ABSTRACT
To compare the expression of microRNA-185-5p (miR-185-5p) in normal foetuses and in foetuses with late-onset growth restriction (FGR) and to determine the factors influencing this expression. In a prospective study, 40 foetuses (22 of them with late-onset FGR and 18 with normal growth) were scanned with Doppler ultrasound after week 35 and followed until birth. Subsequently, blood samples from umbilical cords were collected after delivery to evaluate the expression of miR-185-5p using real-time qPCR. Finally, multivariable regression analysis was applied to determine the clinical and ultrasonographic factors influencing miR-185-5p expression in both normal and late-onset FGR foetuses. In comparison with normal foetuses, late-onset FGR foetuses expressed upregulation of miR-185-5p (2.26 ± 1.30 versus 1.27 ± 1.03 2^-ddCt, P = 0.011). Multivariable regression analysis confirmed that cerebroplacental ratio (P < 0.05) was the only determinant of this overexpression. FGR foetuses overexpress miR-185-5p in relation to brain-sparing. Future studies will be needed to investigate the role of miR-185 in the management of late-onset FGR.
Introduction
Foetal growth restriction (FGR) is defined as the failure to reach the foetal growth potential [Citation1], determining a higher probability of perinatal morbidity and mortality with subsequent neurologic and cardiovascular consequences [Citation2,Citation3]. Late-onset FGR occurs after 34 weeks and is characterized by an unbalance between foetal demands and placental supply, resulting in low cerebroplacental ratio (CPR) values regardless of foetal birth weight (BW) [Citation4]. Although hypoxia in late-onset FGR may be subtle, it produces long-term consequences as it leads to suboptimal dendritic formation, brain underdevelopment and poorer cognitive status [Citation5–8]. As a result, diagnosis of late-onset FGR and prediction of adverse perinatal outcome becomes of key importance. Unfortunately, current models based on Doppler ultrasound alone or combined with serological markers have not proven to be accurate enough to perform clinical predictions [Citation9–13], making the search for new markers of FGR a crucial issue in foetal medicine.
MicroRNAs (miRNAs) are small RNA non-coding sequences with the ability to regulate gene expression by means of inhibition of translation or the promotion of mRNA degradation [Citation14,Citation15]. In cell culture and clinical experimentation, production of some miRNAs is characteristically influenced by hypoxia [Citation16,Citation17]. Among them, miRNA-185-5p (miR-185-5p) stands out for its ability to modulate the levels of vascular endothelial growth factor (VEGF) and its receptor (VEGFR) under the influence of hypoxia inducible factors (HIFs) [Citation18]. VEGF is in fact a mitogen for endothelial cells promoting angiogenesis in vivo and in vitro [Citation19,Citation20]. However, by far, its most interesting role is the action on the neuronal tissue where it behaves as a neurotrophic factor modulating neuronal plasticity and exerting neuroprotection against hypoxia [Citation21,Citation22]. Considering these relationships and in order to investigate new markers of chronic foetal hypoxia and brain damage, we evaluated miR-185-5p expression in normal foetuses and in foetuses affected with late-onset FGR.
Material and methods
Patient recruitment and Doppler-ultrasound examination
This was a prospective study of 40 foetuses conducted at the public tertiary maternity of the La Fe University and Polytechnic Hospital (Institution Review Board and Hospital Ethics Committee permission number 2016/0453). These foetuses underwent a foetal ultrasound examination between 35 and 41 weeks, which included a Doppler assessment of the umbilical artery (UA) and middle cerebral artery (MCA) pulsatility indices (PI). UA PI and MCA PI were recorded using colour and pulse Doppler according to earlier descriptions [Citation23,Citation24], while CPR was calculated as the simple ratio between MCA PI and UA PI [Citation25]. All pregnancies were delivered in 15 days or less after the scan, and only the last examination per foetus was included. In order to adjust for the effect of the GA, birth weight (BW) values were converted into local reference centiles [Citation26] adjusting also for foetal gender, and CPR values were converted into multiples of median (MoM) dividing each value by the 50th centile at each gestational age as earlier described [Citation23]. CPR medians (50th centile) were represented by the equation:
CPR 50th centile = −3.814786276 + 0.36363249 * GA – 0.005646672 * GA2
Where GA was gestational age in weeks with decimals.
All Doppler examinations were performed by the first author, a certified teaching expert in obstetric ultrasound by the Spanish Society of Obstetrics and Gynaecology, using General Electric Voluson® (E8/E6/730) ultrasound machines (General Electric Healthcare, Spain) with 2–8 MHz convex probes during foetal quiescence, in the absence of foetal tachycardia and keeping the insonation angle with the examined vessels as small as possible, always below 30°C. GA was determined according to the crown-rump length in the first trimester. Multiple pregnancies and those complicated by congenital foetal abnormalities were excluded. Gestational characteristics including maternal age, weight, height, body mass index (BMI), parity, number of gestations and ethnicity were collected at examination together with ultrasound parameters. Afterwards, labour data including BW, BW centile, mode of delivery, 5 min Apgar score, cord arterial pH and baby’s destiny were collected at birth.
Ponderal and haemodynamic characteristics of the study population
For comparison purposes, the study evaluated two groups of foetuses:
1-. Late-onset FGR: characterized by a BW<3rd centile or alternatively a BW between the 3rd and 10th centile, plus an abnormal Doppler (represented by a CPR < 0,6765 MoM) [Citation27].
2-. Normal foetuses: characterized by a BW>3rd centile plus a normal Doppler (represented by a CPR > 0,6765 MoM) [Citation27].
Other foetuses, like those with normal BW plus an abnormal CPR, were not included in the study, although they represented an interesting group for future research.
Foetal RNA extraction and quantification
Blood samples were collected from all foetuses in EDTA tubes. Each one was centrifuged at 3000–3500 rpm for 10 min to separate the plasma and this was stored at −80°C until RNA extraction. Cell-free total RNA (including miRNAs) was isolated from 500 µL of plasma using the miRNeasy Serum/Plasma kit (Qiagen®, Valencia, CA USA) following the manufacturer’s protocol. The concentration of cell-free total RNA (including miRNAs) was subsequently quantified using NanoDrop One® UV-spectrophotometer (Thermo Scientific, Wilmington, DE USA).
Real-time qPCR (RT-qPCR) validation from umbilical cord plasma
Reverse transcription reactions were performed using the TaqMan® miRNA Reverse Transcription kit and miRNA-specific stem-loop primers (Part No. 4366597, Applied Biosystems Inc. CA USA) and 100ng of input cell-free RNA in a 20 µL RT reaction. RT-qPCR reactions were performed in triplicate, in scaled-down 10-µL reaction volumes using 5 µL TaqMan® 2x Universal PCR Master Mix (Applied Biosystems Inc. CA USA) with No UNG, 0.5 µL TaqMan® Small RNA assay (20x) (Applied Biosystems Inc. CA USA), [hsa-miR-185-5p (002271)], 3.5 µL of nuclease free water and 1 µL of RT product. RT-qPCR was carried out on an Applied BioSystems QuantStudio5® thermocycler (Applied Biosystems Inc. CA USA). We used circulating hsa-miR-191-5p, one of the most stable miRNAs in terms of read counts in the discovery phase by NGS, and which has been used previously as an endogenous control to normalize the expression in plasma samples. All the fold change data were obtained using the delta-delta CT method (2− ΔΔCT) expressed as 2^-ddCt [Citation28].
Statistical study of microRNA expression
Several tests were performed to evaluate the expression of miR-185-5p. Firstly, we studied the presence of statistically significant differences between FGR and normal foetuses; subsequently investigating the parameters influencing this expression. To find these influences, we performed correlations between miR-185-5p expression and CPR MoM as well as BW centile. Afterwards, a multivariable linear regression analysis for the prediction of miR-185-5p expression was done, including as dependent variables several maternal and foetal parameters (age, smoking, pre-pregnancy weight, height, parity, sex, BW centile, CPR MoM, Apgar score at 5 min and cord arterial pH). Finally, for a better understanding, results were graphically expressed by means of contour graphs. Continuous and categorical variables were compared using Mann–Whitney U and Fisher tests. Correlations were done using Pearson coefficient (R2). P-values below 0.05 were considered significant. Graphs were constructed with R-software® (version 3.4.3) and GraphPad Prism® Mac:5.0. Statistical analysis were done with StatPlus® Mac:Pro 7.3.3.0.
Results
The characteristics of the study population are shown in . In summary, it included 40 foetuses scanned after 34 weeks, of which 16 (40%) were males. The mean maternal age, gravidity, parity and BMI were 33.2, 1.9, 0.5 and 23, while the mean GA at examination and delivery was 38.3 and 38.9 weeks. Most pregnancies initiated labour with induction (80%) due to poor growth, prolonged pregnancy, favourable obstetric conditions or premature rupture of membranes, and delivered spontaneously (50%). In addition, most neonates were born uneventfully, accompanying the mother to the maternity ward (82.5%). Finally, despite the important proportion of foetuses below the 10th centile (55%), no foetus presented Apgar score below 7 at 5 min or cord pH below 7.10.
Table 1. Study population descriptive analysis, N = 40.
compares normal versus FGR pregnancies. In summary, mothers in the FGR group were thinner (P < 0.05) and foetuses had a lower CPR MoM, (P < 0.0001), BW (p < 0.05) and BW centile (p < 0.0001). In addition, FGR foetuses were examined and delivered earlier (p < 0.01).
Table 2. Comparisons between foetal growth restriction and normal foetuses.
shows the distribution of measurements according to the CPR MoM and BW centile [Citation27]. 22 patients presented late-onset FGR, while 18 were foetuses with normal haemodynamic and ponderal features. As earlier described, an important correlation was seen between CPR MoM and BW centile (R2 = 0.62, P < 0.0001) [Citation27].
Figure 1. Scattergram representing the correlation between BW centile and CPR MoM. An important correlation existed between both parameters R2 = 0.62, P < 0.0001.
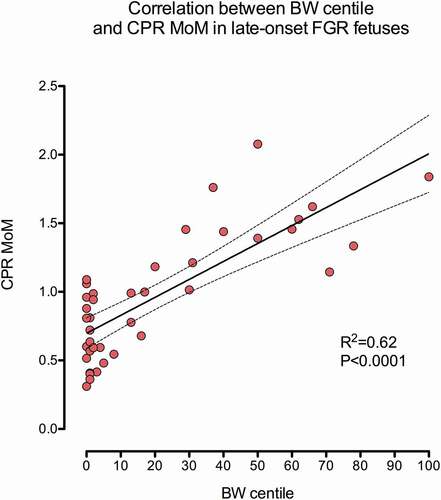
and evaluate miR-185-5p expression (fold change or 2^-ddCt) in FGR and normal foetuses. FGR foetuses showed a higher expression of miR-185-5p (2.2, SD 1.3 versus 1.3, SD 1.0, P = 0.011).
Figure 2. Box and Whiskers graph describing the expression of miR-185-5p in normal and late-onset FGR foetuses (fold change or 2^-ddCt). Whiskers represent the 10th and 90th centiles. Differences between both groups were statistically significant (Mann–Whitney U test, P = 0.011).
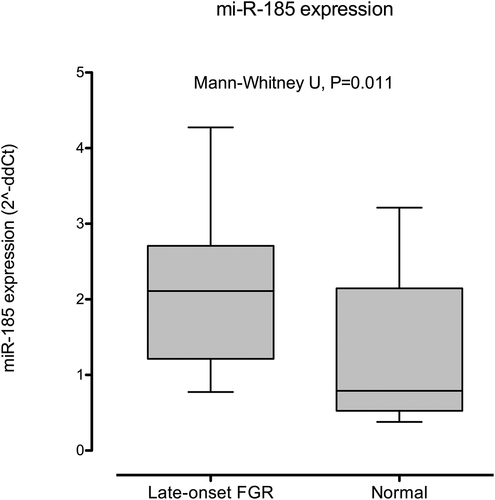
Finally, shows the multivariable regression analysis for the explanation of miR-185-5p expression. Only CPR MoM (a brain sparing marker) was selected as a significant parameter (P < 0.05). This finding was in line with , which shows the strong negative correlation between CPR MoM and miR-185-5p expression (R2 = 0.21, P = 0.003) and with , which depicts, in a contour graph and in coincidence with the regression analysis, how miR-185-5p expression inversely changes according to the CPR MoM.
Table 3. Multivariable linear regression model for the prediction of miR-185 expression.
Discussion
Principal finding
Analysis of umbilical blood at birth by means of qPCR showed that miR-185-5p was overexpressed in late-onset FGR foetuses and that this overexpression was inversely correlated with CPR MoM and not with BW centile.
Research implications
CPR MoM has emerged as the best determinant of adverse outcome at the end of pregnancy [Citation29–32]. However, its accuracy alone or associated with EFW centile and other clinical parameters is insufficient to perform clinical predictions, prompting the need to investigate new parameters of perinatal adversity. In the recent years, the interest has turned to the miRNA, small molecules that regulate human gene expression and may be differentially expressed in normal and pathological conditions [Citation33].
Recent research has proven that the hypoxic response is importantly mediated by the hypoxia-inducible transcription factors (HIF) −1, −2 and −3, which promote angiogenesis and resistance to apoptosis from the very early stages of embryo development [Citation34]. These types behave sequentially with HIF-1 driving the initial response, and HIF-2 as well as HIF-3 participating later during chronic hypoxia and vascular remodelling[Citation35]. HIF activates a myriad of vascular growth factors, crucial for vascular formation and embryo development like VEGF(A–D) and VEGFR [Citation36]. Although initial studies indicated that VEGF was an endothelial cell-specific factor, more recent findings discovered that, in parallel to its angiogenic role, VEGF was a strong promoter of neurogenesis, regulating proliferation, migration and axonal outgrowth. In fact neuronal survival is extremely sensible to VEGF variations and reduction by 25–30%, which can cause motor neuron degeneration [Citation37,Citation38]. VEGF transcription is regulated by many molecules, like inflammatory cytokines and oncoproteins as part of the hypoxic response. However, VEGF can also be influenced at post-transcriptional levels by micro-RNAs.
Influence of miRNAs in the hypoxic response
Recent studies have determined a link between hypoxia and microRNAs. Hypoxia downregulates specific miRNAs, overexpressing HIF and VEGF; thus promoting angiogenesis [Citation39]. In early hypoxia, miRNA downregulation contributes to HIF-1 accumulation, whereas in chronic hypoxia the miRNA expression is changed to maintain low HIF-1 function, and elevated HIF-2 as well as HIF-3 levels. Hence, miRNAs have the ability to govern the hypoxic HIF switch [Citation34,Citation39,Citation40]. Although the global mechanism for the miRNA downregulation during hypoxia remains unknown, one of the mechanisms is the inhibition of the DICER protein [Citation41], a monomeric ribonuclease crucial for micro-RNA biogenesis. Under hypoxia its expression is decreased, so the synthesis of inhibitory miRNAs diminishes and VEGF expression and angiogenesis are increased. Therefore, the microRNA pathway is functionally integrated in the cellular hypoxia response modulating the expression of HIFs, VEGF and VEGFR. Considering that miR-185 inhibits expression of HIF and VEGF [Citation42] by means of DICER inhibition, downregulation of miR-185 during hypoxia has the opposite effect, inducing the expression of VEGF and promoting angiogenesis, neurogenesis and cell proliferation. Conversely upregulation of miR-185 causes the contrary effect. This has practical consequences in oncology. As tumour growth and apoptosis is highly dependent of angiogenesis, miR-185 therapy has been proposed an inhibitor of angiogenesis and hence of tumour proliferation, converting miR-185 in a tumour suppressor [Citation42–44].
A plausible reason for the upregulation of miR-185
Our results showed that FGR foetuses presented ‘upregulated’ and not ‘downregulated’ levels of circulating miR-185 in blood samples from the umbilical cord. A priori, this might seem a contradiction with earlier works suggesting that miR-185 downregulation during hypoxia promoted VEGF angiogenesis and neuronal protection [Citation34,Citation39,Citation40]. However, this might not be absolutely true, as the mechanism by which miR-185 and VEGF interact might depend on the tissue affected and level of hypoxia.
Examples of the first mechanism are: 1- colorectal cancer, where miR-185 upregulation and not downregulation reduces survival, a phenomenon usually related with increased angiogenesis [Citation45], 2- clear cell renal carcinoma, where miR-185 upregulation is associated with higher expression of VEGFR-2 and higher micro-vessel density [Citation46] and 3- squamous cell carcinoma, where hypoxia has been found to decrease VEGF [Citation47]. Moreover, Huayu Tongmai, a Chinese herbal compound capable of treating diabetic angiopathy, exerts its anti-apoptotic effect on endothelial cells by means of miR-185 upregulation [Citation48]. Finally, concerning neurogenesis, and in patients with Parkinson disease, miR-185 upregulation was observed to inhibit autophagy and apoptosis of dopaminergic neurons [Citation49].
On the other hand, an example of the second mechanisms where the interaction between miR-185 and VEGF depends on the degree of hypoxia would be the action of VEGF in the lungs. Relative hypoxia activates HIF-2□, promoting VEGF secretion, lung development and maturation. However, profound hypoxia inactivates HIF-1□ and 2□, diminishing both VEGF and VEGFR expression thus impairing maturation and production of surfactant [Citation50]. In summary, increased levels of VEGF in umbilical cords, earlier described during hypoxia [Citation51], might also be compatible with upregulation of miR-185. Indeed, considering that miR-185 might target different genes in different cell types, cord levels of miR-185 might be only the expression of the overall biological resultant.
Clinical implications
In early-onset FGR, the issue is not the diagnosis but the prematurity, and how to balance it with the risk of foetal hypoxia. Conversely, in late-onset FGR growth anomalies are more subtle and difficult to detect and foetal Doppler, alone or combined with serological markers, has not proven to be accurate enough to predict adverse outcome, making the search of new perinatal markers a crucial issue in foetal medicine.
A practical consequence of the differential miR-185 expression would be the possibility to detect it in maternal blood in order to develop clinical tests. Hypoxia-related miRNA produced in the placenta have been detected in maternal blood [Citation52]. In this regard, if other miRNAs were able to cross the placental barrier and circulate between the mother and the foetus, miR-185b-5p might also be detected in maternal serum and become a marker of outcome in an isolated or combined way, consequently improving the diagnostic accuracy of the foetus with late-onset FGR.
Comparison with earlier reports studying miRNA in FGR
We could not find previous works studying miRNA in foetal blood especially in late-onset FGR foetuses. Only a handful of studies have dealt with miRNA during pregnancy and their samples were mostly collected from placental tissue or maternal blood [Citation53]. Moreover, most studies in FGR foetuses were done in early-onset FGR cases, frequently affected with preeclampsia, or sampled in the second trimester, much earlier in pregnancy [Citation54–57]. As a consequence, in many studies, despite the existence of promising results, the methodology was not appropriate to evaluate the true origin of the differentially expressed miRNA and the results could not be extrapolated [Citation57]. Of note, it is also important to underline that our population only included foetuses with late onset FGR, a distinct entity with a more cumbersome diagnosis. Despite these aspects, some facets in earlier investigations deserve to be considered. One is the lack of coincidence between different studies that show different miRNA expressions in the different groups of FGR foetuses, reflecting the extreme complexity of the mRNA action. Another is the relationship between miRNA expression and several mediators of angiogenesis, especially VEGF [Citation55,Citation58]; a feature also present in our study that was earlier suggested as an important link in the pathophysiology of FGR [Citation59].
Strengths and limitations
The main strengths of this study are its novelties, as we have been the first investigators to study miRNA in foetal blood, and the first to describe miR-185-5p overexpression in FGR as well as relating it with the action of HIF and VEG during the hypoxic response. Shortcomings are the specificity of foetal blood regarding organ production, absence of validation in a different population, the paucity of follow-up and the absence of further data related with neurocognitive evolution in childhood.
Conclusion
FGR foetuses overexpress miR-185-5p, a miRNA related with the hypoxia response that modulates VEGF metabolism and neuronal plasticity. Further work is needed to evaluate its levels in maternal blood in order to assess if it could improve the understanding of late-onset FGR and the prediction of neurocognitive disability.
Authors’ contributions
José Morales-Roselló designed the study, performed the ultrasound examinations, performed part of the statistical analysis and wrote the manuscript.
Eva María García Lopez, José Santiago Ibañez Cabellos and José Luis García Gimenez made the qPCR analysis, supervised the final manuscript and suggested valuable inputs to the text.
Gabriela Loscalzo performed data search and made notable contributions to the final text.
Antonio José Cañada Martínez performed part of the statistical analysis.
Alfredo Perales Marín supervised the manuscript and suggested valuable inputs to the text.
Data availability
The data that support the findings of this study are available from the corresponding author, JMR, upon reasonable request.
Statement of ethics
Institutional Review Board permission was obtained for this study (Reference 2016/453).
Acknowledgments
We would like to thank Patricia Roman Perez (midwife), Dr. Julia Desco (obstetrician) and Dr. Alicia Martinez Varea (obstetrician), for their valuable collaboration in recruiting the patients with FGR. In addition, we would like to thank the midwifery team and the personnel of the maternity operating rooms for their contribution in collecting blood samples.
Disclosure statement
José Luis García-Giménez owns stocks in EpiDisease SL (Spin-Off from the Consortium Center for Biomedical Network Research-Spanish Institute of Health Carlos III), an epigenetics company focused on the development of epigenetic biomarkers. Other authors report no conflict of interests.
Additional information
Funding
References
- American College of Obstetricians and Gynecologists. Practice bulletin no. 134: fetal growth restriction. Obstet Gynecol. 2013;121:1122–1133.
- Lees C, Marlow N, Arabin B, TRUFFLE Group, et al. Perinatal morbidity and mortality in early-onset fetal growth restriction: cohort outcomes of the trial of randomized umbilical and fetal flow in Europe (TRUFFLE). Ultrasound Obstet Gynecol. 2013;42:400–408.
- Yzydorczyk C, Armengaud JB, Peyter AC, et al. Endothelial dysfunction in individuals born after fetal growth restriction: cardiovascular and renal consequences and preventive approaches. J Dev Orig Health Dis. 2017;8:448–464.
- Figueras F, Gratacós E. Update on the diagnosis and classification of fetal growth restriction and proposal of a stage-based management protocol. Fetal Diagn Ther. 2014;36:86–98.
- Miller SL, Huppi PS, Mallard C. The consequences of fetal growth restriction on brain structure and neurodevelopmental outcome. J Physiol. 2016;594:807–823.
- Quezada S, Castillo-Melendez M, Walker DW, et al. Development of the cerebral cortex and the effect of the intrauterine environment. J Physiol. 2018;59:5665–5674.
- Triunfo S, Crispi F, Gratacos E, et al. Prediction of delivery of small for gestational age neonates and adverse perinatal outcomes by feto-placental Doppler at 37 weeks’ gestation. Ultrasound Obstet Gynecol. 2018;52:430–441.
- Morales-Roselló J, Khalil A, Fornés-Ferrer V, et al. Accuracy of the fetal cerebroplacental ratio for the detection of intrapartum compromise in nonsmall fetuses. J Matern Fetal Neonatal Med. 2019;32:2842–2852.
- Bligh LN, Alsolai AA, Greer RM, et al. Cerebroplacental ratio thresholds measured within 2 weeks before birth and risk of Cesarean section for intrapartum fetal compromise and adverse neonatal outcome. Ultrasound Obstet Gynecol. 2018;52:340–346.
- Bligh LN, Alsolai AA, Greer RM, et al. Pre-labour screening for intrapartum fetal compromise in low risk pregnancies at term: cerebroplacental ratio and placental growth factor. Ultrasound Obstet Gynecol. 2018;52:750–756.
- Carthew RW, Sontheimer EJ. Origins and mechanisms of miRNAs and siRNAs. Cell. 2009;136:642e55.
- Bartel DP. MicroRNAs: target recognition and regulatory functions. Cell. 2009;136:215e33.
- Ichihara A, Jinnin M, Yamane K, et al. microRNA-mediated keratinocyte hyperproliferation in psoriasis vulgaris. Br J Dermatol. 2011;165:1003e10.
- Vlachos IS, Paraskevopoulou MD, Karagkouni D, et al. DIANA-TarBase v7.0: indexing more than half a million experimentally supported miRNA:mRNA interactions. Nucleic Acids Res. 2015;43( Database issue):D153–9.
- Lewis BP, Burge CB, Bartel DP. Conserved seed pairing, often flanked by adenosines, indicates that thousands of human genes are microRNA targets. Cell. 2005;120:15–20.
- Bandara KV, Michael MZ, Gleadle JM. MicroRNA biogenesis in hypoxia. Microrna. 2017;6:80–96.
- Nallamshetty S, Chan SY, Loscalzo J. Hypoxia: a master regulator of microRNA biogenesis and activity. Free Radic Biol Med. 2013;64:20–30.
- Rupaimoole R, Wu SY, Pradeep S, et al. Hypoxia-mediated downregulation of miRNA biogenesis promotes tumour progression. Nat Commun. 2014 Oct;5:5202.
- Apte RS, Chen DS, Ferrara N. VEGF in signaling and disease: beyond discovery and development. Cell. 2019;176:1248–1264.
- Ferrara N. Vascular endothelial growth factor: basic science and clinical progress. Endocr Rev. 2004;25:581–611.
- Theis V, Theiss C. VEGF - a stimulus for neuronal development and regeneration in the CNS and PNS. Curr Protein Pept Sci. 2018;19:589–597.
- Ruiz de Almodovar C, Lambrechts D, Mazzone M, et al. Role and therapeutic potential of VEGF in the nervous system. Physiol Rev. 2009;89:607–648.
- Morales-Roselló J, Khalil A, Morlando M, et al. Doppler reference values of the fetal vertebral and middle cerebral arteries, at 19-41 weeks gestation. J Matern Fetal Neonatal Med. 2015;28:338–343.
- Acharya G, Wilsgaard T, Berntsen GK, et al. Reference ranges for serial measurements of umbilical artery Doppler indices in the second half of pregnancy. Am J Obstet Gynecol. 2005;192:937–944.
- Baschat AA, Gembruch U. The cerebroplacental Doppler ratio revisited. Ultrasound Obstet Gynecol. 2003;21:124–127.
- Figueras F, Meler E, Iraola A, et al. Customized birthweight standards for a Spanish population. Eur J Obstet Gynecol Reprod Biol. 2008;136:20–24.
- Morales-Roselló J, Khalil A, Morlando M, et al. Changes in fetal Doppler indices as a marker of failure to reach growth potential at term. Ultrasound Obstet Gynecol. 2014;43:303–310.
- Livak KJ, Schmittgen TD. Analysis of relative gene expression data using real-time quantitative PCR and the 2(-delta delta C(T)) method. Methods. 2001;25:402–408.
- Morales-Roselló J, Khalil A. Fetal cerebral redistribution: a marker of compromise regardless of fetal size. Ultrasound Obstet Gynecol. 2015;46:385–388.
- Morales-Roselló J, Khalil A, Morlando M, et al. Poor neonatal acid-base status in term fetuses with low cerebroplacental ratio. Ultrasound Obstet Gynecol. 2015;45:156–161.
- Khalil AA, Morales-Rosello J, Elsaddig M, et al. The association between fetal Doppler and admission to neonatal unit at term. Am J Obstet Gynecol. 2015;213:57.e1–7.
- Khalil AA, Morales-Rosello J, Morlando M, et al. Is fetal cerebroplacental ratio an independent predictor of intrapartum fetal compromise and neonatal unit admission? Am J Obstet Gynecol. 2015;213:54.e1–10.
- Cai M, Kolluru GK, Ahmed A. Small molecule, big prospects: microRNA in pregnancy and its complications. J Pregnancy. 2017;2017:6972732.
- Koh MY, Powis G. Passing the baton: the HIF switch. Trends Biochem Sci. 2012 Sept;37(9):364–372.
- Yang SL, Wu C, Xiong ZF, et al. Progress on hypoxia-inducible factor-3: its structure, gene regulation and biological function (Review). Mol Med Rep. 2015;12:2411–2416.
- Ramakrishnan S, Anand V, Roy S. Vascular endothelial growth factor signaling in hypoxia and inflammation. J Neuroimmune Pharmacol. 2014;9:142–160.
- Storkebaum E, Lambrechts D, Carmeliet P. VEGF: once regarded as a specific angiogenic factor, now implicated in neuroprotection. Bioessays. 2004;26:943–954.
- Shiote M, Nagano I, Ilieva H, et al. Reduction of a vascular endothelial growth factor receptor, fetal liver kinase-1, by antisense oligonucleotides induces motor neuron death in rat spinal cord exposed to hypoxia. Neuroscience. 2005;132:175–182.
- Serocki M, Bartoszewska S, Janaszak-Jasiecka A, et al. miRNAs regulate the HIF switch during hypoxia: a novel therapeutic target. Angiogenesis. 2018;21:183–202.
- Lisy K, Peet DJ. Turn me on: regulating HIF transcriptional activity. Cell Death Differ. 2008;15:642–649.
- Ho JJ, Metcalf JL, Yan MS, et al. Functional importance of Dicer protein in the adaptive cellular response to hypoxia. J Biol Chem. 2012;287:29003–29020.
- Lu ZJ, Lu LG, Tao KZ, et al. MicroRNA-185 suppresses growth and invasion of colon cancer cells through inhibition of the hypoxia-inducible factor-2α pathway in vitro and in vivo. Mol Med Rep. 2014;10:2401–2408.
- Agrawal R, Pandey P, Jha P, et al. Hypoxic signature of microRNAs in glioblastoma: insights from small RNA deep sequencing. BMC Genomics. 2014;15:686.
- Fan L, Tan B, Li Y, et al. Upregulation of miR-185 promotes apoptosis of the human gastric cancer cell line MGC803. Mol Med Rep. 2018;17:3115–3122.
- Akçakaya P, Ekelund S, Kolosenko I, et al. miR-185 and miR-133b deregulation is associated with overall survival and metastasis in colorectal cancer.Int. J Oncol. 2011;39:311–318.
- Yuan HX, Zhang JP, Kong WT, et al. Elevated microRNA-185 is associated with high vascular endothelial growth factor receptor 2 expression levels and high microvessel density in clear cell renal cell carcinoma. Tumour Biol. 2014;35:12757–12763.
- Eilertsen M, Pettersen I, Andersen S, et al. In NSCLC, VEGF-A response to hypoxia may differ between squamous cell and adenocarcinoma histology. Anticancer Res. 2012;32:4729–4736.
- Liu X, Wang D, Yang X. Huayu Tongmai Granules protects against vascular endothelial dysfunction via up-regulating miR-185 and down-regulating RAGE. Biosci Rep. 2018;38:BSR20180674.
- Wen Z, Zhang J, Tang P, et al. Overexpression of miR‐185 inhibits autophagy and apoptosis of dopaminergic neurons by regulating the AMPK/mTOR signaling pathway in Parkinson’s disease. Mol Med Rep. 2018;17:131–137.
- Tsao PN. Wei SC Prenatal hypoxia downregulates the expression of pulmonary vascular endothelial growth factor and its receptors in fetal mice. Neonatology. 2013;103:300–307.
- Malamitsi-Puchner A, Boutsikou T, Economou E, et al. Vascular endothelial growth factor and placenta growth factor in intrauterine growth-restricted fetuses and neonates. Mediators Inflamm. 2005;2005:293–297.
- Whitehead CL, Teh WT, Walker SP, et al. Circulating MicroRNAs in maternal blood as potential biomarkers for fetal hypoxia in-utero. PLoS One. 2013;8:e78487.
- Chiofalo B, Laganà AS, Vaiarelli A, et al. Do miRNAs play a role in fetal growth restriction? A fresh look to a busy corner. Biomed Res Int. 2017;2017:6073167.
- Awamleh Z, Gloor GB, Han VKM. Placental microRNAs in pregnancies with early onset intrauterine growth restriction and preeclampsia: potential impact on gene expression and pathophysiology. BMC Med Genomics. 2019;12:91.
- Thamotharan S, Chu A, Kempf K, et al. Differential microRNA expression in human placentas of term intra-uterine growth restriction that regulates target genes mediating angiogenesis and amino acid transport. PLoS One. 2017;12:e0176493.
- Higashijima A, Miura K, Mishima H, et al. Characterization of placenta-specific microRNAs in fetal growth restriction pregnancy. Prenat Diagn. 2013;33:214–222.
- Kim SH, MacIntyre DA, Binkhamis R, et al. Maternal plasma miRNAs as potential biomarkers for detecting risk of small-for-gestational-age births. EBioMedicine. 2020;62:103145.
- Li Y, Liu J. MicroRNA-206 predicts raised fetal growth retardation risk through the interaction with vascular endothelial growth factor in pregnancies. Medicine (Baltimore). 2020;99:e18897.
- Tang L, He G, Liu X, et al. Progress in the understanding of the etiology and predictability of fetal growth restriction. Reproduction. 2017;153:R227–R240.