ABSTRACT
Studies from both humans and animal models indicated that maternal chronic poor-quality diet, especially a high fat diet (HFD), is significantly associated with reduced bone density and childhood fractures in offspring. When previously studied in a rat model, our data suggested that maternal HFD changes epigenetic marks such as DNA methylation and histone modifications to control osteoblast metabolism. In mouse embryonic and postnatal offspring bone samples, a ChIP-sequencing (ChIP-Seq)-based genome-wide method was used to locate the repressive histone mark H3K27me3 (mediated via the polycomb histone methyltransferase, Ezh2) and expressive histone mark H3K27ac (p300/CBP mediated) throughout the genome. Using isolated mouse embryonic cells from foetal calvaria (osteoblast-like cells), H3K27me3 ChIP-Seq showed that 147 gene bodies and 26 gene promoters in HFD embryotic samples had a greater than twofold increase in H3K27me peaks compared to controls. Among the HFD samples, Pthlh and Col2a1 that are important genes playing roles during chondro- and osteogenesis had significantly enriched levels of H3K27me3. Their decreased mRNA expression was confirmed by real-time PCR and standard ChIP analysis, indicating a strong association with Ezh2 mediated H3K27me3 epigenetic changes. Using embryonic calvaria osteoblastic cells and offspring bone samples, H3K27ac ChIP-Seq analysis showed that osteoblast inhibitor genes Tnfaip3 and Twist1 had significantly enriched peaks of H3K27ac in HFD samples compared to controls. Their increased gene expression and association with H3K27ac were also confirmed by real-time PCR and standard ChIP analysis. These findings indicate that chronic maternal HFD changes histone trimethylation and acetylation epigenetic marks to regulate expression of genes controlling osteoblastogenesis.
Introduction
In human and animal models, studies have shown that chronic maternal or postnatal early life high fat diet (HFD) impacts the risk of chronic diseases in adult offspring [Citation1,Citation2], and it has persistent and detrimental effects on postnatal adult offspring bone quality [Citation3–6]. The HFD-induced maternal environment permanently changes foetal organ structure and system functions during critical periods in utero, which often coincide with intervals of rapid cell division and differentiation [Citation7]. Epigenetic mechanisms are currently hypothesized to be responsible for these foetal changes. We have previously provided evidence suggesting that such maternal HFD-associated epigenetic mechanisms are also involved in the regulation of bone development [Citation8]. In order to design and implement interventional approaches to improve bone quality and outcomes across the lifespan of offspring born to HFD mothers, epigenetic mechanisms linking the impact of maternal HFD to the regulation of foetal bone cell development and postnatal bone formation need to be investigated.
Expression of certain genes in the genome can be permanently dysregulated without altering the base sequence of DNA: e.g., through histone modifications (trimethylation and acetylation), DNA methylation, chromatin remodelling, the expression of non-coding RNA, and perhaps ubiquitination [Citation9–11]. In contrast to genetics, these instant gene expression regulatory mechanisms are known as epigenetics. Epigenetic mechanisms are important in regulating the differentiation of different types of cells during both prenatal and postnatal tissue development. Maternal nutrition appears to influence epigenetic alterations in offspring during both pre- and postnatal life and programs gene expression in key metabolic pathways, such as fatty acids and glucose metabolism [Citation12–15]. Emerging evidence suggests that maternal obesity caused by excessive consumption of a high-calorie/HFD has a profound influence on the health of cardiovascular and skeletal system of the offspring during foetal development and later-on into adulthood [Citation16–19]. Epigenetic control on osteoblast and osteoclast gene expression, bone development, and homoeostasis, as well as in the onset and progression of musculoskeletal diseases has also been shown to be increasingly common [Citation9,Citation20]. We have recently reported that maternal HFD might affect foetal osteogenic cell behaviours and differentiation through increasing DNA methylation and histone modification [Citation21]. However, the influence of HFD-induced maternal obesity on epigenetic regulation of osteoblast differentiation through histone trimethylation or acetylation has not been fully explored.
Studies have suggested that histone H3 lysine 27 trimethylation (H3K27me3) is a repressive epigenetic histone modification to the DNA packaging protein histone H3 [Citation22]. This trimethylation is associated with transcriptional repression, and downregulation of nearby genes via the formation of heterochromatic regions. For example, the expression of Runx2 (RUNX Family Transcription Factor 2), a transcription factor and the master regulator of mesenchymal cells responsible for osteoblast differentiation, is under the control of H3K27me3 [Citation23]. Although there are many-known enzymes that can catalyse histone methylation [Citation24], H3K27me3 is distinct in that it has only one known methyltransferase: Ezh2, a polycomb group (PcG) protein enhancer of zeste homologue 2, which is responsible for the repression of many genes involved in development and cell differentiation [Citation25,Citation26]. For example, we previously discovered that Ezh2 activation by maternal HFD is significantly associated with decreasing of SATB2 (special AT-rich sequence-binding protein 2) gene expression [Citation9].
In contrast to H3K27me3, histone H3 lysine 27 acetylation (H3K27ac), another epigenetic modification to the DNA packaging protein Histone H3, is associated with higher expression of transcription and therefore considered as an active enhancer mark [Citation27]. This regulation occurs by inducing an open chromatin structure that correlates with gene activation. It is not known if increases in H3K27ac lead to epigenetic overexpression of target genes in embryonic osteoblastic cells and in the bone of adult offspring. We have previously presented evidence that HFD maternal obesity H3K27ac marks are mediated through the activation of p300/CBP and lead to increased senescence-associated gene expression in osteoprogenitors [Citation28]. p300/CBP are together chiefly responsible for the global acetylation of histone H3 residues K18 and K27 and contribute to other locus-specific histone acetylation events; however, it is unknown if such a histone acetylation event is reversible during postnatal development [Citation29]. H3K-associated foetal tissue programming may affect several generations [Citation30] making it a difficult phenomenon to target for disease intervention.
We propose here that maternal HFD in mice modifies both H3K27me3 and H3K27ac epigenetic marks to synergistically regulate osteoblastic gene expressions controlling osteoblastogenesis and bone formation.
Materials and methods
Animals, diets, and tissue sampling
Female 4-week-old C57BL6/J mice were purchased from Jackson Laboratory (https://www.jax.org/). Subsequently, these mice were divided into two groups: one group of mice received control semi-purified AIN-93 G control diet (17% fat diet corn oil) and the other group of mice received a high-fat diet (HFD) (by calories, 25% protein, 45% fat, and 30% carbohydrates). After 12 weeks of diet intervention (pre-pregnancy), mice were time-impregnated (n = 12 per group) by control male mice, and dam diets either HFD or control were continued during pregnancy [Citation5]. Pregnant mice were individually housed in an Association for Assessment and Accreditation of Laboratory Animal Care-approved animal facility at the Arkansas Children’s Research Institute (Little Rock, AR) with constant humidity, lights on from 06:00 to 18:00 hr, at 22°C. All animal procedures were approved by the Institutional Animal Care and Use Committee at the University of Arkansas for Medical Sciences (UAMS, Little Rock, AR). One set of pregnant HFD and control diet mice were sacrificed, and embryos were taken at gestational day 17.5 for analysis. The remainder of pregnant HFD and control diet mice were continued on diet regimens until pups were born. Male and female offspring from either HFD or control diet dams were weaned at 21 d of age onto either HFD or control diet, and diets were continued for 14 weeks.
Isolation of foetal calvarial cells
Pregnant female mice were sacrificed on day 17 postcoitum (with 0 considered the day of the positive vaginal smear) and foetuses collected. For the isolation of foetal calvarial cells, sequential collagenase digestion of calvarial tissue was performed, and cells from second and third digestion were collected and pooled. The procedure was similar to isolation of neonatal mouse and rat calvarial osteoblastic cells as previously published [Citation9].
Chromatin-immunoprecipitation sequencing (ChIP-seq) and standard ChIP analysis
The genome-wide localizations of histone modifications, spanning varying transcriptional states, were analysed via ChIP-seq in foetal calvarial cells from HFD dams and control diet dams, as well as in 17 weeks old offspring bone samples (L4 vertebrae). ChIP grade antibodies for H3K27me3 (Active Motif) and H3k27ac were incubated with ChIP-grade protein A/G magnetic beads. The procedure for standard ChIP-seq and ChIP assay using H3K27me3 (ChIP grade from Cell Signalling) and H3k27ac (Cell Signalling) antibodies has been described previously [Citation9]. All primers used for ChIP assay were designed using Primer Express software 2.0.0 (Applied Biosystems).
Real-time reverse transcription-polymerase chain reaction
Cell RNA from foetal calvarial cells and total RNA from offspring bone tissue (L3 vertebrae) were extracted using TRI Reagent (MRC Inc., Cincinnati, OH) according to the manufacturer’s recommendation, followed by DNase digestion and column cleanup using QIAGEN mini columns [Citation5]. Reverse transcription was carried out using an iScript cDNA synthesis kit from Bio-Rad (Hercules, CA). All primers for real-time PCR and standard ChIP analysis used in this report were designed using Primer Express software 2.0.0 (Applied Biosystems), and they are presented in Supplemental Table 1.
Mouse embryo histology and immunostaining
Immunohistochemical staining for Twist1 was performed on cryosections of whole-mount mouse embryos either from HFD dams or control diet dams. Anti-Twist1 antibody from Abcam (#ab51502) was used for immune-staining for Twist1 expression in embryonic vertebrae and skeletal muscle tissues.
Data availability
Through functional genomic submissions via RT, all microarray ChIP sequencing data were deposited into The ArrayExpress team, assigned ArrayExpress accessions are E-MTAB-11718, E-MTAB-11712, E-MTAB-11713.
Statistics
Numerical variables were expressed as means ± SEM (Standard Error of Mean), and n represents the number of samples/group. For in vitro and ex vivo experiments, differences within groups were evaluated using t-test or one-way ANOVA followed by Tukey’s post hoc test comparing HFD diet group to the control diet group. P < 0.05 was considered significant. Real-time PCR analysis was conducted at least three independent times, and representative antibody immune-staining images were displayed.
Results
Identifying trimethylation marks of genes from embryonic calvaria cells using H3K27me3 ChIP-Seq
Chromatin was precipitated from embryonic calvaria cells from 17.5E foetus from either HFD dams or control diet dams as described in the method section. For analysing H3K27me3 ChIP-Seq data, the SeqMonk software developed by Babraham Bioinformatics was used to create probes to quantify the enriched read counts. A total of 12,530 enriched peaks were identified from HFD obese dams, while 29,339 enriched peaks were observed in the control diet dams using MACS (Model-based Analysis of ChIP-Seq) method. After probe deduplication, quantitation and normalization (according to the software user manual) there were 1,788 enriched peaks containing 1,238 genes that were statistically different (intensity difference p < 0.05, Supplemental Table 2 in Excel sheet) between HFD dams and control diet dams, suggesting that HFD dams have a great influence on H3K27me3 in their embryonic offspring. Among them, 147 gene bodies and 26 gene promoters from HFD dams had a greater than twofold increase in intensity compared to control diet dams. The top 45 genes with the most or least H3K27me3 marks are presented in a b. From these genes, those that are ‘bone-related’ are listed in .
Figure 1. Identification of H3K27me3 target genes affected by maternal HFD (high fat diet) regulating embryonic skeletal development. (a) and (b) representing top 45 genes and significantly H3K27me3 enriched or decreased in gene body (including gene promoter) using Heat Map analysis of ChIP-seq data from foetal calvaria cells either from HFD or control diet dams (pooled three per group). (c) Bone related genes with at least a 1.5 fold significantly changed H3K27me3 enrichment, Con, control; HF, HFD, numbers are detected and aligned peak values. (d) Pthlh was significantly enriched in HFD group for H3K27me3 within the gene body, especially in the promoter region based on the detection of a peak of enrichment (indicated by rectangles) by Illumina DNA sequencing. (e) Real-time PCR for Pthlh mRNA expression in total RNA from foetal calvaria cells either from HFD or control diet dams (6 per group), * p < 0.05 by t-test. (f) Col2al was significantly enriched in HFD group for H3K27me3 within the gene body, especially in the promoter region based on the detection of a peak of enrichment (indicated by rectangles) by Illumina DNA sequencing. (g) Real-time PCR for Col2al mRNA expression in total RNA from foetal calvaria cells either from HFD or control diet dams (6 per group), * p < 0.05 by t-test.
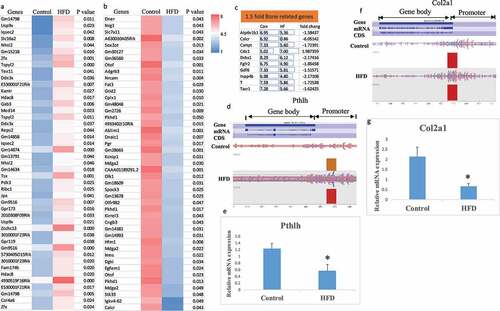
KEGG pathway analysis using Enrich showed that thyroid hormone signalling and enriched genes that are involved in breast cancer were the top two pathways influenced by maternal HFD, while gene ontology analysis showed that regulation of aldosterone biosynthetic process and positive regulation of DNA-dependent DNA replication were the top two GO biological processes influenced by maternal HFD. Additionally, genes involved in the regulation of insulin-like growth factor receptor signalling pathway were also affected (Supplemental Figure S1). A search for the influence of maternal HFD on genes involved in embryonic skeletal development using gene ontology definition terms containing osteoblast, osteoclast, ossification, chondrocyte, cartilage, and skeletal-related genes identified four bone-related genes; Bmp6, Wnt4, Pthlh (parathyroid hormone-like peptide), and Col2a1, that were significantly hyper-H3K27me3 methylated in foetal samples from HFD dams. Individual gene analysis showed that Pthlh and Col2a1 from HFD dams had significant enriched levels of H3K27me3 in their gene promoter regions compared with those from control dams (). Real-time PCR mRNA expression analysis showed significantly down-regulated Pthlh and Col2a1 mRNA expression from HFD dam samples (). Standard ChIP-PCR with H3K27me3 showed significant association between Pthlh, Bmp6, and Col2a1 with H3k27me3 (). Fold enrichment of association between Pthlh, Bmp6, and Col2a1 with H3K27me3 are listed in .
Figure 2. Maternal HFD enhances association of H3K27me3 with Pthlh, BMP6 and Col2al. (a) ChIP of foetal calvaria cells of mouse Pthlh, BMP6 and Col2al enhancer elements by specific anti H3K27me3 antibody. (b) ChIP of foetal calvaria cells of enrichments of Pthlh, BMP6 and Col2al after IP with H3K27me3 antibody. Fold enrichment relative to IgG. *, p < 0.05 by t-test HFD versus control diet dams.
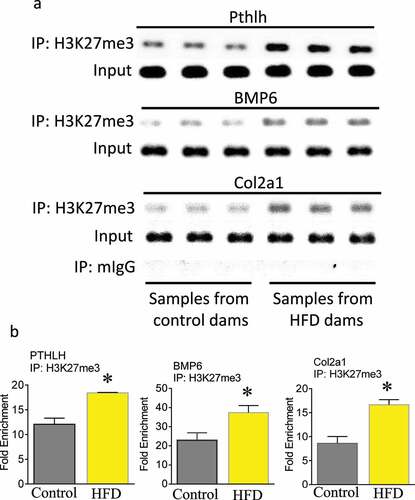
Identifying acetylation marks of genes from embryonic calvaria cells using H3K27ac ChIP-Seq
Using MACS method to analyse H3K27ac ChIP-Seq data from embryonic calvaria cells from E17.5 foetus from either HFD dams or control diet dams, a total of 21,494 enriched peaks were identified from HFD obese dams, while 20,950 enriched peaks were observed in the control dams. After probe deduplication, quantitation, and normalization (according to the software user manual) there were 2,317 enriched peaks containing 2,068 genes that were statistically different (intensity difference p < 0.05, Supplemental Table 3 in Excel sheet) between HFD obese dams and control dams, suggesting that HFD obese dams have a great influence on H3K27ac in this study. Among the 2,068 genes that were significantly different, 1,249 of the gene body enriched genes and 28 of the gene promoter enriched genes from HFD obese dams had a greater than twofold change in intensity when compared to control diet dams. The top 45 genes with the largest increase or decrease in H3K27ac marks when comparing control samples to HFD samples are presented in . We have previously reported that HFD significantly changed the expression of ‘senescence related’ genes [Citation21]; therefore, we searched the list of 2,068 genes that were significantly different for ‘senescence related’ genes and found Eef1e1, Nuak1, Rsl1d1, and Twist1 had significantly increased enrichment of H3K27ac ().
Figure 3. Identification of H3K27ac target genes affected by maternal HFD (high fat diet) regulating embryonic skeletal development. (a) and (b) representing top 45 genes significantly H3K27ac enriched or decreased in gene body (including gene promoter) using Heat Map analysis of ChIP-seq data from foetal calvaria cells either from HFD or control diet dams (pooled three per group). (c) Cell/tissue senescence related genes with at least a 1.5 fold significantly changed H3K27ac enrichment, Con, control; HF, HFD, numbers are detected and aligned peak values. (d) and (e) Tnfaip3 and Twist1 were significantly enriched in HFD group for H3K27ac within the gene body, especially in the promoter region based on the detection of a peak of enrichment (indicated by rectangles) by Illumina DNA sequencing. (f) Real-time PCR for Tnfaip3 and Twist1 mRNA expression in total RNA from foetal calvaria cells either from HFD or control diet dams (6 per group), * p < 0.05 by t-test. (g) Venn diagram shows 34 genes from foetal calvaria cells were significantly H3K27ac enriched and H3K27me3 enriched in their gene body or gene promoter by maternal HFD compared with maternal control diet.
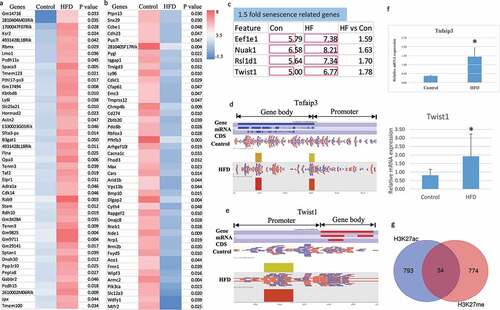
KEGG pathway analysis using Enrichr showed that breast cancer pathways were the top pathways influenced by HFD treatment, while gene ontology analysis showed that postsynaptic density organization and neuron differentiation were the top two GO biological processes influenced by HFD treatment (Supplementary table 2). To focus on searching for the influence of HFD treatment on gene expression involved in skeletal function, we identified 31 bone-related genes using gene ontology definitions containing osteoblast, osteoclast, ossification, chondrocyte, cartilage, and skeletal-related genes. Tumour necrosis factor alpha-induced protein 3 (Tnfaip3) and Twist1 were found to be hyper-H3K27ac acetylated () in foetal samples from HFD dams. Real-time PCR mRNA expression analysis showed significantly increased Tnfaip3 and Twist1 mRNA expression from HFD dam samples (). Indeed, among 827 hyper-H3K27ac acetylated and 808 hyper-H3K27me3 trimethylated genes, there are 34 genes that were both hyper-H3K27ac acetylated and hyper-H3K27me3 trimethylated ().
Standard ChIP-PCR with H3K27ac showed that in embryonic calvaria cells from maternal HFD samples there is a significant association between Tnfaip3 and Twist1 with H3K27ac (). Furthermore, immune-staining was performed using Twist1 antibody on cryosectioned embryonic spine and muscle tissue from control diet or HFD dams. HFD dam samples clearly show more intensive Twist1 red staining than those from control diet dams (under fluorescent microscopy either 20x or 40x magnification). Higher Twist1 expression was also shown in embryonic muscle tissue from HFD dams compared with control diet dams ().
Figure 4. Maternal HFD enhances association of H3K27ac with Tnfaip3 and Twist1. (a) ChIP of foetal calvaria cells of mouse Tnfaip3 and Twist1 enhancer elements by specific anti H3K27ac antibody. (b) ChIP of foetal calvaria cells of enrichments of Tnfaip3 and Twist1 after IP with H3K27ac antibody. Fold enrichment relative to IgG. *, p < 0.05 by t-test HFD versus control diet dams. Immune-staining using Twist1 antibody on cryosectioned embryonic spine (c) and muscle tissue (d) from control diet or HFD dams.
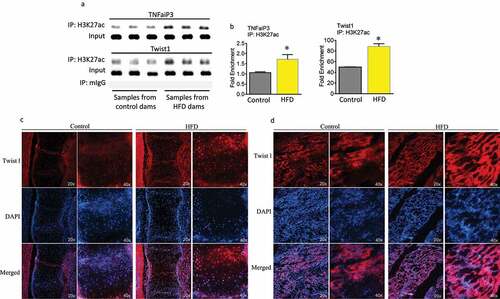
Identifying acetylation marks of genes from adult offspring bone using H3K27ac ChIP-Seq
H3K27ac marks from L3 vertebrae in the adult offspring of dams fed either control or HFD diet were analysed. Mice were divided into four dietary groups based on the maternal diet and offspring diet (three mice per group from four groups of adult offspring); maternal dam control diet with offspring control diet (CC, control–control), maternal dam control diet with offspring high fat diet (CH, control–HFD), maternal dam high fat diet with offspring control diet (HC, HFD–control) and maternal dam high fat diet with offspring high fat diet (HH, HFD–HFD). For each group, 2090 probes were used which encompass a total of 1391 unique genes. Among the 2090 probes, 529 (containing 347 unique genes) showed an increase in H3K27ac intensity; while 1561 probes (containing 1064 unique genes) showed a decrease in H3K27ac intensity (Supplemental Table 4 in Excel sheet). The top 45 genes that were the most significantly acetylated and deacetylated in the HC group compared with CC group are listed in respectively, while the top 45 genes that were the most significantly acetylated and deacetylated in the HH group compared with the CC group are listed in respectively. In , bone-related genes with a 1.5 fold or greater increase or decrease in H3K27ac epigenetic marks from all four groups were heat mapped. These genes are mainly involved in bone development, bone marrow development, bone mineralization, morphogenesis, and resorption. There are 10 genes that were affected by acetylation from both maternal and offspring HFD (). In , there are five genes from embryonic calvaria cells were both acetylated and trimethylated by maternal HFD. There are five genes that were found to be acetylated from embryonic calvaria cells with maternal HFD and from bone tissue of offspring born to HFD dams with offspring control diet (). Using standard ChIP analysis on offspring bone tissue, we were able to confirm that PTHLH, BMP6, and Col2a1 were trimethylated in embryonic tissues and offspring bone tissue by maternal HFD (). Interestingly, for PTHLH and Col2a1 H3K27me3 marks are increased by both maternal and offspring HFD. We were also able to confirm that TNFaiP3 and Twist1 were acetylated at histone 3 lysine 27 in both embryonic tissues and offspring bone tissues by maternal HFD. Standard ChIP analysis () and fold enrichment () showed maternal HFD or offspring HFD increased TNFaiP3 and Twist1 marks at H3K27ac.
Figure 5. Identification of H3K27ac target genes affected by maternal and postnatal offspring HFD regulating bone development. (a) and (b) comparison of represented top 45 genes with significantly H3K27ac enriched or decreased in gene body (including gene promoter) using Heat Map analysis of ChIP-seq data from offspring mouse spine L4 born to control diet dams with offspring control diet (CC) and to HFD dams with offspring control diet (HC) (pooled three per group). (c) and (d) comparison of represented top 45 genes with significantly H3K27ac enriched or decreased in gene body (including gene promoter) using Heat Map analysis of ChIP-seq data from offspring mouse spine L4 born to control diet dams with offspring control diet (CC) or to HFD dams with offspring HFD (HH) (pooled three per group).
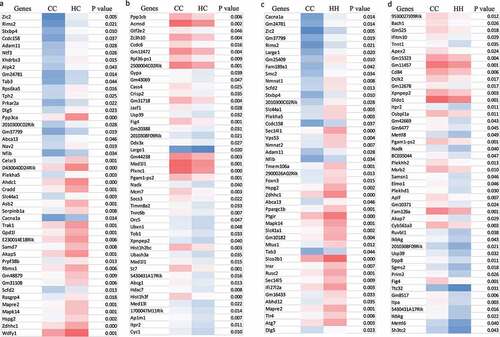
Figure 6. Comparison of H3K27ac target genes affected by maternal and postnatal offspring HFD regulating bone development. (a) representative significantly 1.5 fold changes of bone related genes subset from overall 1.5 fold different genes with H3K27ac enriched or decreased in gene body (including gene promoter) from all four groups, CC, control diet dams with offspring control diet; CH, control diet dams with offspring HFD; HC, HFD dams with offspring control diet; HH, HFD dams with offspring HFD (pooled three per group). (b) Venn diagram shows 10 genes were overlapped from offspring mouse spine L4 with significantly H3K27ac enriched in their gene body or gene promoter by maternal or offspring HFD or both maternal and offspring HFD. (c) Venn diagram shows 5 genes were overlapped from offspring mouse spine L4 and foetal calvaria cells with significantly H3K27ac and H3K27me3 enriched in their gene body or gene promoter by maternal HFD with offspring control diet (CH vs CC) and maternal HFD (HF vs Con). (d) Venn diagram shows 5 genes were overlapped from foetal calvaria cells with significantly H3K27ac and offspring mouse spine L4 with H3K27ac enriched in their gene body or gene promoter by maternal HFD.
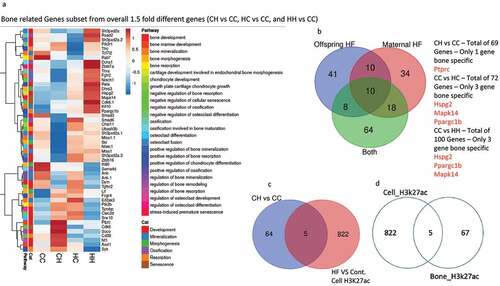
Figure 7. Maternal HFD enhances association of H3K27me3 with Pthlh, BMP6 and Col2al, and enhances association of H3K27ac with Tnfaip3 and Twist1 in offspring mouse spine L4. (a) ChIP of offspring mouse spine L4 of mouse Pthlh, BMP6 and Col2al enhancer elements by specific anti H3K27me3 antibody from four groups, Cont-Cont, dam control diet with offspring control diet; HFD-Cont, dam HFD with offspring control diet; Cont-HFD, dam control diet with offspring HFD; HFD-HFD, dam HFD with offspring HFD. (b) ChIP of offspring mouse spine L4 of enrichments of Pthlh, BMP6 and Col2al after IP with H3K27me3 antibody. Fold enrichment relative to IgG. (c) ChIP of offspring mouse spine L4 of mouse TNFaiP3 and Twist1 enhancer elements by specific anti H3K27ac antibody. (b) ChIP of offspring mouse spine L4 of enrichments of TNFaiP3 and Twist1 after IP with H3K27ac antibody. Fold enrichment relative to IgG.
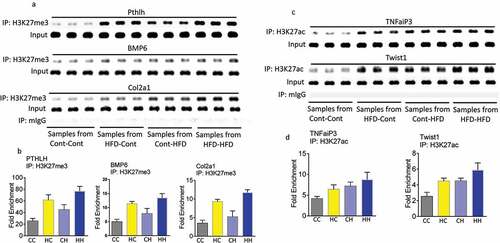
Discussion
Epigenetics can be defined as inheritable, but it remains controversial whether epigenetic processes such as histone modifications and cytosine methylation are irreversible exclusively. In fact, maternal HFD or other nutritional constraints during pregnancy can alter the metabolic phenotype of the offspring by means of epigenetic regulation of specific genes, and this can be passed on to future generations [Citation31]. Previous studies on how HFD-induced obesity can affect epigenetic marks has focused on altered histone methylation and/or acetylation levels in genes involved in specific and general metabolic processes [Citation32]. In our current study, we took embryonic and postnatal offspring osteogenic bone tissues from a maternal HFD-feeding mouse model, and H3K27me3 and H3K27ac marks were determined throughout the genome. We identified that maternal HFD changes the H3K27me3 and H3K27ac marks for a significant number of genes from offspring bone tissue. While a maternal HFD affects many systems and the expression of a variety of genes, osteoblastogenesis-associated Pthlh and Col2a1 are among the genes that are most significantly affected, showing a twofold decrease in expression and significantly enriched H3K27me3 marks. On the other hand, Tnfaip3 and Twist1, which are known negative regulators of osteoblastogenesis, were found to have their gene transcription significantly upregulated when enriched H3K27ac marks were present in embryonic and offspring bone tissue from maternal HFD dams. These findings are significant, providing rationale for further research to focus on determining specific factors associated with bone development that were altered by maternal HFD feeding and mechanisms of maternal HFD-induced persistent bone loss in offspring. Currently, mechanistic insights into how maternal HFD leads to poor bone quality in offspring and accelerated bone loss during adult life of offspring are limited. Our results may provide some new insights into how maternal HFD affects bone development in offspring and could lead to the development of possible approaches to targeting specific genes that are responsible for poor bone quality in offspring.
While postnatal bone mineralization within the skeletal envelope is impacted by a variety of factors such as local paracrine molecules and hormones travelling through the bloodstream [Citation33], dietary factors, and lifestyle such as the amount of physical activity; prenatal bone development is predominantly influenced by maternal nutrition. It has been hypothesized that intrauterine programming of bone development via maternal HFD results in an increased risk of osteoporosis in adulthood [Citation7]. Although there is no convincing clinical data on determining the effect of maternal HFD per se on bone quantity and quality in offspring postnatal, feeding a HFD rich in saturated fat and cholesterol to experimental rodents (both maternal and postnatal) has consistently been shown to inhibit bone formation [Citation34,Citation35]. Previous animal data indicated that inhibition of perinatal skeletal formation by HFD consumption during gestation might be associated with DNA hyper-methylation, suggesting intrauterine foetal skeletal developmental programming [Citation5]. In the current study, we have shown evidence that maternal HFD changes the H3K27me3 and H3K27ac epigenetic marks to regulate the expression of genes involved in osteoblastogenesis and bone development.
We have screened out and identified that four skeletal development-related genes, Bmp6, Pthlh (parathyroid hormone-like peptide), and Col2a1 had a significant enriched level of H3K27me3, and a corresponding decreased mRNA expression in embryonic samples from maternal HFD dams indicating a strong association with Ezh2/H3K27me3 regulation. BMP6 and is well-known gene associated with osteoblastogenesis [Citation36,Citation37]. It has been shown that BMP6 strongly induces alkaline phosphatase (ALP) activity in cells of osteoblast lineage, and that overexpression of BMP6 accelerates osteoblast differentiation [Citation36]. It has also been reported that overexpression of BMP6 is associated with Wnt4 provides protection against bone loss and increases osteoblastogenesis [Citation38]. Whether overexpression of BMP6 or Wnt4 could rescue maternal HFD-feeding-associated decreased osteoblastogenesis and bone formation will need to be studied in the future. The findings of decreased Pthlh and Col2a1 gene expression in embryonic skeletal tissues from maternal HFD dams are also interesting. It is known that Pthlh encodes a parathyroid hormone-related protein, which plays a role in regulation of endochondral and trabecular bone mass formation, and production of strong cortical bone [Citation39]. The loss-of-function of Pthlh leads to low bone mass, chondrodystrophic phenotype, and impaired osteoblast differentiation in mice. Col2a1 gene encodes the alpha I chain of type II collagen and mutation of this gene can cause many different skeletal abnormality patterns including hip disorders and decreased chondrocyte differentiation in mice [Citation40]. Embryonic skeletogenesis is mostly through chondrogenesis, therefore, decreased Pthlh and Col2a1 gene expression indicates that maternal HFD effects an early stage of osteoblastogenesis most likely through Ezh2/H3K27me3 regulation. It is possible that manipulation of Ezh2 may solve the problems of decreased expression for these osteogenic genes and potentially prevent maternal HFD-induced decreases of osteoblastogenesis and postnatal bone formation. To test these hypotheses, specific studies focusing on exploring the relationships between Ezh2/maternal HFD and bone development will be required.
In our current study, we have also analysed H3K27ac marks in both embryonic and postnatal bone tissues using model-based analysis of ChIP-Seq method. Results suggested that maternal HFD has a great influence on regulating H3K27ac. Among them, 1,249 gene bodies and 28 gene promoters had a greater than twofold increase in samples from HFD dams compared to control diet dams. We identified 31 bone-related genes using gene ontology definitions containing the words osteoblast, osteoclast, ossification, chondrocyte, cartilage, and skeletal-related genes. Tnfaip3 and Twist1 were two genes whose expression was not only changed in embryonic skeletal tissues but also in adult offspring bone. Maternal HFD effects on H3K27ac regulation seem to be linked to osteogenic inhibitory genes. As we described previously [Citation21], increased H3K27ac is associated with increased p300/CBP activity. The mammalian p300/CBP is a pair of ubiquitously expressed, paralogous proteins that belong to a distinct family of histone acetyltransferases (HATs) [Citation41]. Although increased p300/CBP activity enhances H3K27ac and is consistent with previous evidence [Citation42], the activation of p300/CBP through maternal HFD, leading to increased H3K27ac of DNA from embryonic osteo-progenitors are novel findings. P300/CBP is essential for animal development as deletion of either one in mice leads to early embryonic lethality [Citation43]. These two HATs have been shown to function as transcription co-activators for hundreds of transcription factors including nuclear receptors (NRs).
Tnfaip3 (TNFa-induced protein 3) encodes protein A20, a cytoplasmic zinc finger protein and ubiquitin-modifying enzyme that has been characterized as a dual inhibitor of nuclear factor-κB (NF-κB) and mediates inflammatory signalling and cell death [Citation44]. The biological activity of Tnfaip3 is more likely associated with osteoclast function; however, there is evidence that Tnfaip3 is involved in osteoblastogenesis as well [Citation45]. In contrast, Twist1 is known to negatively regulate osteoblast differentiation in both mouse and human mesenchymal stem cells [Citation46]. Molecular silencing of Twist1 enhances osteogenic differentiation of murine mesenchymal stem cells; however, it has not been proven whether overexpression of Twist1 will suppress osteoblastogenesis. Nonetheless, we showed that maternal HFD not only leads to increased H3K27ac marks for Tnfaip3 and Twist1 in DNA isolated from embryonic osteogenic cells and adult offspring bone tissue, leading to increased expression of these two osteogenic suppressor genes. Relevant to the role of p300/CBP HATs, evidence has been shown that activation of p300/CBP leads to hyperacetylation of histone N-terminal tail lysines to correlate strongly with active gene transcription [Citation28]. P300/CBP are together chiefly responsible for the global acetylation of histone H3 residues K18 and K27 and contribute to other locus-specific histone acetylation events. H3K-associated embryonic tissue programming may affect several generations [Citation30] making it a difficult phenomenon to target for disease intervention. In our current study, we present clear data that maternal HFD increases H3K27ac marks and leads to epigenetic overexpression of target genes in embryonic osteoblastic cells and in bone of adult offspring. It supports our hypotheses that maternal HFD increases H3K27ac in osteogenic cells to change osteoblastic cell differentiation and activity.
Maternal HFD, best known for predisposing not only dam but also offspring to obesity and metabolic diseases, has recently seen increased investigation in both animal models and humans for its effects on offspring tissue phenotype. We have shown previously that regardless of postnatal HFD challenge, adult offspring from HFD dams have significantly suppressed bone formation. We have presented evidence suggesting that suppressed bone formation in adult offspring from HFD dams is a phenomenon of early bone involution/degeneration and may be in part due to histone acetylation, i.e., epigenetic regulation of genes involved in osteoblast differentiation or activity. For this reason, we have performed H3K27ac ChIP-Seq in offspring bone samples from four groups: maternal HFD with offspring control diet (HC) or HFD; maternal control diet with offspring HC or HFD. We have attempted to determine if those osteo-associated genes acetylated in embryos with maternal HFD have an effect on bone development when compared to offspring with control diet. We have observed that the genes described above, Tnfaip3 and Twist1, remained H3K27 acetylated in DNA of bone from control diet offspring born to HFD dams. Acetylation of those genes was also amplified in bone from offspring with HFD born to HFD dams. This phenomenon is also true for genes with increased H3K27me3 epigenetic marks (Bmp6, Pthlh, and Col2a1).
In conclusion, these data strongly support our earlier published results that postnatal HFD accelerates bone loss in offspring born to HFD dams. However, additional work is needed to prove that increased DNA acetylation or trimethylation of those genes during embryonic stages are associated with persistent defects of bone development in postnatal offspring.
Author contributions
Jin-Ran Chen designed and performed the study, and wrote the paper; Haijun Zhao and Oxana P. Lazarenko performed cell, biochemical and molecular experiments, and in vivo sample analysis; Michael Blackburn helped to perform ChIP analysis, and Kartik Shankar contributed to the performance of experiments and study designs.
Study limitations
We have used mouse embryonic cells isolated from foetal calvaria (osteoblast-like cells) and postnatal offspring bone samples, and a ChIP-sequencing (ChIP-Seq)-based genome-wide method was used to locate the repressive histone mark H3K27me3 (mediated via the polycomb histone methyltransferase, Ezh2) and expressive histone mark H3K27ac (p300/CBP mediated) throughout the genome. We determined that chronic maternal HFD changes histone trimethylation and acetylation epigenetic marks to regulate expression of genes controlling osteoblastogenesis. There are study limitations if those identified genes are truly associated with maternal HFD. Future and additional works are needed to prove that increased DNA acetylation or trimethylation of those genes during embryonic stages are associated with persistent defects of bone development in postnatal offspring.
Supplemental Material
Download Zip (1.1 MB)Acknowledgement
This work was supported by the United States Department of Agriculture (USDA)/Agricultural Research Service (ARS), project number # USDA-ARS project 6026-51,000-010-05S, to the Arkansas Children’s Nutrition Center. We would like to thank the following people for their technical assistance: Matt Ferguson, Hoy Pittman, and Bobby Fay.
Disclosure statement
No potential conflict of interest was reported by the author(s).
Supplementary material
Supplemental data for this article can be accessed online at https://doi.org/10.1080/15592294.2022.2111759
Additional information
Funding
References
- Petersen SB, Rasmussen MA, Olsen SF, et al. Maternal dietary patterns during pregnancy in relation to offspring forearm fractures: prospective study from the Danish national birth cohort. Nutrients. 2015;7(4):2382–2400.
- Cole ZA, Gale CR, Javaid MK, et al. Maternal dietary patterns during pregnancy and childhood bone mass: a longitudinal study. J Bone Miner Res. 2009;24(4):663–668.
- Liang C, Oest ME, Jones JC, et al. Gestational high saturated fat diet alters C57BL/6 mouse perinatal skeletal formation. Birth Defects Res B. 2009;86(5):362–369.
- Kushwaha P, Khambadkone SG, Li M, et al. Maternal high‑fat diet induces long‑lasting defects in bone structure in rat offspring through enhanced osteoclastogenesis. Calcif Tissue Int. 2021;108(5):680–692. Online ahead of print.
- Chen JR, Zhang J, Lazarenko OP, et al. Inhibition of fetal bone development through epigenetic down-regulation of HoxA10 in obese rats fed high-fat diet. FASEB J. 2012;26(3):1131–1141.
- Barker DJ. The origins of the developmental origins theory. J Intern Med. 2007;261(5):412–417.
- Dennison EM, Harvey NC, Cooper C. Programming of osteoporosis and impact on osteoporosis risk. Clin Obstet Gynecol. 2013;56(3):549–555.
- Chen JR, Lazarenko OP, Blackburn ML, et al. Maternal obesity programs senescence signaling and glucose metabolism in osteo-progenitors from rat and human. Endocrinology. 2016;157(11):4172–4183.
- Chen JR, Zhao H, Lazarenko OP, et al. Maternal regulation of SATB2 in osteo-progeniters impairs skeletal development in offspring. FASEB J. 2020;34(2):2511–2523.
- Szyf ME. DNA methylation, and chromatin modifying drugs. Annu Rev Pharmacol Toxicol. 2009;49(1):243–263.
- Egger G, Liang G, Aparicio A, et al. Epigenetics in human disease and prospects for epigenetic therapy. Nature. 2004;429(6990):457–463.
- Collas P. Programming differentiation potential in mesenchymal stem cells. Epigenetics. 2010;5(6):476–482.
- Levin BE, Govek E. Gestational obesity accentuates obesity in obesity-prone progeny. Am J Physiol. 1998;275(4):R1374–R1379.
- King JC. Maternal obesity, metabolism, and pregnancy outcomes. Annu Rev Nutr. 2006;26(1):271–291.
- Shankar K, Harrell A, Liu X, et al. Maternal obesity at conception programs obesity in the offspring. Am J Physiol Regul Integr Comp Physiol. 2008;294(2):R528–R538.
- Boyle KE, Patinkin ZW, Shapiro AL, et al. mesenchymal stem cells from infants born to obese mothers exhibit greater potential for adipogenesis: the healthy start baby BUMP project. Diabetes. 2016;65(3):647–659.
- Cooper C, Harvey N, Cole Z, et al. Developmental origins of osteoporosis: the role of maternal nutrition. Adv Exp Med Biol. 2009;646:31–39.
- Samuelsson AM, Matthews PA, Argenton M, et al. Diet-induced obesity in female mice leads to offspring hyperphagia, adiposity, hypertension, and insulin resistance: a novel murine model of developmental programming. Hypertension. 2008;51(2):383–392.
- McCurdy CE, Bishop JM, Williams SM, et al. Maternal high-fat diet triggers lipotoxicity in the fetal livers of nonhuman primates. J Clin Invest. 2009;119(2):323–335.
- Liang C, Oest ME, Prater MR. Intrauterine exposure to high saturated fat diet elevates risk of adult-onset chronic diseases in C57BL/6 mice. Birth Defects Res B. 2009;86(5):377–384.
- Chen JR, Lazarenko OP, Zhao H, et al. Maternal obesity impairs skeletal development in adult offspring. J Endocrinol. 2018;239(1):33–47.
- Bannister AJ, Kouzarides T. Regulation of chromatin by histone modifications. Cell Res. 2011;21(3):381–395.
- Montecino M, Stein G, Stein J, et al. Multiple levels of epigenetic control for bone biology and pathology. Bone. 2015;81:733–738.
- Marmorstein R, Trievel RC. Histone modifying enzymes: structures, mechanisms, and specificities. Biochim Biophys Acta. 2009;1789(1):58–68.
- Kuzmichev A, Nishioka K, Erdjument-Bromage H, et al. Histone methyltransferase activity associated with a human multiprotein complex containing the Enhancer of Zeste protein. Genes Dev. 2002;16(22):2893–2905.
- Creyghton MP, Cheng AW, Welstead GG, et al. Histone H3K27ac separates active from poised enhancers and predicts developmental state. Proc Natl Acad Sci U S A. 2010;107(50):21931–21936.
- Tamura I, Maekawa R, Jozaki K, et al. Transcription factor C/EBPβ induces genome-wide H3K27ac and upregulates gene expression during decidualization of human endometrial stromal cells. Mol Cell Endocrinol. 2021;520:111085.
- Bose DA, Donahue G, Reinberg D, et al. RNA binding to CBP stimulates histone acetylation and transcription. Cell. 2017;168(1–2):135–149.e22.
- Kruhlak MJ, Hendzel MJ, Fischle W, et al. Regulation of global acetylation in mitosis through loss of histone acetyltransferases and deacetylases from chromatin. J Biol Chem. 2001;276(41):38307–38319.
- Rando OJ, Chang HY. Genome-wide views of chromatin structure. Annu Rev Biochem. 2009;78(1):245–271.
- Breton CV, Landon R, Kahn LG, et al. Exploring the evidence for epigenetic regulation of environmental influences on child health across generations. Commun Biol. 2021;4(1):769.
- Etchegaray JP, Mostoslavsky R. Interplay between metabolism and epigenetics: a nuclear adaptation to environmental changes. Mol Cell. 2016;62(5):695–711.
- Riggs BL, Khosla S, Melton LJ 3rd. Sex steroids and the construction and conservation of the adult skeleton. Endocr Rev. 2002;23(3):279–302.
- Parhami F, Tintut Y, Beamer WG, et al. Atherogenic high-fat diet reduces bone mineralization in mice. J Bone Miner Res. 2001;16(1):182–188.
- Chen JR, Zhang J, Lazarenko OP, et al. Soy protein isolates prevent loss of bone quantity associated with obesity in rats through regulation of insulin signaling in osteoblasts. FASEB J. 2013;27(9):3514–3523.
- Zhu F, Friedman MS, Luo W, et al. The transcription factor osterix (SP7) regulates BMP6-induced human osteoblast differentiation. J Cell Physiol. 2012;227(6):2677–2685.
- Li X, Li Z, Wang J, et al. Wnt4 signaling mediates protective effects of melatonin on new bone formation in an inflammatory environment. FASEB J. 2019;33(9):10126–10139.
- Han Y, You X, Xing W, et al. Paracrine and endocrine actions of bone-the functions of secretory proteins from osteoblasts, osteocytes, and osteoclasts. Bone Res. 2018;6(1):16.
- Ansari N, Ho PW, Crimeen-Irwin B, et al. Autocrine and paracrine regulation of the murine skeleton by osteocyte-derived parathyroid hormone-related protein. J Bone Miner Res. 2018;33(1):137–153.
- Barbieri O, Astigiano S, Morini M, et al. Depletion of cartilage collagen fibrils in mice carrying a dominant negative Col2a1 transgene affects chondrocyte differentiation. Am J Physiol Cell Physiol. 2003;285(6):C1504–12.
- Bedford DC, Brindle PK. Is histone acetylation the most important physiological function for CBP and p300? Aging (Albany NY). Aging. 2012;4(4):247–255.
- Zhong J, Ding L, Bohrer LR, et al. p300 acetyltransferase regulates androgen receptor degradation and PTEN-deficient prostate tumorigenesis. Cancer Res. 2014;74(6):1870–1880.
- Yao TP, Oh SP, Fuchs M, et al. Gene dosage-dependent embryonic development and proliferation defects in mice lacking the transcriptional integrator p300. Cell. 1998;93(3):361–372.
- Rusu I, Mennillo E, Bain JL, et al. Microbial signals, MyD88, and lymphotoxin drive TNF-independent intestinal epithelial tissue damage. J Clin Invest. 2022;132(5):e154993.
- Mabilleau G, Chappard D, Sabokbar A. Role of the A20-TRAF6 axis in lipopolysaccharide-mediated osteoclastogenesis. J Biol Chem. 2011;286(5):3242–3249.
- Bialek P, Kern B, Yang X, et al. A twist code determines the onset of osteoblast differentiation. Dev Cell. 2004;6(3):423–435.