ABSTRACT
Preimplantation development is critical for reproductive successes in mammals. Thus, it is important to understand how preimplantation embryogenesis is regulated. As a key event of preimplantation development, epigenetic reprogramming has been widely studied, yet how epigenetic complexes regulate preimplantation development remains largely unknown. Retinoblastoma binding protein 4 (RBBP4) and 7 (RBBP7) are integral components of epigenetic complexes including SIN3A, NuRD, and CoREST. Here, we demonstrate that double knockdown of Rbbp4 and 7, but not individually, causes embryonic lethality during the morula-to-blastocyst transition. Mechanistically, depletion of RBBP4 and 7 results in dysregulation of genes related to cell cycle, lineage development, and regulation of transcription, which is accompanied by cell cycle block, disrupted lineage specification and chromatin structure. Interestingly, RBBP4/7 depletion leads to a dramatic increase in H3.3 and H3K27ac abundance during morula-to-blastocyst transition. ChIP-seq analysis in early embryos and embryonic stem cells reveals enrichment of H3.3 at the promoter regions of RBBP4/7 target genes. In summary, our studies demonstrate the compensatory role of RBBP4/7 and reveal its potential mechanisms in preimplantation development.
Summary sentence:
RBBP4 and RBBP7 play a compensatory role in regulating cell proliferation, apoptosis, and histone H3.3 deposition during preimplantation development.
Introduction
Preimplantation development marks the beginning of a new life’s development and is critical for reproductive successes in mammals. Indeed, defects in preimplantation development represent one of the major reasons leading to infertility/subfertility or developmental complications in various species, including human [Citation1] and cows [Citation2,Citation3]. Morphologically, the fertilized 1-cell embryo undergoes three sequential cleavage divisions and become compacted and polarized at 8-cell stage. These morphological changes take place along with maternal zygotic transition (MZT), during which maternal factors are gradually degraded and zygotic genome activated (ZGA). The following cleavages give rise to the inside and outside portions of the embryos when the first lineage specification programis initiated. The polarized outside cells form the trophectoderm (TE), while the apolar inside cells are known as the inner cell mass (ICM) [Citation4]. ICM will further develop into epiblast (EPI) and primitive endoderm (PE). Establishment of these three lineages by implantation is required for successful pregnancy. Although the preimplantation embryogenesis has been extensively studied, yet the regulatory mechanisms remain largely unknown.
A remarkable reprogramming of the epigenome occurs during preimplantation development [Citation5]. The epigenetic information mainly includes DNA methylation, histone modification, and chromatin remodelling. In differentiated somatic cells, such information is stable, but the epigenetic information changes dynamically during gametogenesis and early embryonic development. The epigenetic reprogramming is precisely regulated and plays an important role in the establishment of totipotency. The dysregulation of epigenetic modifying enzymes can lead to abnormal gametogenesis or early embryonic developmental arrest [Citation6,Citation7]. Aberrant epigenetic reprogramming has been closely linked with the developmental failure in mouse embryos [Citation8]. Meanwhile, abnormal epigenetic information is also an important barrier to the development of somatic cell nuclear transfer embryos and the main cause of low cloning efficiency [Citation9]. However, the mechanisms underlying epigenetic reprogramming during preimplantation development remains unclear.
Retinoblastoma binding protein 4 (RBBP4) and 7 (RBBP7) (also known as Retinoblastoma associated protein 48 and 46, RBAP48 and RBAP46) are two highly homologous nuclear proteins that play key roles in establishing and maintaining chromatin structure [Citation10–12]. They are commonly seen in several critical transcriptional corepressors involved in histone deacetylation and chromatin assembly, including SIN3A, NuRD, and CoREST [Citation13]. RBBP4/7 physically interact with histone tails (H3 and H4) and are promising candidates for recruiting the epigenetic complexes. Genetic deletion of RBBP4 does not affect blastocyst formation but results in severe apoptosis, abnormal ICM specification, and hyperacetylated histones during mouse preimplantation development [Citation14]. We previously found that deficiency of both RBBP4 and 7 results in a developmental arrest during morula-to-blastocyst transition [Citation6], which is worse than the phenotype of Rbbp4 knockout embryos. This discrepancy suggests a compensatory role of RBBP4 and 7 in preimplantation development. However, the specific roles and the underlying mechanisms are yet to be defined.
Herein, we show that co-knockdown of Rbbp4 and 7 (coKD) resulted in embryonic lethality during the morula-to-blastocyst transition. The developmental failure is accompanied by a substantial perturbation of the transcriptomes and lineage development as well as increased incidence of apoptosis, enhanced histone acetylation and delayed cell cycle. Interestingly, RBBP4/7 depletion leads to a significant increase in H3.3 and H3K27ac abundance during morula-to-blastocyst transition. Overall, our data show that RBBP4 and 7 play compensatory and essential roles during preimplantation development.
Results
Co-depletion of RBBP4 and 7 results in a developmental failure during morula-to-blastocyst transition
We previously found individual knockdown of Rbbp4 (R4KD) or Rbbp7 (R7KD) does not affect preimplantation development while co-knockdown of Rbbp4 and Rbbp7 significantly influences the blastocyst formation rate by 50% via RNAi strategy [Citation6](Fig. S1A). To further determine the developmental potential of the remaining blastocysts in coKD groups, blastocysts were cultured individually to examine if outgrowths could be formed. Results showed the potential to form outgrowth was greatly compromised in coKD groups (Fig. S1B and S1C). Thus, these results collectively suggest RBBP4 and 7 play a redundant and essential role in preimplantation development.
Functional redundancy of RBBP4 and 7 in regulating gene expression
To examine how RBBP4 and 7 regulate preimplantation development in a molecular perspective, we performed RNA-sequencing (RNA-seq) on negative control (NC), R4KD, R7KD, and coKD morula ()). Both replicates for RNA-seq samples from each group exhibited high correlation (Fig. S2A-S2D). Meanwhile, Rbbp4 and 7 were significantly ablated in R4KD and R7KD groups, respectively, while both genes were dramatically reduced in coKD groups, further confirming a robust knockdown efficiency for both genes (Table S3-S5).
Figure 1. Functional redundancy of RBBP4 and 7 in regulating gene expression. A. Experimental scheme for RNA-seq of morula in NC (non-specific siRNAs), R4KD (Rbbp4 siRNAs), R7KD (Rbbp7 siRNAs) and coKD (Rbbp4 and Rbbp7 siRNAs) groups. B, C and D. Heatmaps showing differentially expressed genes at morula between NC and R4KD (panel B), R7KD (panel C), coKD (panel D) embryos. E. Gene ontology analysis of downregulated genes identified in coKD embryos relative to NC groups. F and G. Venn diagrams showing the common differentially expressed genes at morula stage identified among R4KD, R7KD and coKD embryos (panel F) or Hdac1/2 coKD, Sin3a KD and coKD embryos (panel G) relative to NC groups.
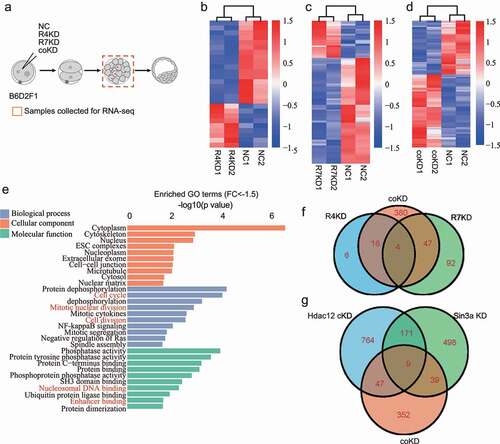
Results showed 26 genes were differentially expressed (DEGs, P-adj≤0.05; Fold change≥1.5 or ≤ −1.5) with 17 downregulated and 9 upregulated genes in R4KD embryos () and Table S3) while there are 143 DEGs, of which 106 downregulated and 37 upregulated genes in R7KD embryos () and Table S4). In contrast, 447 genes were differentially expressed, of which 257 were upregulated and 190 were downregulated in coKD embryos () and Table S5). These comparative analyses suggest individual knockdown of Rbbp4/7 has minor influences on the global gene expression and Rbbp4/7 plays a compensatory role in regulating gene expression during preimplantation development.
To explore the mechanisms how RBBP4 and 7 regulate preimplantation development, Gene ontology (GO) analysis was performed. Analysis of upregulated genes indicated that the overrepresented genes involved in negative regulation of cell growth, and positive regulation of apoptotic process (Fig. S2E). The most enriched GO terms of downregulated genes included cell cycle, mitotic nuclear division, and nucleosome DNA binding ()).
Because RBBP4/7 are present together with HDAC1/2 in multiple transcriptional repressive complexes, such as NuRD, CoREST, and SIN3A. To address if there is a link regarding the functional role of RBBR4/7 and HDAC1/2 in preimplantation embryos, we compared DEGs caused by Rbbp4/7 coKD and Hdac1/2 coKD. Results revealed that the number of DEGs caused by Rbbp4/7 coKD is significantly less than the one in Hdac1/2 coKD and Sin3a KD morula (). Meanwhile, only a small portion of the dysregulated genes was also affected by Hdac1/2 coKD or Sin3a KD (). This result indicates that HDAC1/2 and SIN3A play a more broad role in regulating gene expression in mouse preimplantation development.
RBBP4 and 7 reduction blocks cell cycle progression in preimplantation embryos
We previously showed that there is a reduction in total cell number per blastocyst in coKD groups [Citation6], which could be attributed to the defective cell cycle progression and/or increased apoptosis. In accordance, our present RNA-seq data revealed abnormal expression of genes involved in cell cycle progression, including E2f2, Cdca3, Gadd45a, and Gadd45b ()).
Figure 2. RBBP4 and 7 reduction blocks cell cycle progression in preimplantation embryos. A. RBBP4 and 7 reduction leads to dysregulation of cell cycle related genes, including E2f2, Cdca3, Gadd45a and Gadd45b. B and C. Immunostaining against EdU to assess DNA synthesis in NC and coKD blastocysts. Scale bar = 50 μM. D-G. Immunostaining against phosphorylation of S10 on histone H3 (pH3S10), an established marker for G2/M phase, in NC and coKD morula (d and e) and blastocysts (f and g). Scale bar = 50 μM. H-K. Immunostaining analysis of phosphorylation of Rb (pRb), a marker for G1/S checkpoint, in NC and coKD morula (h and i) and blastocysts (j and k). Scale bar = 50 μM.
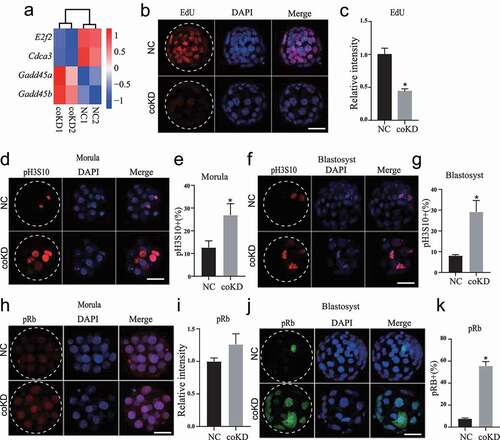
To assess the cell proliferation status of the coKD embryos, we analysed DNA synthesis with 5-Ethynyl-2′-deoxyuridine (EdU) incorporation and mitosis with histone H3 serine 10 phosphorylation staining (pH3S10), a well-established hallmark for G2/M phase. IF analysis revealed EdU signal is diminished in coKD embryos relative to controls (), suggesting a cell cycle block at S phase. Results showed the percentage of pH3S10 positive blastomeres is significantly increased in coKD embryos at both morulae and blastocyst stage (), suggesting a cell cycle block at G2/M phase. Moreover, percent pH3S10 positive blastomeres is also significantly increased in R4KD embryos but not in R7KD embryos (Fig. S3A), which is consistent with the phenotype seen in Rbbp4 knockout blastocysts [Citation14].
RBBP4 and 7 are known for binding directly to retinoblastoma protein (Rb) [Citation15], whose phosphorylation is involved in progression through G1/S checkpoint [Citation16]. To establish if RBBP4/7 depletion affected Rb phosphorylation, we performed IF against pRb and found pRb was not obviously altered at morula stage; however, percent pRb was greatly increased at blastocyst stage (). Collectively, these results demonstrate RBBP4/7 does not affect progression through G1/S checkpoint but is required for G2/M progression in preimplantation embryos.
RBBP4 and 7 reduction induces genome damage and increases apoptosis in preimplantation embryos
We next performed TUNEL assay to assess the apoptotic status of cells in preimplantation embryos. Results show the incidence of apoptosis was markedly increased in coKD blastocysts but not in R4 and R7 KD embryos (,b) and Fig. S4A).
Figure 3. RBBP4 and 7 reduction induces genome damage and increases apoptosis in preimplantation embryos. A and B. Cell apoptosis analysis of NC and coKD blastocysts. Three replicates of 5–10 blastocysts per group were analysed. C and D. Representative pictures of immunostaining against Histone H2AX phosphorylation (rH2AX), a marker for DNA damage, in NC and coKD blastocysts. Asterisks refer to significant differences (P < 0.05). E. Immunostaining of TRP53 acetylation (p53) in NC and coKD blastocysts.
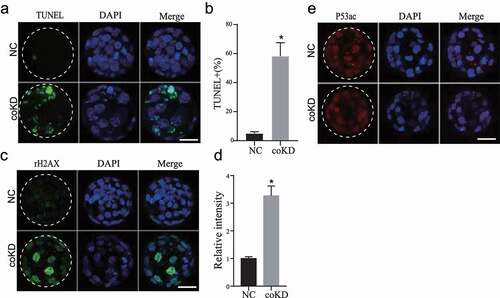
The cell cycle arrest and the apoptosis could be attributed to increased DNA damages. We next analysed DNA damage and repair by assessing the level of γH2AX, a well-known marker for DNA damage. IF results displayed unchanged γH2AX signal in both R4KD and R7KD embryos (Fig. S4B). In contrast, γH2AX signal was increased dramatically in coKD embryos (,d)). Acetylation of p53 is a critical upstream molecule inducing DNA damage. We then evaluated the level of TRP53 acetylation (p53ac) and found that it was unchanged in coKD ()). In summary, these results indicate RBBP4/7 are essential for genome integrity and embryo viability.
Recently, it has been shown that intercellular microtubule bridges between blastomeres are unique structures with critical roles in preimplantation embryos [Citation17]. Surprisingly, we found the ratio of intercellular bridges was drastically decreased in coKD embryos (Fig. S4C and S4D), suggesting defective cell–cell communications.
Double knockdown of Rbbp4 and 7 results in failed lineage specification of trophectoderm and inner cell mass
The earliest lineage specification takes place during the morula-to-blastocyst transition and generates TE (future placental cells) and ICM (future embryo proper), we thus decided to examine the cell differentiation program in coKD embryos. Transcriptome profiling revealed substantial enrichment of lineage-specific genes among DEGs in coKD embryos ()). Previously, we documented Rbbp4/7 coKD but not individual KD impair TE specification. Here, we further quantified the expression of OCT4, a critical molecular marker of ICM. IF results show the OCT4 is not restricted into the ICM in coKD embryos as in control embryos ()). Collectively, these data demonstrate a failure of the first cell fate decision that normally gives rise to TE and ICM cells.
Figure 4. Double knockdown of RBBP4 and 7 results in failed lineage specification of trophectoderm and inner cell mass. A. RNA-seq analysis reveals dysregulation of genes related to lineage development. B. Immunostaining analysis of OCT4, a marker of trophectoderm, and GATA6, a marker for primitive endoderm, in NC and coKD blastocysts at E4.25 (Three replicates of 3–5 embryos per group). OCT4 is restricted into ICM regions (dashed circle) in NC blastocysts, but was still observed in trophectoderm cells (arrows) in coKD blastocysts. C and D. Immunostaining analysis of SOX17, a marker for primitive endoderm, in NC and coKD blastocysts at E4.25 (Three replicates of 3–5 embryos per group). E and F. Immunostaining analysis of YAP in NC and coKD blastocysts at E4.25 (monolayer)Three replicates of 3–5 embryos per group.
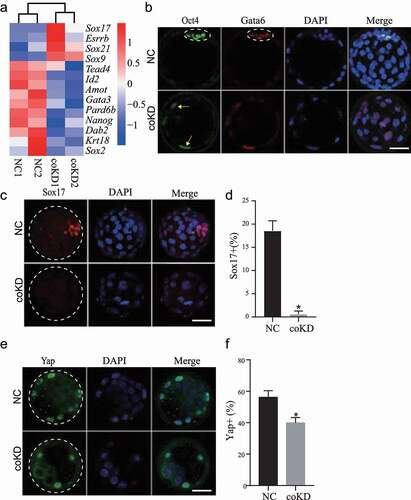
The second lineage specification occurs in late blastocysts when the ICM differentiates into EPI and PE. We examined GATA6 and SOX17, markers of PE, to determine if the second lineage specification failed as well. Results showed GATA6 was not corrected patterned inside the ICM while SOX17 was drastically abolished ()). Thus, these results demonstrate that RBBP4/7 are required for the first and second lineage specification in mouse preimplantation embryos. However, given that the developmental progression is blocked in coKD blastocysts, we cannot rule out the possibility that the failed lineage specification is an indirect effect of RBBP4/7 deficiency.
Hippo pathway is well known to be involved in regulating lineage development during mouse preimplantation development. The unphosphorylated YAP1 localizes to the nuclei and promotes CDX2 expression with TEAD4 in TE cells while phosphorylated YAP translocates into the cytoplasm [Citation4]. RNA-seq revealed no difference in the expression of Tead4 and Yap1 between NC and KD embryos. However, IF analysis showed that the number of YAP1-positive blastomeres was reduced significantly in coKD group () and 4(f)), but TEAD4 is unchanged (data not shown). The data suggest the defective TE formation in coKD embryos could be at least in part attributed to the abnormal Hippo signalling pathway.
Depletion of both RBBP4 and 7 decreases HDAC1 and increases H4K16ac
RBBP4 and 7 are integral subunits of multiple transcriptional repressive complexes that containing HDAC1/2, including SIN3A, NuRD, and CoREST [Citation18,Citation19]. We then examined the level of major components of these complexes in coKD embryos. RNA-seq analysis shows insignificant changes in mRNA abundance for these components ()). Interestingly, we found that the signal of HDAC1 is diminished at blastocyst stage while HDAC2 is normal in coKD embryos ()), suggesting RBBP4/7 is critical to recruit HDAC1 or maintain its stability. It is also possible such HDAC1 decrease could be due to the developmental delay seen in coKD blastocysts. Results show that the level of H4K16ac is increased significantly in coKD at blastocyst stage but not morula stage ( and S6A). Such increase in H4K16ac could be attributed to the decrease in HDAC1 level.
Figure 5. Depletion of both RBBP4 and 7 decreases Hdac1 and increases H4K16ac. A. mRNA abundance of major components in RBBP4/7-containing transcriptional complexes in NC and coKD morulas. B. Representative images of immunostaining against HDAC1. C. HDAC1 signal intensity is diminished in coKD blastocysts. Three replicates of 3–5 embryos per group. D. HDAC2 level is not changed obviously in coKD blastocysts. E. Representative images of immunostaining against H4K16ac. F. H4K16ac signal intensity is increased in coKD blastocysts. Three replicates of 3–5 embryos per group. G and H. Ectopic injection of Hdac1 mRNA could rescue SOX17. Asterisks refer to significant differences (P < 0.05).
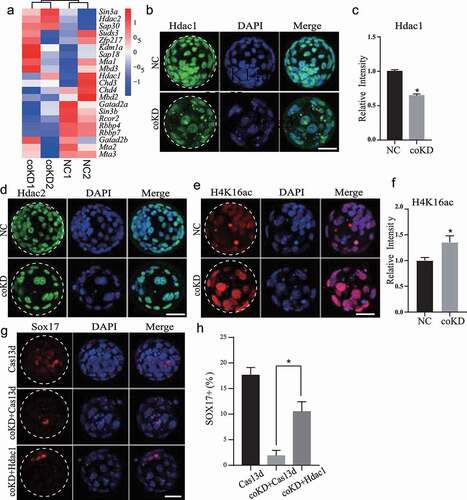
We then asked if ectopic expression of HDAC1 could rescue the development of coKD embryos. We found microinjection of Hdac1 mRNA together with Rbbp4/7 siRNAs did not rescue the blastocyst rate, however, improved the percentage of SOX17-positive cells (). The data suggest RBBP4/7 plays a stage-specific role during morula-to-blastocyst transition and regulates PE development partly through HDAC1.
Besides histone deacetylases HDAC1/2, histone demethylase LSD1 also interacts with RBBP4/7 to form the core components of multiple transcriptional corepressors, including NuRD and CoREST [Citation13]. LSD1 can specifically remove methyl groups of H3K4me1/2 and H3K9me1/2. We sought to identify if the function of LSD1 is compromised after knocking down Rbbp4/7 and found unchanged H3K9me2 at blastocyst stage in coKD embryos (Fig. S7A). Meanwhile, H3K4me3, a transcriptional activation mark, also exhibited no significant difference (Fig. S7B).
H3.3 is increased in embryos deficient of both RBBP4 and 7 from morula stage.
RNA-seq results show that H3f3b mRNA is increased in coKD but not in individual KD embryos at morulae stage ()). H3f3b is one of H3.3-encoding genes. In consistent with the change in mRNA, IF results displayed global increase of H3.3 at both morula and blastocyst stage in coKD but not in individual KD groups () and S8), suggesting a regulatory role of RBBP4/7 in H3f3b expression. It seems possible that the observed effects could be directly caused by RBBP4/7 since the phenotype could be observed at morula stage when cell numbers are similar between control and coKD embryos.
Figure 6. H3.3 is increased in embryos deficient of both RBBP4 and 7. A. Double knockdown of Rbbp4/7, not individually, leads to an increase in H3f3b mRNA abundance. B-E. Immunostaining against H3.3 in NC and coKD morula (b and c) and blastocysts (d and e). Scale bar = 50 μM. F and G. Immunostaining analysis of H3K27ac level in NC and coKD embryos. Scale bar = 50 μM. Asterisks refer to statistical significance (P < 0.05).
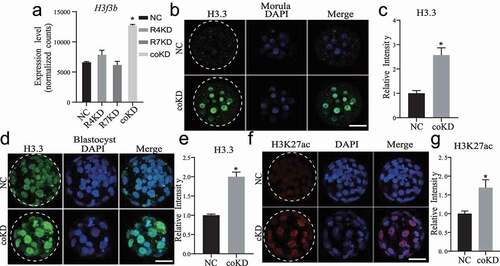
Loss of H3.3 causes decreased global H3K27ac both in mouse [Citation20] and Drosophila [Citation21]. Our IF analysis showed H3K27ac level is unchanged in coKD at morula stage (Fig. S6B), however, H3K27ac level is enhanced at blastocyst stage (), but not in individual KD groups (Fig. S6C). It is possible that RBBP4/7 regulate H3K27ac indirectly since the effect was only observed at blastocyst stage, when the cell number is compromised in coKD groups.
H3.3 and H3K27ac are enriched in promoter regions of RBBP4/7 target genes.
To ask if the increased H3.3 could be a mechanism explaining the dysregulated gene expression in RBBP4/7-deficient embryos, we analysed the distribution of H3.3 at those dysregulated genes using public datasets [Citation22]. Analysis of these ChIP-seq data in embryonic stem cells and 8-cell embryos in mice both indicate the enrichment of H3.3 at the promoter region of those dysregulated genes (,b)).
Figure 7. H3.3 and H3K27ac are enriched in promoter regions of RBBP4/7 target genes. A. Average H3.3 signal density (RPKM) around the transcription start site (TSS) regions (±2.5 Kb) for upregulated and downregulated genes in 8-cell mouse embryos and embryonic stem cells (mESC). B. Average H3K27ac signal density (RPKM) around the transcription start site (TSS) regions (±2.5 Kb) for upregulated and downregulated genes in coKD morula and NC morula.
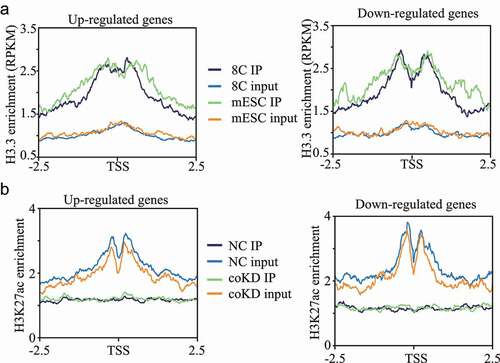
We also performed ultra-low-input ChIP-seq analysis of H3K27ac and found that H3K27ac is also enriched in the promoter regions with a slight reduction in coKD morula compared with NC (data not shown). Specifically, we found that H3K27ac enrichment in the promoter regions are both slightly reduced for up- and downregulated genes, suggesting the changes in the expression of dysregulated genes is not mediated through H3K27ac at morula stage.
Discussion
The present work demonstrated a functional redundant role for RBBP4 and 7 in fostering preimplantation development in mice. Depletion of both RBBP4 and 7 led to an embryonic mortality during morula-to-blastocyst transition in conjunction with a disrupted transcriptomic profile. Mechanistically, we documented three abnormal molecular events contributing to the developmental failure. First, the cell cycle was blocked with increased apoptosis and genome instability. Second, the early lineage specification event was dramatically perturbed with defects including abnormal OCT4 and SOX17 pattern and aberrant Hippo pathway. Meanwhile, critical epigenetic factors, including HDAC1 and histone variant H3.3, were dysregulated.
RBBP4 and 7 are two highly homologous proteins that exist together in multiple transcriptional complexes [Citation13]. Both genes are expressed throughout preimplantation development [Citation14,Citation23]. We found that independent knockdown of Rbbp4 and 7 does not affect blastocyst formation, suggesting a redundant role during preimplantation development [Citation6]. Indeed, the compromised development of coKD embryos could be observed at morula-to-blastocyst transition. The remaining coKD blastocysts also fails to form outgrowths. These data indicate RBBP4 and 7 are functional redundant during preimplantation development. We also documented compensatory roles of RBBP4 and 7 participating in the regulation of lineage specification factors, cell cycle, and epigenetic factors, further consolidating our conclusions. Such molecular redundancy has also been observed for HDAC1 and 2 knockdown in mouse preimplantation development [Citation6].
RNA-seq analysis revealed that the number of upregulated genes is greater than the one of downregulated genes in coKD groups. This result could be attributed to the fact that RBBP4/7 are present together with HDAC1/2 in multiple transcriptional repressive complexes, such as NuRD, CoREST, and SIN3A. Furthermore, results revealed that the number of DEGs caused by Rbbp4/7 coKD is significantly less than the one in Hdac1/2 coKD and Sin3a KD morula (). Meanwhile, only a small portion of the dysregulated genes was also affected by Hdac1/2 coKD or Sin3a KD (). This result indicates that HDAC1/2 and SIN3A play a more broad role in regulating gene expression in mouse preimplantation development.
Interestingly, we found H3.3 abundance is greatly improved during morula-to-blastocyst transition in coKD groups. It has been shown that accumulation of the histone variant H3.3 across the genome is associated with gene activation [Citation24] and open chromatin [Citation25]. H3.3 is enriched for active histone modifications including H3K4me3 and H3K27ac. Enrichment of H3.3 at promoter regions was observed for RBBP4/7 target genes, suggesting that RBBP4/7 regulates these target genes likely through H3.3 deposition at their promoters. We further found H3K27ac is also enriched in the promoters of these target genes; however, there are no obvious changes of H3K27ac enrichment in coKD morula. This result implies that the dysregulation of RBBP4/7 target genes is not due to H3K27ac content changes at their promoters.
In summary, our data show that RBBP4 and 7 play compensatory and essential roles during preimplantation development. The functional role is likely mediated through the regulation of cell proliferation, apoptosis, and epigenetic factors.
Materials and methods
Mouse embryo culture
Superovulation was induced by PMSG (San-Sheng pharmaceutical Co. Ltd., Ningbo, China) and hCG (San-Sheng pharmaceutical Co. Ltd., Ningbo, China) injection at the dose of 10 IU with 46–48 h apart to B6D2F1 (C57BL/6 × DBA2, Beijing Vital River Laboratory Animal Technology Co., Ltd.) female mice (8–10 weeks old). Upon hCG injection, the super-ovulated mice were then co-caged with B6D2F1 stud male mice. 20–22 h later, putative zygotes were collected from B6D2 F1 female mice and the enclosed cumulus cells were removed by using hyaluronidase (Sigma, St Louis, MO, USA). Embryos were cultured in KSOM and incubated at 37°C and 5% CO2 in humidified air.
Outgrowth
Blastocysts were collected on day 4 after hCG injection and incubated individually in DMEM (Gibco) containing 10% FBS (Gibco) on a 48-well plate coated with 0.1% Gelatin (Gibco).
siRNA synthesis and microinjection
siRNA-mediated gene silencing in mouse preimplantation embryos was carried out as published previously [Citation6,Citation8]. siRNA oligo sequences were designed and synthesized by GenePharma (Shanghai, China). Basic Local Alignment Search Tool (BLAST) analysis was performed for all siRNAs and non-specific targeting was not found. The sense and antisense oligo sequences of the siRNAs are presented in Table S1.
siRNAs were delivered into the cytoplasm of zygote by using a Piezo-drill (Eppendorf, Germany) and Eppendorf transferman micromanipulators. siRNA at a dosage of 20 µM was filled up into the microinjection pipette and then a constant flow was made and tuned to enable the successful microinjection. Around 10 pL of siRNA was microinjected into the cytoplasm of zygotes. In control groups, embryos were microinjected with a non-specific siRNA with the same dosage. The efficacy, efficiency, and the specificity of these siRNAs in mouse preimplantation embryos were validated in our previous study [Citation6].
TUNEL
Embryos were briefly washed in 0.1% polyvinylpyrrolidone/phosphate buffer saline (PVP/PBS), fixed in 4% paraformaldehyde (PFA) for 1 h and permeabilized in PBS containing 0.5% Triton X-100 and 0.1% sodium citrate for 1 h. The permeabilized samples were then incubated with a buffer solution containing 2 mM dATP, TDT 10×, CoCl2, 0.5 units/μL terminal deoxynucleotidyl transferase enzyme and 0.5 mM FITC-dUTP for 1 h at 37°C in humidity. DNA was stained with 4′,6-diamidino-2-phenylindole (DAPI). Embryos were loaded onto slides and imaged with a Zeiss confocal microscope system (LSM880).
Immunofluorescence
Embryo samples were washed briefly in 0.1% PVP/PBS followed by fixation in 4% PFA/PBS for 25 min at room temperature. The fixed embryos were then permeabilized by treating with 0.2% Triton X-100 for 25 min and then blocked in 10% FBS/0.1% Triton X-100/PBS for 1 h. The incubation with primary antibodies (Table S2) were performed for 1 h at room temperature or overnight at 4°C and the treatment with secondary antibodies was at 37°C for 1 h. DNA was labelled with DAPI. Embryos were observed with a Zeiss LSM880 confocal microscope (Zeiss). The identical parameters, including pinhole size, exposure time, detection gain, were used for embryos in the same replicate. The images were taken at 5 µm intervals between optical sections under 40× objective lens. All confocal images were z-projected using Image J. For measuring the signal fluorescent intensity, the signal region was selected and the background subtracted to analyse the absolute intensity with Image J.
EdU staining
E4.5 embryos were cultured in 50 µM EdU in a 1:1 mix of KSOM at 37°C, in 5% CO2 for 30 min and then fixed in 4% paraformaldehyde in PBS 30 min at room temperature. EdU detection was carried out according to the manufacturer’s manual.
RNA-seq library construction and bioinformatic analysis
Embryos were collected at the morula stage as RNA-seq sample for each group (NC, R4KD, R7KD, and coKD groups; 15 morula per sample; n = 2). Extraction of the total RNA was performed by using Picopure RNA isolation kit (Life Technologies, Grand Island, NY, USA) based on the manufacturer’s instruction. Equal amount of GFP and RFP mRNA (2 × 106 copies) was supplemented into each sample as spike-in control prior to RNA extraction. oligo(dT)25 beads were used to isolate mRNAs. Sequencing libraries were constructed by using NEB Next Ultra RNA Library Prep Kit for Illumina (New England Biolabs) according to the manual. In brief, mRNAs were subject to fragmentation followed by reverse transcription. The cDNA library subsequently underwent end repair, poly(A)-tailing, adaptor ligation, and PCR amplification for 12–15 cycles. High-throughput sequencing was conducted by Novogene with Illumina Hiseq X Ten. The sequencing reads were compared to transcripts and analysed by using Salmon (https://combine-lab.github.io/salmon/) [Citation26]. Differential expressed genes were determined by employing DESeq2 package (P adjusted <0.05 and fold change >1.5 or <-1.5) [Citation27,Citation28]. Heatmaps were created by using pheatmap package in R. GO and KEGG analyses of differentially expressed genes were performed by the Database for Annotation, Visualization and Integrated Discovery (DAVID).
Ultra-low-input native ChIP-seq (ULI-NChIP-seq) and bioinformatic analysis
The ULI-NChIP-seq procedure was performed as previously described with minor modifications [Citation29]. Approximately 500 cells were used per reaction, and two biological replicates were performed for each group. 500 ng of histone H3K27ac antibody (Active Motif, cat. no. 39,133) was used for each immunoprecipitation reaction. The sequencing libraries were generated using the NEB Next Ultra II DNA Library Prep Kit (NEB) following the manufacturer’s instructions. Paired-end 150-bp sequencing was performed on a HiSeq X Ten (Illumina) at the Novogene Co., Ltd.
Sequencing reads were aligned to the mouse genome (mm10) using Bowtie2 with a default setting after removing adaptor sequences and low-quality reads by trimmomatic [Citation30]. PCR duplicates and low-mapping-quality (MapQ<20) reads were removed by Samtools. After confirming reproducibility between replicates, they were merged using Samtools for the analysis. H3K27ac and H3.3 enrichments in TSS were computed using deepTools computeMatrix. H3.3 ChIP-seq datasets (H3.3 data for mouse 8-cell embryo and mESC) were obtained from GSE139527.
Statistical analysis
At least three biological replicates were performed for all experiments unless otherwise stated. Differences between two groups were determined by two-tailed unpaired Student’s t tests. For quantification of IF results, nuclear areas were outlined based on DAPI signal and mean intensity measured using NIH ImageJ. Signal intensities were normalized to control embryos. A value of P < 0.05 was considered to be statistically significant. RNA-seq results and ChIP-seq were analysed with R (http://www.rproject.org). The graphs were constructed by GraphPad Prism 7.0 (GraphPad Software, USA). Results are stated as mean ± S.E.M.
Author contribution statement
K.Z. and L.X. performed study concept and design; X.L. and L.L. performed embryo microinjection and staining, writing, review, and revision of the paper; Y. D. and B.H. performed RNA-seq and ChIP-seq experiments and analysis. S.W. provided technical support and data interpretation. All authors read and approved the final paper.
Ethics statement
All experiments involving lab animals were performed according to the guidelines for the care and use of lab animals and approved by Zhejiang University.
Supplemental Material
Download Zip (1.4 MB)Acknowledgments
We thank all members of the Zhang laboratory for their helpful discussions.
Supplementary material
Supplemental data for this article can be accessed here
Disclosure statement
We declare that there are no any competing financial interests in relation to the work described.
Data Availability Statement
The datasets generated and/or analysed during the current study are being uploaded to NCBI GEO and will be available soon. https://www.ncbi.nlm.nih.gov/geo/query/acc.cgi?acc=GSE188298
Additional information
Funding
References
- Wilcox AJ, Harmon Q, Doody K, et al. Preimplantation loss of fertilized human ova: estimating the unobservable. Hum Reprod. 2020;35(4):743–750.
- Reese ST, Franco GA, Poole RK, et al. Pregnancy loss in beef cattle: a meta-analysis. Anim Reprod Sci. 2020;212:106251.
- Wiltbank MC, Baez GM, Garcia-Guerra A, et al. Pivotal periods for pregnancy loss during the first trimester of gestation in lactating dairy cows. Theriogenology. 2016;86(1):239–253.
- Rossant J. Genetic Control of early cell lineages in the mammalian embryo. Annu Rev Genet. 2018;52(1):185–201.
- Eckersley-Maslin MA, Alda-Catalinas C, Reik W. Dynamics of the epigenetic landscape during the maternal-to-zygotic transition. Nat Rev Mol Cell Biol. 2018;19(7):436–450.
- Zhao PP, Wang HN, and Wang H, et al. Essential roles of HDAC1 and 2 in lineage development and genome-wide DNA methylation during mouse preimplantation development. Epigenetics-Us. 2020;15(4):369–385.
- Andreu-Vieyra CV, Chen RH, Agno JE, et al. MLL2 is required in oocytes for bulk histone 3 lysine 4 trimethylation and transcriptional silencing. Plos Biol. 2010;8(8):e1000453.
- Zhao PP, Li S, Wang HA, et al. Sin3a regulates the developmental progression through morula-to-blastocyst transition via Hdac1. Faseb J. 2019;33(11):12541–12553.
- Matoba S, Liu Y, Lu F, et al. Embryonic development following somatic cell nuclear transfer impeded by persisting histone methylation. Cell. 2014;159(4):884–895.
- Yw Q, Yc W, Re H Jr., et al. A retinoblastoma-binding protein related to a negative regulator of Ras in yeast. Nature. 1993;364(6438):648–652.
- Qian YW, Lee EY. Dual retinoblastoma-binding proteins with properties related to a negative regulator of ras in yeast. J Biol Chem. 1995;270(43):25507–25513.
- Murzina NV, Pei XY, Zhang W, et al. Structural basis for the recognition of histone H4 by the histone-chaperone RbAp46. Structure. 2008;16(7):1077–1085.
- Laugesen A, Helin K. Chromatin repressive complexes in stem cells, development, and cancer. Cell Stem Cell. 2014;14(6):735–751.
- Miao X, Sun T, Barletta H, et al. Loss of RBBP4 results in defective inner cell mass, severe apoptosis, hyperacetylated histones and preimplantation lethality in micedagger. Biol Reprod. 2020;103(1):13–23.
- Nicolas E, Morales V, Magnaghi-Jaulin L, et al. RbAp48 belongs to the histone deacetylase complex that associates with the retinoblastoma protein. J Biol Chem. 2000;275(13):9797–9804.
- Buchkovich K, Duffy LA, Harlow E. The retinoblastoma protein is phosphorylated during specific phases of the cell-cycle. Cell. 1989;58(6):1097–1105.
- Zenker J, White MD, Templin RM, et al. A microtubule-organizing center directing intracellular transport in the early mouse embryo. Science. 2017;357(6354):925–928.
- Nicolas E, Morales V, Magnaghi-Jaulin L, et al. RbAp48 belongs to the histone deacetylase complex that associates with the retinoblastoma protein. J Biol Chem. 2000;275(13):9797–9804.
- Zhang Y, Ng HH, Erdjument-Bromage H, et al. Analysis of the NuRD subunits reveals a histone deacetylase core complex and a connection with DNA methylation. Genes Dev. 1999;13(15):1924–1935.
- Martire S, Gogate AA, Whitmill A, et al. Phosphorylation of histone H3.3 at serine 31 promotes p300 activity and enhancer acetylation. Nat Genet. 2019;51(6):941-+.
- Leatham-Jensen M, Uyehara CM, Strahl BD, et al. Lysine 27 of replication-independent histone H3.3 is required for Polycomb target gene silencing but not for gene activation. Plos Genet. 2019;15(1):e1007932.
- Ishiuchi T, Abe S, Inoue K, et al. Reprogramming of the histone H3.3 landscape in the early mouse embryo. Nat Struct Mol Biol. 2021;28(1):38–49.
- Balboula AZ, Stein P, Schultz RM, et al. Knockdown of RBBP7 unveils a requirement of histone deacetylation for CPC function in mouse oocytes. Cell Cycle. 2014;13(4):600–611.
- Huang C, Zhu B. H3.3 turnover: a mechanism to poise chromatin for transcription, or a response to open chromatin? Bioessays. 2014;36(6):579–584.
- Chen P, Zhao JC, Wang Y, et al. H3.3 actively marks enhancers and primes gene transcription via opening higher-ordered chromatin. Gene Dev. 2013;27(19):2109–2124.
- Love MI, Huber W, Anders S. Moderated estimation of fold change and dispersion for RNA-seq data with DESeq2. Genome Biol. 2014;15(12):550.
- Patro R, Duggal G, Love MI, et al. Salmon provides fast and bias-aware quantification of transcript expression. Nat Methods. 2017;14(4):417–419.
- Sahraeian SME, Mohiyuddin M, Sebra R, et al. Gaining comprehensive biological insight into the transcriptome by performing a broad-spectrum RNA-seq analysis. Nat Commun. 2017;8(1):59.
- Brind’Amour J, Liu S, and Hudson M, et al. An ultra-low-input native ChIP-seq protocol for genome-wide profiling of rare cell populations. Nat Commun. 2015;6;6033.
- Bolger AM, Lohse M, Usadel B. Trimmomatic: a flexible trimmer for Illumina sequence data. Bioinformatics. 2014;30(15):2114–2120.