was quantified using Taqman specific primers for Hsa-MiR-27a-3p (Applied Biosystems, UK) assay id: 478,384. RNU44 assay id: 001094 was also quantified and the data were normalized to this and presented as fold change. All samples were run in quadruple for each individual sample.
MicroRNA transfection
Healthy dermal fibroblasts were seeded into a twenty-four well plate and left to adhere overnight. Cells were then transfected with microRNA mimic microRNA27a-3p-3p (Applied Biosystems, MC10939) or scramble control at 75 nM for 48 h using HIPerFect transfection reagent (Qiagen, UK) according to manufacturer’s instructions. After 48 h the media was removed and sFRP-1 was quantified by ELISA following the manufacturer’s instructions. In some experiments cells were transfected with microRNA27a-3p-3p mimic (75 nM) or scramble control and after 4 h TGF-β1 (5 ng/ml) was added and after 48 h the cells were lysed and RNA harvested using TRIzol reagent (Invitrogen). 1 µg of RNA was reverse transcribed to cDNA using reverse transcriptase (Invitrogen) QPCR was performed for collagen1A1 with primers forward 5'-CAA GAG GAA GGC CAA GTC GAG G-3', reverse 5'- CGT TGT CGC AGA CGC AGA T-3', Axin‐2, forward 5'‐CGG GAG CCA CAC CCT TC‐3' and reverse 5'‐TGG ACA CCT GCC AGT TTC TTT‐3', TIMP-1: forward 5'-GAC GGC CTT CTG CA ATT CC-3', reverse 5'-GTA TAA GGT GGT CTG GTT GAC TTC TG-3' 18S: forward 5'-GAA TGG CTC ATT AAA TCA GTT ATG G-3', reverse 5'-TAT TAG CTC TAG AAT TAC CAC AGT TAT CC-3' PPARγ: forward 5'-GAA ATG ACC ATG GTT GAC-3' Reverse 5'-CCG CTA GTA CAA GTC CTT GTA-3' using SYBR green (Sigma, UK). Data were normalized to the housekeeping gene 18S and relative expression quantified using the Delta Delta CT method [Citation14].
Luciferase reporter assay for targeting the 3ʹUTR of sFRP1
The 3'UTR of sFRP-1 that was predicted to bind microRNA27a-3p-3p was identified using Targets can, was amplified up by PCR from human genomic DNA and inserted into the PmirGlo vector using Infusion cloning (Clontech) for the plasmid reporter generation using primers 3'_UTR_SFRP1_F (5'-3') GCTCGCTAGCCTCGAaatgtgaatcgcagctgtgg SFRP1_R(5'-3')CGACTCTAGACTCGAtga'acacgtacgggaattact. HEK293 cells were cultured overnight at 8 × 104 cells in DMEM + 10% FCS and then microRNA27a-3p-3p mimic (50 nM) was transfected using Effectene (Qiagen, UK) at 500 ng/ml reporter plasmid and 50 ng renilla (pRL-SV40 vector; Promega) for transfection efficacy was co-transfected simultaneously. Scramble matched control microRNA was added as controls. After 48 h of transfection, the cells were lysed and firefly and Renilla luciferase determined using the dual luciferase reporter (Promega, UK) using a microlumat plus luminometer, n = 4 independent cultures. Renilla luciferase was used to normalize for transfection efficacy and then the data once normalized was set to percentage change compared to plasmid plus scramble microRNA mimic.
TOPflash assay
TOPflash plasmids were purchased from Addgene and transfected into HEK293 cells at 500 ng/ml into a 12-well plate using FuGENE HD (Promega, UK). For normalization, they were also co-transfected simultaneously with pCMV Renilla luciferase at 50 ng/ml. After 4 h the transfection complexes were replaced with microRNA27a-3p mimic (75 nM) or matched scramble controls and all wells then received recombinant Wnt3a (100 ng/ml) in 0.5% FCS/DMEM for a further 24 h after which the cells were lysed and dual luciferase report (Promega, UK) was used to determine luminescence. Renilla luciferase was used to normalize transfection efficacy and activity was expressed as fold change n = 4 independent cultures.
5'aza-2'-deoxycytidine treatment
SSc fibroblasts were cultured in six-well plates and 1 µM of 5'aza-2-deoxycytidine (Sigma, UK) was added or vehicle control (acetic acid) for 72 h. After which time the cells were lysed and mir-27a-3p was quantified as above n = 4 independent cultures.
Sircol assay
SSc dermal fibroblasts were cultured and then transfected with AntimiR27a-3p (80 nM) or matched scramble control after 48 h in culture soluble collagen was measured in the cell supernatant by SirCol assay (Bicolour, Northern Ireland) as previously described [Citation15]. Data were normalized and set as 100% to scramble control transfection from four individual SSc donors.
Western blotting
SSc dermal fibroblasts were cultured in six well plates and transfected with AntimiR27a-3p (80 nM) or matched scramble control with HiPerfect reagent (Qiagen) (n = 3). After 48 h cells were harvested in RIPA buffer containing protease inhibitors and then total protein quantified using the Bradford assay. Twenty microgram total protein was loaded and subjected to polyacrylamide gel electrophoresis at 120 V. The protein was then transferred to a nitrocellulose membrane and blocked with 5% (w/v) blocking buffer and then probed with a specific collagen 1 antibody (Abcam; ab233639), washed and the incubated with secondary HRP linked antibody for 1 h before being exposed. Membranes were stripped and reprobed for β-actin (Abcam; ab8227, UK) as a loading control to confirm equal loading.
MTT assay
SSc dermal fibroblasts were cultured and transfected with Anti-Mir-27a-3p or scramble control (80 nM). MTT assay was performed using manufactures instructions (Sigma, UK). Triton-X was ran as a positive control.
Results
We sought to measure the levels of sFRP-1 in sera from early diffuse SSc patients. ) demonstrates that there are significantly reduced levels of sFRP-1 in early diffuse SSc patients compared to healthy controls 2.2 ng/ml HC v 0.71 ng/ml SSc (P = 0.001 Mann-Whitney U test n = 10).
Figure 1. Serum sFRP1 is reduced in SSc. (a) Serum levels of sFRP1 were measured by ELISA (RayBiotech) in diffuse SSc patients or healthy controls (n = 10). *Significantly different from HC; Mann-Whitney U test. 1B). Healthy dermal fibroblasts were cultured in vitro and transfected with 75 nM of microRNA27a-3p or matched scramble for 48 h after which the supernatant was collected and sFRP-1 measured by specific ELISA (*significant difference P = 0.028 Mann-Whitney U test n = 4). 1 C). SSc and healthy control fibroblasts were measured for microRNA27a-3p by Taqman PCR and normalized to RNU44 and data shown as fold change over healthy control (*significant difference P = 0.011; Mann-Whitney U test; n = 5) Data is the mean and SD
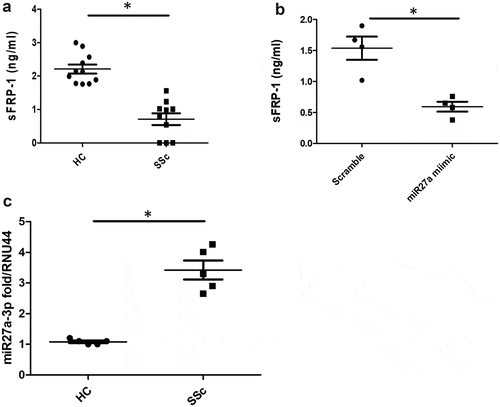
Because previous studies have shown that sFRP-1 is controlled by DNA methylation at its promoter region, we hypothesized that microRNAs could also be involved in regulating sFRP-1. Using Targetscan software we found that microRNA27a-3p could target sFRP-1 theoretically. Indeed, transfection of microRNA27a-3p into normal healthy dermal fibroblasts reduced the levels of sFRP-1 significantly compared to scrambled concentration-matched microRNA ()) (P = 0.028, Mann-Whitney U test, n = 4).
Incubation of normal healthy dermal fibroblasts with Transforming growth factor-β1 (TGF-β1) 5 ng/ml, used because this is a potent pro-fibrotic cytokine [Citation16], did not significantly alter the levels of microRNA27a-3p, indicating that this is not playing a role in modulating the miR-sFRP-1 axis (1.2 fold change TGF-β1 treated v vehicle treated control 1.1 fold; n = 5 P = >0.05 Mann-Whitney U test). We could confirm that the TGF-β1 was functional as the levels of collagen were increased significantly after incubation compared to vehicle treated cultures (three fold increased).
We next determined the levels of microRNA27a-3p in SSc dermal fibroblasts compared to healthy dermal fibroblasts and found significant elevation of microRNA27a-3p expression 3.4 fold compared to 1.1 fold for healthy controls (P = <0.011, Mann-Whitney U test, n = 5) ()).
To assess whether sFRP-1 is a direct target of microRNA27a-3p, as opposed to an indirect target, we cloned the 3ʹUTR of sFRP-1 that is predicted to bind, into the PmirGlo vector, downstream of firefly luciferase. We then transfected this vector into HEK293 cells with microRNA27a-3p or scrambled control and quantified luciferase activity. ) demonstrates significant repression of sFRP-1 luciferase with microRNA27a-3p transfection compared to scramble control at the matched concentration.
Figure 2. sFRP-1 is a direct target of microRNA27a-3p-3p and regulates Wnt pathway (a) Luciferase activity was detected after transfection of a luciferase vector containing the binding site for microRNA27a-3p-3p in the 3ʹUTR of sFRP-1 and microRNA27a-3p mimic (50 nM) or matched scramble controls in HEK293 cells. Data is relative luciferase normalized to Renilla luciferase and compared to scramble microRNA. Data is the mean and SD from 4 independent experiments *significantly different to control (P = 0.026 Mann-Whitney U test; n = 4). (b) sFRP-1 ‘UTR binding site is shown at the top and the microRNA27a-3p-3p seed region at the bottom, directly below is where this was cloned into the luciferase vector and its plasmid map. Bases in bold face are the key binding sites. (c) Collagen1 levels were quantified by qPCR after microRNA27a-3p transfection or scramble control in the presence of 5 ng/ml TGF-β1. Data is the mean and SD *significantly different compared to scramble (P = 0.029 Mann-Whitney U test; n = 5). (d) Axin-2 expression was quantified by qPCR after microRNA27a-3p transfection or scramble control. Data is the mean and SD* significantly different compared to scramble (P = 0.024 Mann-Whitney U test; n = 5). (e) TIMP-1 expression was quantified by qPCR after microRNA27a-3p transfection or scramble control. Data is the mean and SD. No significant difference was observed between groups (Mann-Whitney U test; n = 5)
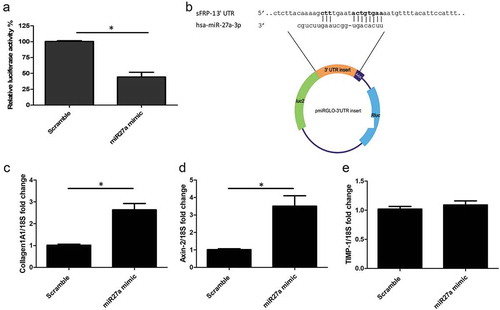
Compared to plasmid vector treated with scramble microRNA there was over 52% repression of luciferase 3ʹUTR of sFRP-1 (P = 0.026; Mann-Whitney U test n = 4), confirming this is a true direct target. ) shows the microRNA27a-3p binding site in sFRP-1 and the vector plasmid map used in this study.
Transfection of microRNA27a-3p mimic with TGF-β1 in healthy dermal fibroblasts led to significantly increased expression of collagen1A1 and the Wnt target gene Axin-2 () significantly different compared to scramble control (P = 0.029 Collagen1A1) Mann-Whitney U test n = 5) but no significant change was apparent in TIMP-1 gene expression compared to scramble control microRNA ()), however MMP-1 protein, the primary protease for collagen, was significantly reduced by microRNA27a-3p compared to scramble control (P = 0.009 students t test n = 5) ()) and the antifibrotic nuclear receptor PPARγ was also significantly reduced compared to scramble control at the mRNA level (P = 0.006 Students t test n = 4) ()) To confirm that the Wnt pathway was activated in these cells with microRNA27a-3p expression we used TOPflash reporter assay which is a reporter system based on TCF activation (Wnt). Transfection of the reporter into HEK293 cells and transfection of microRNA27a-3p-3p increased reporter activity by 2.5 fold compared to scramble control microRNA at matched concentration, ) (P = <0.0001 Students t test; n = 4).
Figure 3. MMP-1 is reduced after miR-27a-3p. (a) MMP-1 was quantified by ELISA after transfection of dermal fibroblasts with microRNA27a-3p or scramble control. Data is the mean and SD *significantly different to control (P = 0.009 Students t test, n = 5) (b) PPARγ is reduced after miR27a-3p is increased. PPARγ was quantified after miR27a-3p transfection or scramble control. Data is the mean and SD. Gene expression is normalized to 18S as the housekeeping gene and shown as fold change. *significantly different compared to scramble (Students t test P = 0.006 n = 4) (c) HEK293 cells were transfected with TOPflash and then incubated with scramble mimics or microRNA27a-3p mimics plus Wnt3a (100 ng/ml). After 24 h Wnt activity was measured by luciferase activity and normalized to Renilla to normalize for transfection efficacy. Data is the mean and SD * = significant difference compared to scramble (P = <0.001 students t test; n = 4)
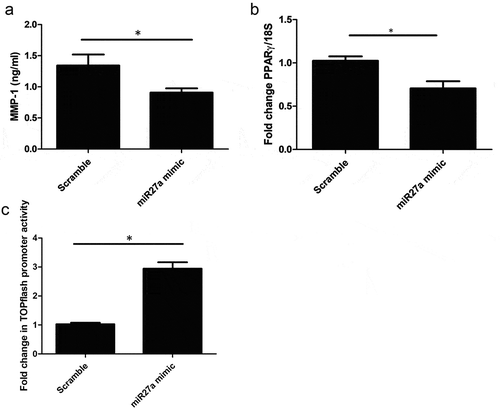
Because methylation has been found to be important in fibrosis and the regulation of sFRP-1 we wondered if incubating with the pan demethylase 5'aza’C would lead to repression of miR-27-a-3p. It was found that incubation of SSc dermal fibroblasts with 5'aza’C had no significant effect on miR expression levels (Vehicle 1.025 SD; 0.043 vs 5'aza’C 1.011 SD; 0.034 P = 0.7 Mann-Whitney U test n = 4).
Finally using SSc dermal fibroblasts with known reduced sFRP-1 levels we transfected mir27a-3p antagonists, that would block interaction with sFRP-1 mRNA, and measured collagen release. ) demonstrates that anti-miR-27a-3p reduced collagen release by 33% compared to scramble control (100% SD; 0.5 v 67 SD; 4.2% P = <0.0001 Students t test n = 4). We also saw reduction in collagen 1 levels by western blotting after miR27a-3p inhibition ()). To confirm this was not simply due to cell death induced by the miR we performed an MTT assay for cell viability that demonstrated no significant difference in cell viability across the conditions ()).
Figure 4. Anti-miR-27a-3p treatment reduced collagen in SSc fibroblasts. (a) SSc dermal fibroblasts were cultured and transfected with antagomiR27a-3p or scramble (80 nM) controls and after 48 h the media was removed and collagen quantified by Sircol assay. Data is the percentage of scramble control after 48 h transfection and is the mean and SD *Significant difference versus scramble control, (P = <0.0001; students t test n = 4). (b) Representative western blot of collagen1 after scramble control microRNA or antagomiR27a-3p after 48 h. β-actin is the loading control. Scr = Scramble, Anti-miR = AntagomiR27a-3p. (c) SSc dermal fibroblasts were transfected with antagomiR27a-3p or scramble controls and after 48 h MTT assay was performed. No significant difference between groups was observed P = >0.05; students t test, n = 4)
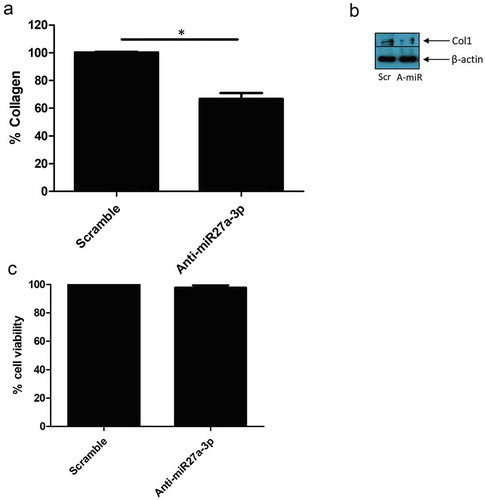
Discussion
Wnt proteins are lipid modified glycoproteins that have critical roles in development and tissue homoeostasis [Citation17]. It is now appreciated that the Wnt pathway is critical in fibrosis and SSc [Citation18–21]. The Wnt inhibitor sFRP-1 blocks Wnt signalling extracellularly and we recently demonstrated that genetic reduction of sFRP-1 increased collagen production in isolated skin fibroblasts [Citation6] and mice with a genetic deletion of sFRP-1 have enhanced kidney fibrosis [Citation22].
We sought to determine the serum levels of sFRP-1 in early diffuse SSc and found that they are significantly reduced, confirming isolated in vitro studies [Citation7]. Furthermore, we found that microRNA27a-3p-3p regulated sFRP-1 levels as overexpression reduced the Wnt inhibitors expression. We hypothesized that TGF-β1 would regulate the levels of the microRNA however, this was not found. In SSc dermal fibroblasts, we also found elevated microRNA27a-3p levels compared to healthy controls, suggesting that microRNA27a-3p reduced sFRP-1 levels epigenetically and leads to enhanced Wnt signalling leading to fibrosis (). This adds to previous work demonstrating methylation of sFRP-1 leads to its repression in dermal fibroblasts [Citation7]. Importantly, we demonstrate that SSc dermal fibroblasts when microRNA27a-3p was inhibited reduced collagen release, suggesting that epigenetic restoration of Wnt antagonist may be a therapeutic option.
Figure 5. Putative pathway in SSc. In SSc there are lower levels of sFRP-1 as opposed to the healthy situation, mediated by microRNA27a-3p repression. This leads to attenuated inhibition of Wnt via the Frz receptor that ultimately leads to activation of the myofibroblast and fibrosis. Dark lines indicate more inhibition. Frz; Frizzled receptor, Wnt; Wingless-related integration site, SSc: systemic sclerosis
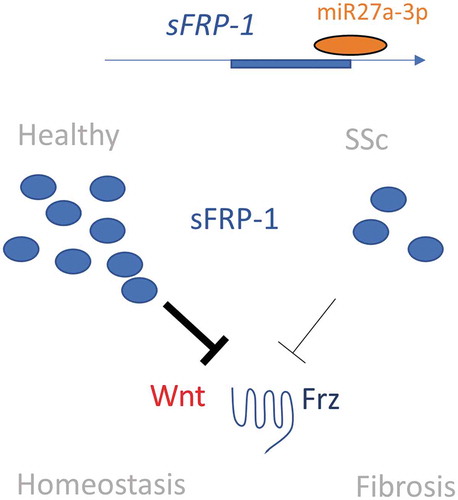
MicroRNAs are small RNAs that regulate gene expression at the post-transcriptional level [Citation23]. The mechanism through which miRs mediate their effects is through binding of the seed region of the miR and specific bases within the 3ʹUTR of the mRNA that leads to repression [Citation9]. We confirmed that microRNA27a-3p can target sFRP-1 directly by cloning the 3ʹUTR that contains the binding site into a luciferase vector and transfecting with microRNA27a-3p or scramble control and could see a significant reduction in luciferase activity. Therefore, confirming that this is a bona fide target of the microRNA. We found also that microRNA27a-3p-3p overexpression led to upregulation of collagen1A1 and the known Wnt target gene Axin-2 suggesting enhancement of the Wnt pathway. Although no change was seen in the expression of TIMP-1, suggesting Wnt does not regulate this. However, MMP-1 was significantly reduced after overexpression of microRNA27a-3p. An intricate balance exists between the breakdown (MMPs) and the genesis of matrix. TIMPs are the primary proteins that inhibit MMPs and thus a shift in the ratio of TIMP:MMP will favour the deposition of ECM. It is not predicted that MMP-1 is a direct target of microRNA27a-3p thus it is likely that the effect is either indirect and a consequence of Wnt signalling. Examination of PPARγ mRNA after miR27a-3p transfection demonstrated reduction of this specific nuclear receptor. PPARγ is an antifibrotic molecule that is reduced in fibrotic diseases including SSc [Citation24]. Indeed agonists that stimulate PPARγ are anti-fibrotic [Citation25]. It is unknown if miR27a-3p directly or indirectly targets PPARγ in these cells. We could confirm also direct activation of the Wnt signalling pathway using the TOPflash assay which is widely used assay which uses three copies of the TCF binding sites.
MicroRNA27a-3p has been demonstrated to be upregulated in experimental diabetic kidney fibrosis mediated by high glucose [Citation26] and is often upregulated in carcinomas [Citation27]. Indeed, it was shown that antagomiR that inhibits microRNA27a-3p in vivo led to both reduced microRNA27a-3p expression and reduced fibrosis associated with less collagen and fibronectin levels in diabetic nephropathy mice [Citation26]. Hepatic stellate cells are the cells in the liver responsible for the production of ECM leading to liver fibrosis when activated. These cells are akin to activated skin myofibroblasts in SSc. It was shown that microRNA27a-3p-3p is upregulated in these cells by leptin and promotes the expression of both collagen and alpha-smooth muscle actin via down regulation of Liver X receptor-α [Citation28]. Indeed, liver X receptor-α activation by a specific agonist reduced the bleomycin-induced fibrosis in the bleomycin animal model [Citation29]. It is possible that this receptor is also targeted in SSc by microRNA27a-3p as microRNAs have multiple targets, although we did not explore this within this study. Blockade of TGF-β signalling in hepatic stellate cells reduced miR27a levels [Citation30], but in our hands TGF-β1 stimulation did not alter the microRNAs expression. We also demonstrated that pan demethylation did not alter the expression of microRNA27a-3p, indicating that this is not a methylation-sensitive miR.
What regulates microRNA27a-3p and leads to its reduction in SSc is not known, although we found no reduction with TGF-β1, a known potent pro-fibrotic molecule [Citation2]. It is possible other cytokines and chemokines are regulating this microRNA, which then leads to enhanced fibrosis. Indeed, epithelial cells can produce cytokines in SSc that result in myofibroblast activation possibly mediated through microRNAs [Citation31]. Importantly we showed that modulation of microRNA27a-3p levels through inhibition reduced collagen in diseased SSc cells, both secreted and endogenous, and that this was not associated with cell toxicity. Given the importance of Wnt signalling in SSc the identification of epigenetic regulators of this pathway is of primary importance.
Conclusions
To our knowledge this is the first study to identify reduced serum levels of sFRP-1 in early diffuse SSc, and that this is regulated by microRNA27a-3p. We identify microRNA27a-3p as an anti-fibrotic microRNA and targeting this may be useful as a therapeutic in diffuse SSc. Further replication studies should be undertaken to determine sFRP-1s utility as a possible biomarker.
Declarations
Ethics approval and consent to participate: All patients gave informed consent and this study was ethically approved by the Research Ethics committee Sunderland (UK) under approval number REC/13/NE/0089 and followed the declaration of Helsinki guidelines.
Consent for publication
The datasets used and/or analysed during the current study are available from the corresponding author on reasonable request.
Disclosure statement
No potential conflict of interest was reported by the authors.
References
- Henderson J, Bhattacharyya S, Varga J, et al. Targeting TLRs and the inflammasome in systemic sclerosis. Pharmacol Ther. 2018;192:163–169.
- Allanore Y, Simms R, Distler O, et al. Systemic sclerosis. Nat Rev Dis Primers. 2015;1(1):15002.
- Denton CP, Khanna D. Systemic sclerosis. Lancet. 2017;390(10103):1685–1699.
- O’Reilly S. Epigenetics in fibrosis. Mol Aspects Med. 2017;54:89–102.
- Henderson J, Distler J, O’Reilly S. The role of epigenetic modifications in systemic sclerosis: a druggable target. Trends Mol Med. 2019;25(5):395–411.
- Henderson J, Brown M , Horsburgh S, et al. Methyl cap binding protein 2: a key epigenetic protein in systemic sclerosis. Rheumatology. 2018;key327–key327.
- Dees C, Schlottmann I, Funke R, et al. The Wnt antagonists DKK1 and SFRP1 are downregulated by promoter hypermethylation in systemic sclerosis. Ann Rheum Dis. 2014;73(6):1232–1239.
- Beyer C, Schramm A, Akhmetshina A, et al. β-catenin is a central mediator of pro-fibrotic Wnt signaling in systemic sclerosis. Ann Rheum Dis. 2012;71(5):761–767.
- Bartel DP. MicroRNAs: genomics, biogenesis, mechanism, and function. Cell. 2004;116(2):281–297.
- Frank-Bertoncelj M, Klein K, Gay S. Interplay between genetic and epigenetic mechanisms in rheumatoid arthritis. Epigenomics. 2017;9(4):493–504.
- O’Reilly S. MicroRNAs in fibrosis: opportunities and challenges. Arthritis Res Ther. 2016;18(1):11.
- van den Hoogen F, Khanna D, Fransen J, et al. 2013 classification criteria for systemic sclerosis: an American college of rheumatology/European league against rheumatism collaborative initiative. Ann Rheum Dis. 2013;72(11):1747–1755.
- O’Reilly S, Ciechomska M, Cant R, et al. Interleukin-6 (IL-6) trans signaling drives a STAT3-dependent pathway that leads to hyperactive transforming growth factor-β (TGF-β) signaling promoting SMAD3 activation and fibrosis via gremlin protein. J Biol Chem. 2014;289(14):9952–9960.
- Livak KJ, Schmittgen TD. Analysis of relative gene expression data using real-time quantitative PCR and the 2−ΔΔCT method. Methods. 2001;25(4):402–408.
- Huber LC, Distler JHW, Moritz F, et al. Trichostatin A prevents the accumulation of extracellular matrix in a mouse model of bleomycin-induced skin fibrosis. Arthritis Rheumatism. 2007;56(8):2755–2764.
- Lafyatis R. Transforming growth factor β–at the centre of systemic sclerosis. Nat Rev Rheumatol. 2014;10(12):706–719.
- Wiese KE, Nusse R, van Amerongen R. Wnt signalling: conquering complexity. Development. 2018;145(12):dev165902.
- Beyer C, Reichert H, Akan H, et al. Blockade of canonical Wnt signalling ameliorates experimental dermal fibrosis. Ann Rheum Dis. 2013;72(7):1255–1258.
- Akhmetshina A, Palumbo K, Dees C, et al. Activation of canonical Wnt signalling is required for TGF-β-mediated fibrosis. Nat Commun. 2012;3(1):735.
- Kim K-I, Jeong D-S, Yoon T-J, et al. Inhibition of collagen production by ICG-001, a small molecule inhibitor for Wnt/β-catenin signaling, in skin fibroblasts. J Dermatol Sci. 2017;86(1):76–78.
- Teratani T, Tomita K, Suzuki T, et al. Aortic carboxypeptidase–like protein, a WNT ligand, exacerbates nonalcoholic steatohepatitis. J Clin Invest. 2018;128(4):1581–1596.
- Matsuyama M, Nomori A, Nakakuni K, et al. Secreted frizzled-related protein 1 (Sfrp1) regulates the progression of renal fibrosis in a mouse model of obstructive nephropathy. J Biol Chem. 2014;289(45):31526–31533.
- Horsburgh S, Fullard N, Roger M, et al. MicroRNAs in the skin: role in development, homoeostasis and regeneration. Clin Sci. 2017;131(15):1923–1940.
- Wei J, Bhattacharyya S, Varga J. Peroxisome proliferator-activated receptor γ: innate protection from excessive fibrogenesis and potential therapeutic target in systemic sclerosis. Curr Opin Rheumatol. 2010;22(6):671–676.
- Wei J, Ghosh AK, Sargent JL, et al. PPARγ downregulation by TGFß in fibroblast and impaired expression and function in systemic sclerosis: a novel mechanism for progressive fibrogenesis. Plos One. 2010;5(11):e13778.
- Wu L, Wang Q, Guo F, et al. MicroRNA-27a induces mesangial cell injury by targeting of PPARγ, and its in vivo knockdown prevents progression of diabetic nephropathy. Sci Rep. 2016;6:26072.
- Zhou L, Liang X, Zhang L, et al. MiR-27a-3p functions as an oncogene in gastric cancer by targeting BTG2. Oncotarget. 2016;7(32).
- Li Z, Ji L, Su S, et al. Leptin up-regulates microRNA-27a/b-3p level in hepatic stellate cells. Exp Cell Res. 2018;366(1):63–70.
- Beyer C, Huang J, Beer J, et al. Activation of liver X receptors inhibits experimental fibrosis by interfering with interleukin-6 release from macrophages. Ann Rheum Dis. 2015;74(6):1317–1324.
- Davoodian P, Ravanshad M, Hosseini SY, et al. Effect of TGF-β/smad signaling pathway blocking on expression profiles of miR-335, miR-150, miR-194, miR-27a, and miR-199a of hepatic stellate cells (HSCs). Gastroenterol Hepatol Bed Bench. 2017;10(2):112–117.
- Aden N, Nuttall A, Shiwen X, et al. Epithelial cells promote fibroblast activation via IL-1α in systemic sclerosis. J Invest Dermatol. 2010;130(9):2191–2200.