ABSTRACT
Testicular germ cell tumours (TGCTs) are the most common tumours in young adults of European ancestry. The high heritability and the constantly increased incidence, which has doubled over the last 20 years, strongly suggest that both genetic and environmental factors are likely to shape the TGCT susceptibility. While genome-wide association studies have identified loci associated with TGCT susceptibility, the role played by environmental molecular vectors in TGCT susceptibility remains unclear. Evidence shows that sperm non-coding RNAs provide a good vision of the environmental stresses experienced by men. Here, to determine whether TGCT impacts the abundance of specific non-coding RNAs in sperm, small RNA deep sequencing analysis of sperm of 25 men aged between 19 and 42 years, diagnosed with (n = 16) or without (n = 9) TGCT was performed. The primary analysis showed no statistical significance in the sncRNA population between the TGCT and non-TGCT groups. However, when sperm physiological parameters were considered to look for differentially expressed sncRNA, we evidenced 11 differentially expressed sncRNA between patients and control which allow a clear discrimination between control and TGCT samples after Hierarchical Clustering analysis. Together, these findings indicate that sperm small non-coding RNAs abundance may have the potential for diagnosing men with TGCT. However, specific care should be taken regarding sperm physiological parameters of the TGCT patients. Hence, larger studies are needed to confirm our findings and to determine whether such a signature associates with the risks to develop TGCT.
Introduction
The testicular germ cell tumours (TGCT) have high heritability, which can be estimated at 37–49% [Citation1]. Its constantly increased incidence has doubled over the last 20 years [Citation2], strongly suggesting that both genetic and environmental factors are likely to shape genetic and non-genetic TGCT inheritances [Citation3,Citation4]. Although the genetic TGCT inheritance has been recently suggested by the identification of several susceptible loci associated with TGCT [Citation5], environmentally induced marks that may be involved in non-genetic (epigenetic) TGCT inheritance are still unknown [Citation6].
Sperm small non-coding RNAs (sncRNAs) could represent a step further into the identification of environmentally induced marks involved in epigenetic TGCT inheritance [Citation7]. Indeed, although spermatozoa are highly differentiated, transcriptionally inert cells, they contain a complex suite of coding and noncoding RNAs [Citation8]. The presence and abundance of sperm RNAs reflect not only sperm maturation and semen quality but also the paternal environment [Citation9–12]. Indeed, experimental studies conducted on animal evidence that environmental factors, such as unhealthy diet and psychotraumatic stress, can impact sperm sncRNA signature [Citation13–15]. Importantly, these molecules, once transmitted to the progeny, influence the phenotype of the resulting progenies, strongly suggesting a role of these small RNA molecules in epigenetic inheritance of these pathologies [Citation14–16]. However, whether testicular cancer impacts sperm sncRNAs profile is still an open question.
This is an important topic since significant progress has been made in the treatment of these cancers, leading to a significant improvement in the life expectancy [Citation17]. Given the young age of patients with TGCT [Citation2], most of them will plan fatherhood later in life and sperm banking must be proposed before any gonadotoxic treatment. Nevertheless, it is already known that this is particularly important given that men suffering from TGCT have an alteration in sperm parameters, in particular in sperm concentration and motility [Citation18] and that the therapies used to treat testis cancer are known to negatively impact fertility [Citation19]. Here, we aim to determine whether patients with TGCT exhibit a sperm-specific sncRNA profile. Identifying specific sncRNA signature might, on the one hand, help identify biomarkers of TGCT, and the possible involvement of small RNA in TGCT epigenetic inheritance. On the other hand, it may be a step forward to the molecular mechanism involved in epigenetic TGCT inheritance by altering the developmental competences of the future embryo and the long-term health of the conceptus.
Materials and methods
Study population
We used sperm from 16 individuals who had fertility preservation for TGCT and donated to the research. These sperm samples were registered and transferred to the GERMETHEQUE biobank (BB-0033–00081), a national multi-site biobank specialized in fertility, procreation, and human development site of Tenon hospital. Sperm from nine men with normal semen parameters and without any pathological context, also registered to the GERMETHEQUE biobank, were used as control. All the samples (study and control) came from the same centre, namely Tenon hospital. Patients and control were recruited over a period from 1995 to 2017. Several technicians performed the analyses, but all were trained in the same way (reproducibility test done).
Semen samples were collected, before orchiectomy, following masturbation into a sterile plastic cup. Semen volume, concentration, and progressive motility were evaluated according to the WHO 1999 and 2010 recommendations, depending on the year of recruitment, but the interpretation of the results was done according to WHO 2010 [Citation20,Citation21]. Sperm morphology was assessed using David’s classification [Citation22]. Information concerning the patients’ and controls’ clinical details and sperm parameters has been collated in Supplementary Table S1, but some data were missing. Summary information is presented in .
Table 1. Summary information of the participants.
The GERMETHEQUE biobank is a national multi-site biobank specialized in fertility, procreation, and human development. It provided sperm samples and their associated data to realize research project. GERMETHEQUE obtained consent from each patient and control to use their samples (CPP 2.15.27). The GERMETHEQUE pilotage committee approved the study design the 17/03/21. The Biobank has a declaration DC-2014–2202 and an authorization AC-2015–2350. The request number made to GERMETHEQUE is the 20210201 and its contract is referenced under the number: CHUT : 18 313 C.
The study protocol was approved by a local ethics committee (IRB CLEA-2021- 171) on 5 March 2021.
Sperm freezing
Semen samples of TGCT individuals and controls were frozen according to the same standardized protocol. The semen samples were diluted with cryoprotectant medium (SpermFreezTM, FertiPro NV, Belgium) and distributed into sperm straws (SpermFreezeTM, FertiPro, Beernem, Belgium). They were frozen in liquid nitrogen vapours using an automatic freezer (Nano-Digitcool, Cryo Bio System). The straws were then plunged and stored into liquid nitrogen.
RNA extraction
Two to ten sperm straws per individual were thawed and pooled for RNA extraction. The number of straws to be used was calculated to get at least 2 million spermatozoa. To remove any somatic contamination, the semen was centrifugated 10 min at 2000×g and the pellet washed twice in 1 ml of 0.1% sodium dodecyl sulphate (SDS) + 0.5% triton [Citation23]. A search for somatic cells using visual examination under microscope was performed, and no contamination was detected.
RNA extraction was performed by using 2 ml of Trizol (Invitrogen) according to the manufacturer’s recommendations with some modifications. Briefly, sperm pellets were homogenized in 100 µl of H2O supplemented with 1 µl β-mercaptoethanol and incubated 10 min at room temperature. The concentration of extracted RNA was measured by Qubit.
The quality of the extracted sperm RNA was determined by measuring five microRNAs (miRNAs – hsa-miR-103a-3p, hsa-miR-191-5p, hsa-miR-451a, hsa-miR-23a-3p by reverse transcription followed by quantitative PCR (RT-qPCR). These miRNAs are expressed in biofluids like serum, plasma, and also in human sperm [Citation24,Citation25]. For all samples, miRNAs could be detected (Supplementary Table S2A).
Small RNA sequencing and analysis
Twenty-five libraries and sequencing were performed by the Qiagen service provider, starting from 10 ng total RNA and using the QIAseq miRNA Library Kit®. Deep sequencing was performed in Illumina HISeq2000, targeting 20 M single-reads (75 bp) per sample. Information about the individual library sizes is given in Supplementary Table S2B.
Fastq files were checked with FASTQC for quality and trimmed, against known common Illumina adapter/primer sequences, using trimmomatic. The SmallRNAs UCAGenomix pipeline with Illumina adaptor trimming was used, and read sizes < 15 nucleotides were discarded. The SPORTS1.0 pipeline [Citation26], a small non-coding RNA annotation pipeline optimized for rRNA- and tRNA-derived small RNAs was used to align trimmed reads of 15–50 bp in length. We used the default settings and aligned to the human genome (Grch38), which are available on the SPORTS1.0 github (https//github.com/junchaoshi/sports1.0). Averages summarized over biotypes were based on the default annotation result output files.
Normalization and differential expression analysis were performed using the DESeq2 R package on the summary files generated with the SPORTS1.0 pipeline. The baseMean for each gene, the minimum mean count among all conditions, was at least 50 counts. The differentially expressed genes were calculated based on the fitted p values, where padj values correspond to p-value adjusted for multiple testing using Benjamini–Hochberg method for false discovery rate (FDR). Sequences were considered significant when FDR adjusted p ≤ 0.1.
Next-Generation Sequencing (NGS) experiments have been deposited in the SRA Database with accession number (PRJNA862464).
Ingenuity Pathways Analysis (IPA) was used to analyse the data.
Results
Sperm sncRNA profiles of TGCT patients
To determine whether sperm sncRNA profiles are modified in patients with testicular cancer, we deeply analysed the spermatic sncRNA expression of 16 men with TGCT and 9 control men without any testis disorders. The age and the physiological sperm parameters of patients and controls are summarized in . Sperm small RNA raw sequencing data were processed by the SPORTS1.0 pipeline, which allows the annotation and quantification of different sncRNAs, including tsRNAs (tRNA-derived small RNAs), rsRNAs (rRNA-derived small RNAs), piRNAs (piwi-associated small RNAs), YRNAs, and miRNAs. Principal Component Analysis (PCA) () and small RNA length distribution analyses () revealed that patient 10 is an outlier and, therefore, was removed from further analyses.
Figure 1. Sperm sncRNA expression from fresh frozen sperm samples of TGCT patients and control men. (a) Principal components analysis performed with all samples. (b) Small RNA length distributions of one representative TGCT patient, one representative non-TGCT man and the outlier TGCT patient n°10. (c) Pie chart representation of sncRNA population in control patients and control men, as indicated. Mean values are indicated in %. No statistical significance was found in the different sncRNA population between the TGCT and non-TGCT groups. (d) Heat map of a supervised hierarchical clustering based on the 100 most expressed sncRnas. In the heat map orange indicates relative high expression and blue indicates relative low expression. The coloured squares above the graph designate TGCT and non-TGCT samples. (e) Principal components analysis performed without patient n°10.
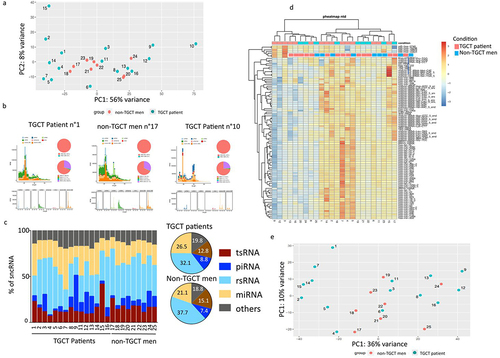
As summarized in , sperm sncRNA populations were mainly composed by rsRNAs (1/3), miRNAs (1/4), piRNAs (1/10), and tsRNAs (1/10). No Statistical significance was found in the sncRNA population between the TGCT and non-TGCT groups using ordinary two-way ANOVA with Sidak’s multiple comparisons test (). Indeed, the PCA and heatmap performed with the 100 most expressed sncRNAs did not discriminate between the 2 groups (). Furthermore, no differentially expressed sncRNA between TGCT patients and control men was evidenced by DeSEQ2 analysis (Supplementary Table S3).
Semen quality had a strong impact on sncRNA signature
Looking closely at the PCA distribution shown in , we observed a nice clusterization of two groups of TGCT patients which could be associated with primary semen parameter (sperm concentration, sperm progressive motility, and sperm vitality) alterations, pathologies known to be observed in a sub-population of TGCT patients [Citation27]. As shown in Supplementary Table S1, on all 15 study TGCT patients, 8 of these exhibited sperm parameters alterations (Supplementary Table S1). Interestingly, six out these TGCT patients were characterized by sperm parameter alterations, namely, patients 1, 2, 4, 5, 7, and 15 was quite well separated from the other group characterized by normal sperm parameters, namely, patients n°3, 6, 8, 9, 12, and 16 ( and Supplementary Table S1), suggesting that sperm parameters have an impact on sncRNA profiles. This observation was in accord with recent studies revealing clear associations between fertility/motility and sperm sncRNAs deregulation, including miRNAs and tRNA [Citation9–11,Citation28].
Identification of Differentially Expressed sncRnas in sperm between TGCT patients and non-TGCT men
Based on this observation, we decided to analyse separately the sncRNA profiles of these two groups of TGCT patients, namely TGCT patients characterized with normal sperm parameters (normal semen TGCT patients, n = 7) and TGCT patients characterized with low semen quality (namely one of the semen parameters is below WHO guidelines) (low semen TGCT patients, n = 8) and look for significant differentially expressed sncRNAs. When sncRNA profile of low semen TGCT patients was compared with that of non-TGCT men (), 229 sncRNAs differentially expressed (padj ≤0.1) were identified with 71 piRNA (57 down-regulated and 15 upregulated), 101 miRNAs (1 down-regulated and 100 upregulated), and 43 tRNA fragments (0 downregulated and 43 upregulated) (Supplementary Table S4). Interestingly, IPA identified significant changes in gene expression relevant to cell proliferation of tumours cell lines and HOTAIR regulatory pathway, described to be involved in several cancer development but also to sperm motility () [Citation29]. Although this comparison distinguished quite well the TGCT patients with low semen quality from the non-TGCT men, the identified differentially expressed sncRNAs might be the result of difference in terms of semen parameters and not due to cancer. To eliminate the potential influence of semen parameter alterations in the sncRNA profiles, TGCT patients exhibiting normal semen parameters () were selected, and their sncRNA profiles were compared with the sperm of healthy men (). This new analysis identified 11 differentially expressed sncRNAs between the two groups (FDR adjusted p ≤ 0.1) including 7 piRNA (5 are upregulated and 2 are downregulated), 3 downregulated tsRNAs, and 1 downregulated miRNAs ( and Supplementary Table S5). Furthermore, supervised hierarchical clustering based on these 11 differential sncRNAs discriminate quite well TGCT patients from non-TGCT men (). To determine whether these deregulated sncRNAs could be helpful as biomarker to differentiate TGCT samples from non-TGCT samples, the abundance of each sncRNA was statistically tested using the Mann–Whitney test. Interestingly, the abundance of three of them, namely, the miR-224, the piR-has-23,921, and the mature-tRNA-His-GTG, were found to be statistically significantly different between normal TGCT patients and non-TGCT patients (). Importantly, none of these deregulated sncRNA was found deregulated when low semen quality TGCT patient group was compared with non-TGCT group (Supplementary Table S4) indicating that semen parameters should be considered with great attention before performing sncRNA-seq analysis.
Figure 2. Influence of sperm motility on sperm sncRNA profiles. (a) Principal components analysis performed on TGCT patients with low semen quality (low TGCT patient) according to WHO standard semen analysis and non-TGCT men (b) IPA analysis performed with the sncRnas deregulated in low semen quality compared to non-TGCT men shows the deregulation of several pathways involved in cancer; Orange and blue symbols represent downregulated and upregulated pathways. Orange edges: relationships predicted as activating. Blue edges: relationship predictive as inhibitory. (c) Principal components analysis performed with TGCT patients and non-TGCT men, both groups characterized by normal semen quality, according to WHO standard semen analysis. (d) Heat map of a supervised hierarchical clustering based on the significantly differentially expressed sncRnas fragments (padj <0.1). In the heat map orange indicates relative high expression and blue indicates relative low expression. The coloured squares above the graph designate TGCT and non-TGCT samples. (e) Abundance of differentially expressed sncRnas, Mann–Whitney test were applied to calculate the p-values (indicated above each comparison).
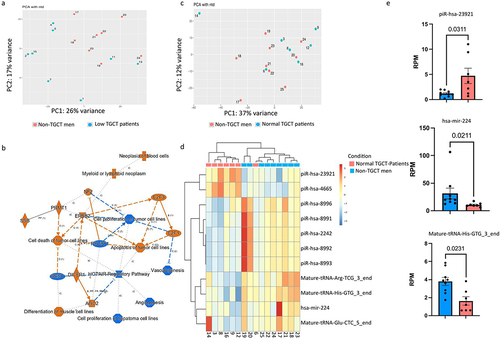
Table 2. Summary information of the selected participants based on their physiological sperm parameters.
Discussion
The current study is the first one to our knowledge reporting the impact of TGCT on sperm sncRNA profile. Indeed, although the impact of environmental factors, such as diet, chemical molecules, and psychological stress, was well established, that of TGCT on sncRNA profile was unknown. By performing small RNA-seq analysis of sperm collected from 25 men aged between 19 and 42 years with or without TGCT, we suggest that TGCT impacts on sperm sncRNAs expression profile. However, our data also demonstrate that sperm parameters greatly impacts sncRNA profiles. Since TGCT is associated with semen abnormalities (sperm concentration and sperm progressive motility) before the treatment [Citation27], extreme care should be taken when sperm-specific sncRNA signature of TGCT is sought. Here, to overcome this challenge, TGCT men with normal or low semen quality were analysed separately. Although 229 sncRNAs were found significantly deregulated when TGCT patients with low semen quality were compared with non-TGCT men, only 11 sncRNAs were found deregulated when sperm of TGCT patients with normal semen quality was compared with non-TGCT men. Importantly, none of these 11 sncRNAc were found deregulated when TGCT patients with low semen quality group were compared with non-TGCT group (Supplementary Table S4) indicating that semen parameters should be considered with great attention before performing sncRNA-seq analysis. Among the confirmed deregulated sncRNA, we identified one piRNA, one tRNA, and the miR-224. Although the role of piRNA and fragments of tRNA have been considered as potential biomarkers of cancer in several studies [Citation30,Citation31], the piRNA and the tRNA found deregulated in TGCT patients have never been specifically analysed. However, the miR-224 has been shown to be associated with several cancer [Citation32]. Whether this dysregulation could be involved in the pathogenesis or be altered by the TGCT needs to be further investigated.
Two types of TGCT are described in the literature, namely, the non-seminomatous germ cell tumours and the seminomatous germ cell tumours [Citation33]. Unfortunately, because of the unavailability of complete biological and oncogenic data, we could not make the difference between these two subtypes. Bridging transcriptomic signature and genetic studies, such as that performed by the Testicular Cancer Consortium comparing 10,159 patients and 179,683 controls allowing the identification of 22 susceptibility loci, is a step forward for the understanding of the development of TGCT cancer [Citation5].
It is important to specify that the current study was limited by the small patient number, the unavailability of the complete biological (especially the absence of abstinence days for some of the patients) and oncogenic patient data. A larger cohort with complete biological and clinical information is needed to fully validate our results.
Finally, considering the role of sperm sncRNAs in ensuring health development of the offspring [Citation16,Citation34–36], the high association between the potential target genes of the deregulated miRNAs and pathways involved in cancers, it would indicate that deregulation of sncRNAs in TGCT patients might potentially be an environmental vector of epigenetic TGCT susceptibility. Further studies are needed to validate this hypothesis.
Authors Contributions
VG and CD conceived and designed the project. VG, CD, PJI, MGS, NS, MT, IB, and RHH performed the experiments. CD, MGS, NS, MP, IB, and RHH collected the samples. VG wrote the manuscript. VG and CD contributed to the data analysis. VG, CD, PJI, MGS, NS, MP, RL, MT, IB, and RHH edited the paper. All the authors read and approved the final manuscript.
Supplemental Material
Download Zip (1.2 MB)Acknowledgments
This study was partly supported by research funding from the Canceropôle PACA, Institut National du Cancer and Région Sud, and the Fondation ARC (PJA 20191209377).
Disclosure statement
No potential conflict of interest was reported by the author(s).
Data availability statement
The data that support the findings of this study are available from the corresponding author (VG) upon reasonable request. SRA Database with accession number (PRJNA862464).
Supplementary material
Supplemental data for this article can be accessed online at https://doi.org/10.1080/15592294.2023.2241009
Additional information
Funding
References
- Litchfield K, Thomsen H, Mitchell JS, et al. Quantifying the heritability of testicular germ cell tumour using both population-based and genomic approaches. Sci Rep. 2015;5(1):13889. doi: 10.1038/srep13889
- Engholm G, Ferlay J, Christensen N, et al. NORDCAN–a Nordic tool for cancer information, planning, quality control and research. Acta Oncol. 2010;49(5):725–8. doi: 10.3109/02841861003782017
- Hemminki K, Li X. Familial risk in testicular cancer as a clue to a heritable and environmental aetiology. Br J Cancer. 2004;90(9):1765–1770. doi: 10.1038/sj.bjc.6601714
- von Eyben FE, Kristiansen K, Kapp DS, et al. Epigenetic regulation of driver genes in testicular tumorigenesis. Int J Mol Sci. 2023;24(4):4148. doi: 10.3390/ijms24044148
- Pluta J, Pyle LC, Nead KT, et al. Identification of 22 susceptibility loci associated with testicular germ cell tumors. Nat Commun. 2021;12(1):4487. doi: 10.1038/s41467-021-24334-y
- Beranger R, Le Cornet C, Schuz J, et al. Occupational and environmental exposures associated with testicular germ cell tumours: systematic review of prenatal and life-long exposures. PLoS One. 2013;8(10):e77130. doi: 10.1371/journal.pone.0077130
- Das MK, Ø P H, Haugen TB. Diverse roles and targets of miRNA in the pathogenesis of testicular germ cell tumour. Cancers (Basel). 2022;14(5):1190. doi: 10.3390/cancers14051190
- Gòdia M, Swanson G, Krawetz SA. A history of why fathers’ RNA matters. Biol Reprod. 2018;99(1):147–159. doi: 10.1093/biolre/ioy007
- Nätt D, Kugelberg U, Casas E, et al. Human sperm displays rapid responses to diet. PLoS Biol. 2019;17(12):e3000559. doi: 10.1371/journal.pbio.3000559
- Pantos K, Grigoriadis S, Tomara P, et al. Investigating The role of the microRNA-34/449 family in male infertility: a critical analysis and review of the literature. Front Endocrinol. 2021;12:709943. doi: 10.3389/fendo.2021.709943
- Rahbar S, Pashaiasl M, Ezzati M, et al. MicroRNA-based regulatory circuit involved in sperm infertility. Andrologia. 2020;52(1):e13453. doi: 10.1111/and.13453
- Jodar M, Selvaraju S, Sendler E, et al. The presence, role and clinical use of spermatozoal RNAs. Human Reproduction Update. 2013;19(6):604–624. doi: 10.1093/humupd/dmt031
- Chen Q, Yan M, Cao Z, et al. Sperm tsRnas contribute to intergenerational inheritance of an acquired metabolic disorder. Science. 2016;351(6271):397–400. doi: 10.1126/science.aad7977
- Gapp K, Jawaid A, Sarkies P, et al. Implication of sperm RNAs in transgenerational inheritance of the effects of early trauma in mice. Nat Neurosci. 2014;17(5):667–669. doi: 10.1038/nn.3695
- Grandjean V, Fourre S, De Abreu DA, et al. RNA-mediated paternal heredity of diet-induced obesity and metabolic disorders. Sci Rep. 2015;5(1):18193. doi: 10.1038/srep18193
- Rassoulzadegan M, Grandjean V, Gounon P, et al. RNA-mediated non-Mendelian inheritance of an epigenetic change in the mouse. Nature. 2006;441(7092):469–474. doi: 10.1038/nature04674
- Chovanec M, Cheng L. Advances in diagnosis and treatment of testicular cancer. BMJ. 2022;379:e070499. doi: 10.1136/bmj-2022-070499
- Rives N, Perdrix A, Hennebicq S, et al. The semen quality of 1158 men with testicular cancer at the time of cryopreservation: results of the French National CECOS Network. J Androl. 2012;33(6):1394–1401. doi: 10.2164/jandrol.112.016592
- Bates GE, Taub RN, West H. Fertility and cancer treatment. JAMA Oncol. 2016;2(2):284. doi: 10.1001/jamaoncol.2015.4143
- Organization WH. WHO laboratory manual for the examination of human semen and sperm-cervical mucus interaction. 4th ed. Cambridge: Cambridge University Press; 1999.
- WHO. WHO laboratory manual for the examination and processing of human semen. 5th ed. Geneva: World Health Organization; 2010.
- Auger J, Jouannet P, Eustache F. Another look at human sperm morphology. Hum Reprod. 2016;31(1):10–23. doi: 10.1093/humrep/dev251
- Bianchi E, Stermer A, Boekelheide K, et al. High-quality human and rat spermatozoal RNA isolation for functional genomic studies. Andrology. 2018;6(2):374–383. doi: 10.1111/andr.12471
- Schuster A, Tang C, Xie Y, et al. SpermBase: A database for sperm-borne RNA contents. Biol Reprod. 2016;95(5):99. doi: 10.1095/biolreprod.116.142190
- Xu H, Wang X, Wang Z, et al. MicroRNA expression profile analysis in sperm reveals hsa-mir-191 as an auspicious omen of in vitro fertilization. BMC Genomics. 2020;21(1):165. doi: 10.1186/s12864-020-6570-8
- Shi J, Ko EA, Sanders KM, et al. Sports1.0: A tool for annotating and profiling non-coding RNAs optimized for rRNA- and tRNA-derived small RNAs. Int J Genomics Proteomics. 2018;16(2):144–151. doi: 10.1016/j.gpb.2018.04.004
- Djaladat H, Burner E, Parikh PM, et al. The association between testis cancer and semen abnormalities before orchiectomy: a systematic review. J Adolesc Young Adult Oncol. 2014;3(4):153–159. doi: 10.1089/jayao.2014.0012
- Zhu H, Shyh-Chang N, Segre AV, et al. The Lin28/let-7 axis regulates glucose metabolism. Cell. 2011;147(1):81–94. doi: 10.1016/j.cell.2011.08.033
- Liu SJ, Dang HX, Lim DA, et al. Long noncoding RNAs in cancer metastasis. Nat Rev Cancer. 2021;21(7):446–460. doi: 10.1038/s41568-021-00353-1
- Ameli Mojarad M, Ameli Mojarad M, Shojaee B, et al. piRNA: A promising biomarker in early detection of gastrointestinal cancer. Pathol Res Pract. 2022;230:153757. doi: 10.1016/j.prp.2021.153757
- Zhu L, Li J, Gong Y, et al. Exosomal tRNA-derived small RNA as a promising biomarker for cancer diagnosis. Mol Cancer. 2019;18(1):74. doi: 10.1186/s12943-019-1000-8
- Chen W, Fan XM, Mao L, et al. MicroRNA-224: as a potential target for miR-based therapy of cancer. Tumour Biol. 2015;36(9):6645–6652. doi: 10.1007/s13277-015-3883-3
- Cheng L, Albers P, Berney DM, et al. Testicular cancer. Nat Rev Dis Primers. 2018;4(1):29. doi: 10.1038/s41572-018-0029-0
- Siklenka K, Erkek S, Godmann M, et al. Disruption of histone methylation in developing sperm impairs offspring health transgenerationally. Science. 2015;350(6261):aab2006. doi: 10.1126/science.aab2006
- Wei Y, Yang CR, Wei YP, et al. Paternally induced transgenerational inheritance of susceptibility to diabetes in mammals. Proc Natl Acad Sci U S A. 2014;111(5):1873–1878. doi: 10.1073/pnas.1321195111
- Raad G, Serra F, Martin L, et al. Paternal multigenerational exposure to an obesogenic diet drives epigenetic predisposition to metabolic diseases in mice. Elife. 2021;10:10. doi: 10.7554/eLife.61736