ABSTRACT
N6-Methyladenosine (m6A) plays a key role in the occurrence and development of various cancers. Fat mass and obesity‐associated protein (FTO) was is involved in multiple cancers owing to its demethylase activity, and the molecular mechanism underlying FTO-promoted bladder cancer proliferation and migration via the regulation of RNA stability requires further investigation. In the present study, FTO was upregulated in bladder cancer and related to poor prognosis. Gain- and loss-of-function experiments showed that the upregulation of FTO promoted bladder cancer proliferation and migration. Mechanistic studies showed that FTO enhanced the stability of signal transducer and activator of transcription 3 (STAT3) mRNA in an m6A-dependent manner, thereby increasing STAT3 expression, which subsequently promoted P-STAT3 expression and activated STAT3 signalling pathway. Overall, this study revealed that the critical role of FTO in the progression of bladder cancer and could provide a novel avenue to regulate oncogene STAT3.
KEYWORDS:
Introduction
Bladder cancer is the most common urological tumour, with more than 550,000 new bladder cases and 200,000 deaths worldwide every year [Citation1]. This is due to its high recurrence rates and invasiveness. It is reported that 1-year and 5-year recurrence rates of bladder cancer are 15–61% and 31–78% respectively [Citation2]. Despite the availability of novel early diagnostic and progressively advancing treatment methods, bladder cancer remains to be the main cause of tumour-associated mortality.
Bladder cancer progression is a complex process. In the tumour malignant progression, it is often found that various mechanisms of human body are disturbed. The stage of RNA modification is the main process of epigenetics [Citation3]. N6-Methyladenosine (m6A) as the most common internal RNA modification [Citation4], affects all aspects of RNA metabolism such as RNA stability, splicing, translation, and transport [Citation5]. Numerous studies have reported that the dysregulation of m6A is widely involved in tumorigenesis and tumour progression [Citation6–9]. The effect of Fat mass and obesity‐associated protein (FTO) on biological function and molecular mechanism have been verified in various types of cancer [Citation10,Citation11]. Particularly, FTO regulates tumour progression via mediating RNA stability in m6a-dependent manner [Citation12]. Thus, it is necessary to further explore the regulatory mechanism of FTO-mediated demethylation in bladder cancer.
Signal transducer and activator of transcription 3 (STAT3) is the most common member of the STAT family. STAT3 is involved in the development of various cancers, activated by various cytokines, growth factors, and oncogenic proteins [Citation13,Citation14]. In addition to phosphorylation, methylation can also regulate STAT3 transcriptional activity by altering the STAT3 state, thereby activating STAT3 signalling pathway in cancer [Citation15]. However, the effect of m6a modification on STAT3 signalling pathway in bladder cancer remains unclear.
The current study investigates new advancements in understanding of the functions and underlying molecular mechanisms of FTO in bladder cancer. Our finds provide a novel insight into treatment of bladder cancer.
Methods
Human samples
Bladder cancer tissue samples and their adjacent tissues were collected from Qilu Hospital of Shandong University between 2021 and 2022 (aged 51–79 years; 10 male and 2 female). All participants were informed of the study before surgery and provided consent. The Institutional Review Board of Qilu Hospital of Shandong University supported this study (approval no. 2020046). All the samples were immediately snap-frozen in liquid nitrogen and stored at −80°C.
Cell culture
The bladder cancer cell lines T24 and UM-UC-3 were purchased from the Chinese Academy of Science Committee Type Culture Collection Cell Bank (Shanghai, China). T24 cells and UM-UC-3 cells were cultured in 1640 medium (Gibco, USA) and Dulbecco’s Modified Eagle Medium (Gibco, USA), respectively. Medium was supplemented with 10% foetal bovine serum (Gibco, USA). All cell lines were cultured in a 5% CO2 humidified incubator at 37°C.
Bioinformatics analysis
The associations between FTO expression and the overall survival of patients with bladder cancer in The Cancer Genome Atlas (TCGA) database (https://cancergenome.nih.gov/) was analysed using Gene Expression Profiling Interactive Analysis (http://gepia.cancer-pku.cn). The correlations between FTO expression and STAT3 expression of bladder cancer in TCGA database was also analysed using Gene Expression Profiling Interactive Analysis. The associations between clinical characteristics of patients with bladder cancer and patient prognosis were analysed using R software according to TCGA database.
Cell transfection and stable cell line construction
T24 and UM-UC-3 cells were plated in six-well dishes and cultured in a 5% CO2 humidified incubator at 37°C. On reaching 40% confluency, T24 and UM-UC-3 cells were transfected with the FTO overexpression lentivirus, negative control, FTO knockdown lentivirus, or scramble control (GeneChem, China). All cells were transfected using Lipofectamine 3000 (Invitrogen, USA). The multiplicity of infection (MOI) of T24 cells and UM-UC-3 cells was 10 and 20 respectively. The infected cells were selected by puromycin (2 μg/mL).
T24 and UM-UC-3 cells were plated in six-well dishes and cultured in a 5% CO2 humidified incubator at 37°C. STAT3-carrying plasmid for overexpression and NC were constructed by Promega. STAT3 siRNA and NC siRNA were obtained from GenePharma (Shanghai, China). All cells were transfected using Lipofectamine 3000 (Invitrogen, USA).
All sequences were presented in Supplementary Table 1.
Western blotting
Total proteins were extracted from the cells and tissues using radio immunoprecipitation assay buffer (BIOSS) mixed with phenylmethylsulfonyl fluoride (BIOSS) and quantified using bicinchoninic acid assay (Beyotime Institute of Biotechnology). Protein extractions (30ug per well) were separated using 10% sodium dodecyl sulphate-polyacrylamide gel electrophoresis and transferred onto polyvinylidene fluoride membranes (MilliporeSigma). The membranes were blocked with 5% fat-free dried milk for 1 h at room temperature. After incubation with high-affinity anti-FTO (1:1000, Abcam, USA), anti-STAT3 (1:1000, Cell Signaling Technology, USA), anti- phosphorylation-STAT3 (1:1000, Cell Signaling Technology, USA), anti-Bcl-2 (1:1000, Cell Signaling Technology, USA), anti-c-Myc (1:1000, Abways Technology, China), anti-CyclinD-1 (1:1000, Abways Technology, China), anti-β-actin (1:5 000, Bioss, China), or anti-GAPDH (1:5000, Bioss, China) antibodies at 4°C overnight, the membranes were incubated with the HRP-conjugated secondary antibodies goat anti-rabbit (1:10,000; Boster, China) or goat anti-mouse (1:5000; Boster, China) for 1 h at room temperature. Proteins were detected using BeyoECL chemiluminescence kit (Biyuntian, China) and detected using the Amersham ImageQuant 800 system (Cytiva). The density of bands were measured using ImageJ (v1.51, National Institutes of Health).
Immunohistochemistry (IHC)
All bladder cancer tumour, normal and xenograft tumour tissues were fixed in a 10% formalin solution for 24 h at room temperature, before they were embedded in paraffin and cut into 4-μm-thick sections. They were then dehydrated in an ascending ethanol gradient and cleared with xylene. The slices were treated with 95°C Sodium Citrate Antigen Retrieval Solution (Solarbio, China) for 15 min. The slices were then treated with 3% H2O2 at 37°C for 10 min and blocked in 3% BSA (Solarbio, China) solution for 30 min at room temperature. Immunohistochemical staining were subsequently performed. The sections were incubated with antibodies against FTO (1:200, Abcam, USA) and STAT3 (1:200, Cell Signaling Technology, USA) at 4°°C overnight and then incubated with the HRP-conjugated secondary antibody goat anti-rabbit (1:10,00; Boster, China) for 1 h at room temperature. Finally, the sections were incubated with 3,3’-diaminobenzidine to reveal positive staining and counterstained with Haematoxylin (Solarbio, China) lasting for 2 min at room temperature. Staining images were acquired using a microscope (magnification, x200; ZEISS, Axio Vert.A1, Germany).
RNA extraction and quantitative real-time PCR (qRT-PCR)
We extracted total RNA from tissues and cells using TRIzol reagent (Invitrogen, USA) and then synthesized cDNA from total RNA using Evo M-MLV RT Premix (Accurate Biology, China), according to the manufacturer’s instructions. qRT-PCR was performed in triplicate on a LightCycler 96 instrument (Roche, USA) using Premix Pro Taq HS qPCR Kit (Accurate Biology, China). The relative RNA expression levels were analysed using the 2−ΔΔCT method, with the levels normalized to GAPDH. All PCR primers were obtained from Accurate Biology (China). Supplementary Table 2 listed the all sequences.
Cell growth and proliferation assays
For colony formation assay, the transfected cell lines were seeded into six-well plates (800 cells/well) at 5% CO2 and 37°C. After 1 week, the colonies were fixed with 100% methanol for 30 min at room temperature and stained with 0.1% crystal violet for 30 min at room temperature. Under a microscope (ZEISS, Axio Vert.A1, Germany), colonies containing > 50 cells were considered survivors and counted.
For CCK-8 assay, transfected cells were seeded into 96-well plates at a density of 2,000 cells per well and quantified every 24 h using the Cell Counting kit-8 (BIOSS) according to the manufacturer’s instructions. Briefly, each well was added with 10 ul CCK-8 solution and the plate was incubated at 37°C for 1 h in dark. The CCK-8 assay absorbance was read at 450 nm using a spectrophotometer (Tecan Group, Ltd.).
EdU incorporation assay
EdU assays were performed using an EdU Apollo DNA in vitro kit (Ribobio, China), according to the manufacturer’s instructions. Briefly, the transfected cells were plated in 24-well plates overnight. 30 μM EdU were added into each well at 37°C for 90 min. After fixation with 4% paraformaldehyde and incubation in 0.5% Triton X-100, the cells were stained using Apollo for 30 min.
Lastly, the cells were incubated with DAPI and observed under a fluorescence microscope. The number of EdU-positive cells was measured using image J.
Transwell migration and wound healing assays
Migration assays were performed using an 8.0 Corning™ 24-well Transwell assay plate (Corning, USA). The transfected cell lines were seed into the upper chamber of the 24-well Transwell plates (20000 cells/well). Approximately 700 μL of medium containing 20% FBS was added to the lower chambers. After about 24 h, cells on the lower surface of the membrane were fixed with methanol and stained with crystal violet. Migrating cells were counted under a microscope.
FTO-overexpressing and or knockdown cells were cultured in six-well plates at 37°C in an incubator at 5% CO2. The cells were then wounded by scratching with plastic 200-μl pipette tips. After 24 h of incubation at 37°C with serum-free medium, the wound healing area was observed by microscopy (magnification, x100; ZEISS, Axio Vert.A1, Germany). The scratch area was measured using ImageJ (v1.51, National Institutes of Health) and the migration rate was calculated as (scratch area at 0 h-scratch area at 24 h)/scratch area at 0 h × 100%.
M6A-RNA immunoprecipitation (MeRIP) assay
T24 cells with stable overexpression of FTO were subjected to MeRIP assay using Magna RIP RNA-Binding Protein Immunoprecipitation Kit (Millipore, USA), according to the manufacturer’s instructions. Briefly, RIP lysate was obtained from stable T24 cells using RIP lysis buffer, followed by the immunoprecipitation with anti--N6-methyladenosine (m6a, Cell Signaling Technology, USA), or anti-immunoglobulin G (IgG; Millipore, USA) antibodies which was linked to magnetic bead suspension. Total RNA was purified by TRIzol reagent and detected by RT-qPCR
RNA stability assay
Cells with overexpression and knockdown of FTO were treated with 5ug/ml actinomycin D (Aladdin, A113142) to inhibit transcription. Cells were collected at 0 h, 2 h, 4 h, 6 h. Total RNA was extracted by TRIzol reagent and analysed by qRT-PCR.
Treatment of methylation inhibitors
Bladder cancer cells were cultured in six-well plates. After seeding, cells were treated with the global methylation inhibitor DAA. Total RNA was extracted using TRIzol reagent and measured using qRT-PCR.
Statistical analysis
All data were statistically performed using GraphPad Prism 9 and data were presented as mean ± standard deviation (SD). We analysed comparisons between groups by student’s t-test and assessed overall survival using the Kaplan – Meier method. Univariate Cox analysis and Multivariate Cox analysis were used to detect independent prognostic factors. All experiments were repeated at least for three times, and statistical significance was set at P < 0.05.
Results
FTO was upregulated in bladder cancer and was correlated with patient survival
We detected FTO expression in bladder cancer tissues and adjacent tissues by western blotting. The expression of FTO was markedly increased in tumour tissues compare with adjacent tissues (). IHC also shows the positive rate of FTO was higher in tumour tissues than in normal tissues (). We examined the relationship between FTO and bladder cancer patient prognosis using the Gene Expression Profiling Interactive Analysis (GEPIA) database (http://gepia.cancer-pku.cn/). Kaplan-Meier survival analysis showed that high FTO expression was significant correlated with poorer overall survival in bladder cancer patients (). Furthermore, we analysed the associations between clinical characteristics of the patients and patient prognosis using the RStudio according to TCGA database. Univariate cox regression analysis and multivariate cox regression analysis showed that FTO serves as an independent predictor ().
Figure 1. FTO was upregulated in bladder cancer and was correlated with patient survival. a. Western blotting revealed that FTO expression in bladder cancer (T) and matched adjacent normal (N) tissues. b. FTO expression in bladder cancer (Tumor) and matched adjacent normal (Normal) tissues detected by IHC analysis at 200× magnification. Scale bars indicate 50 μm. c. Kaplan–Meier survival curves of overall survival based on the GEPIA database. d. FTO was considered as an independent predictor through Univariate and multivariate cox regression analysis.
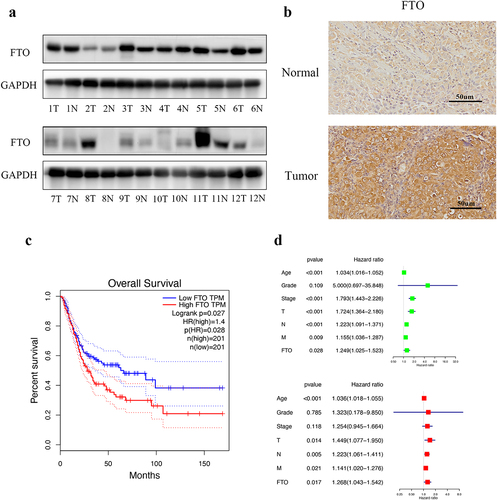
The overexpression of FTO promoted proliferation and migration abilities of bladder cancer
To identity the functions of FTO in T24 and UM-UC-3 cell lines, we used lentiviral transfection to upregulate the FTO expression. Western blotting confirmed the FTO expression (). Overexpression of FTO increased the colony-forming ability via colony formation assays (). CCK-8 and EdU assays revealed that overexpression of FTO significantly promoted the proliferation of bladder cancer cells ().
Figure 2. The overexpression of FTO promoted proliferation and migration abilities of bladder cancer. a. FTO expression was detected by western blotting after FTO overexpression in bladder cancer cells. b. Colony formation assay showed that the colony-forming ability of FTO-overexpressing cells. c and d. the proliferative ability of FTO-overexpressing cells was determined using CCK-8 (C) and EdU (D, magnification × 200, scale bars indicate 50 μm) assays. e Transwell migration assay of FTO-overexpressing cells at 100× magnification. f. Wound healing assays of FTO-overexpressing cells at 100× magnification. Scale bars indicate 100 μm. Scale bars indicate 100 μm. Data are presented as mean ± SD. *P < 0.05, **P < 0.01, and ***P < 0.001.
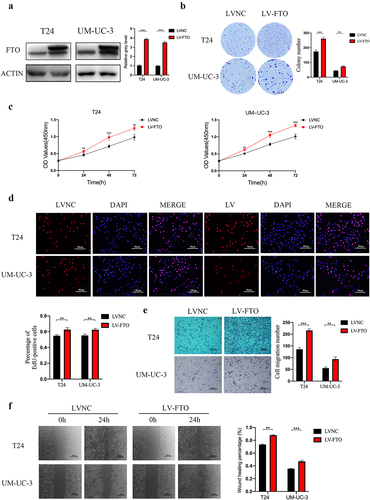
We further examined the role of FTO in migration using transwell assays and wound healing assays. Transwell assays showed that FTO overexpression enhanced the migratory ability of T24 and UM-UC-3 cells (). Wound healing assays also demonstrated that FTO overexpression promoted cancer cells migration ().
FTO knockdown suppressed proliferation and migration abilities of bladder cancer
Stable FTO-knockdown T24 and UM-UC-3 cells were constructed using lentiviral transfection and were confirmed by western blotting (). Colony formation assays revealed that FTO knockdown decreased the colony-forming ability (). The ability of cell proliferation was significantly downregulated in FTO knockdown cells by CCK-8 and EdU assays (). Transwell assays and wound healing assays demonstrated that FTO knockdown decreased the migratory ability of T24 and UM-UC-3 cells ().
Figure 3. FTO knockdown suppressed proliferation and migration abilities of bladder cancer. a. FTO expression was detected by western blotting after FTO knockdown in bladder cancer cells. b. Colony formation assay showed that the colony-forming ability of FTO-knockdown cells. c and d. the proliferative ability of FTO- knockdown cells was determined using CCK-8 (C) and EdU (D, magnification × 200, scale bars indicate 50 μm) assays. e. Transwell migration assay of FTO-knockdown cells at 100× magnification. Scale bars indicate 100 μm. f. Wound healing assays of FTO-knockdown cells at 100× magnification. Scale bars indicate 100 μm. Data are presented as mean ± SD. *P < 0.05, **P < 0.01, and ***P < 0.001.
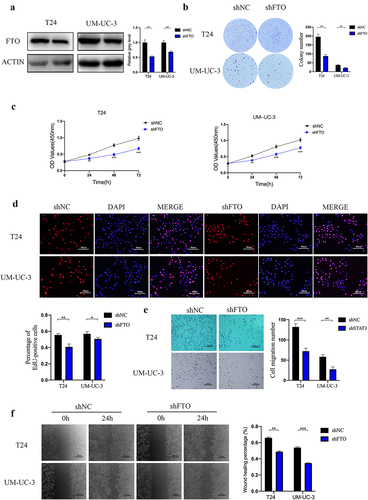
FTO regulated the expression of STAT3 by modulating demethylation-mediated STAT3 mRNA stability
The downregulation of m6A has reported to be related with mRNA stability [Citation16]. We next investigated whether FTO-mediated demethylation could promote bladder cancer progression by activating STAT3 pathway. According to the GEPIA database (http://gepia.cancer-pku.cn/), FTO has a positive correlation with STAT3 (). Western blotting and qRT-PCR revealed that FTO overexpression cell lines showed increased levels of STAT3 () and FTO knockdown decreased the expression of STAT3 (). Additionally, we revealed that FTO overexpression increased the stability of STAT3 mRNA in T24 cells () and FTO knockdown reduced the stability of STAT3 (). Furthermore, down-regulated mRNA expression of STAT3 was markedly rescued by DAA, suggesting the regulatory effect of FTO on STAT3 expression in m6a-dependent manner (). The MeRIP assay testified that FTO overexpression triggered the significant reduction of m6A level in STAT3 mRNA in T24 cells (). In summary, these results show that FTO-mediated demethylation increased STAT3 expression by enhancing mRNA stability.
Figure 4. FTO regulated the expression of STAT3 by modulating demethylation-mediated STAT3 RNA stability. a. Correlation analysis between FTO and STAT3 expression (r = 0.52, P < 0.001) using the GEPIA database. b am=nd c. STAT3 protein and mRNA expression was detected by western blotting (B) and Qrt-PCR (C) after FTO overexpression in T24 and UM-UC-3 cells. d. STAT3 mRNA expression was detected by Qrt-PCR after FTO overexpression and simultaneous treatment with actinomycin D in T24 cells. e and f. STAT3 protein and mRNA expression was detected by western blotting (B) and Qrt-PCR (C) after FTO knockdown in T24 and UM-UC-3 cells. g. STAT3 mRNA expression was detected by Qrt-PCR after FTO knockdown and simultaneous treatment with actinomycin D in T24 cells. h. FTO mRNA expression as determined by Qrt-PCR after FTO knockdown and simultaneous treatment with or without 3-deazaadenosine (3-DAA) in T24 cells. i. m6A-modified mRNA levels in FTO-overexpressing T24 cells were measured using Qrt-PCR. j and k. P-STAT3, Bcl-2, c-Myc and CyclinD-1 protein expression level as determined by western blotting after FTO overexpression and FTO knockdown in T24 and UM-UC-3 cells. Data are presented as mean ± SD. *P < 0.05, **P < 0.01, and ***P < 0.001.
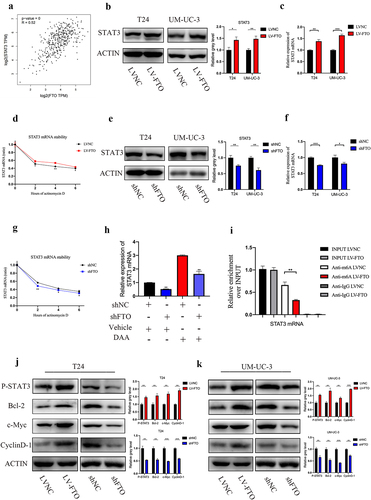
To investigate whether FTO stimulated STAT3 signalling by reducing level of m6A modification in STAT3, we verified phosphorylation status of STAT3 and the expression of downstream targets of STAT3. FTO overexpression increased the phosphorylation levels of STAT3 and expression of Bcl-2, c-Myc and CyclinD-1 in bladder cancer. Phosphorylation levels of STAT3 and expression of Bcl-2, c-Myc and CyclinD-1 decreased with FTO knockdown (). These results suggested that FTO-mediated m6A demethylation activates the STAT3 signalling pathway.
STAT3 served as an oncogene of bladder cancer
To investigate the role of STAT3 in bladder cancer. Colony formation assays showed that the colony-forming ability was increased by upregulating STAT3 expression and was reduced by downregulating STAT3 expression (). CCK-8 assays revealed that STAT3 overexpression enhanced the proliferative ability of bladder cancer cells and STAT3 knockdown inhibited cancer cells proliferation (). Transwell assays showed that STAT3 overexpression promoted bladder cancer cells migration and STAT3 knockdown decreased the ability of migration ().
Figure 5. STAT3 served as an oncogene of bladder cancer. a and b. the colony-forming ability of STAT3 overexpressing (A) and knockdown (B) cells as determined by colony formation assay. c and d. the proliferative ability of STAT3-overexpressing (C) and knockdown (D) cells as determined by CCK-8 assay. e and f. Transwell migration assay of STAT3-overexpressing (E) and knockdown (F) cells at 100× magnification. Scale bars indicate 100 μm. Data are presented as mean ± SD. *P < 0.05, **P < 0.01, and ***P < 0.001.
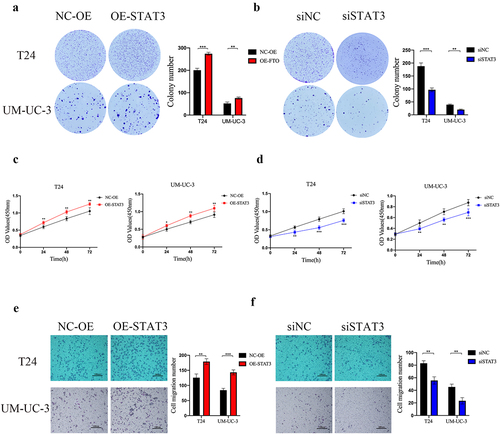
Discussion
Accumulating evidence has confirmed that m6A affects human crucial physiological and pathological processes [Citation17–20]. FTO as the first m6A demethylase, serves an important role in tumour progression [Citation21]. It is reported that FTO is frequently dysregulated in multiple types of cancer [Citation11,Citation22,Citation23]. FTO-mediated demethylation is widely involved in tumour growth, immunotherapy and drug resistance [Citation24–26]. Zhou et al. and Fan et al. have reported that FTO promotes malignant progression of bladder cancer [Citation24,Citation27], which is consistent with our study. Our study revealed that FTO promoted proliferative and migratory capacity of bladder cancer by enhancing the stability of STAT3 mRNA.
The signal transducer and activator of transcription (STAT) proteins are a family of cytoplasmic transcription factors. Among them, as a convergent point of numerous carcinogenic signalling pathways [Citation28–30], STAT3 is required for constitutively activation or maintenance of transformed phenotype in various tumours such as breast cancer, brain tumours, lung cancer, prostate cancer, pancreatic cancer, renal carcinoma, myeloma [Citation31,Citation32]. Abundant evidences show that STAT3 is generally related with poor prognosis and the crucial role of STAT3 in tumour cells proliferation, differentiation, apoptosis, invasion and immune responses [Citation33,Citation34]. Many studies have focused on exploring effective strategies to target the STAT3 signalling pathway, such as directly inhibiting STAT3 protein, inhibiting upstream tyrosine kinases, and DNA binding complexes. However, extensive crosstalk and alternative signalling pathways are identified in STAT3-activated malignancies, including epigenetic mechanisms [Citation35]. Our study shows FTO overexpression reduces methylation level of bladder cancer cells via an m6A-dependent manner and subsequently activates STAT3 signalling pathway.
In summary, we demonstrated that FTO intervention is an effective therapeutic strategy to inhibit STAT3 signalling pathway and we confirmed FTO serves as a potential therapeutic target in bladder cancer.
Supplemental Material
Download Zip (20.5 KB)Acknowledgments
We thank the patients that are involved in this study.
Disclosure statement
No potential conflict of interest was reported by the author(s).
Data availability statement
The data generated in this study are available upon request from the corresponding author.
Supplementary material
Supplemental data for this article can be accessed online at https://doi.org/10.1080/15592294.2023.2242688
Additional information
Funding
References
- Sung H, Ferlay J, Siegel RL, et al. Global cancer statistics 2020: GLOBOCAN estimates of incidence and mortality worldwide for 36 cancers in 185 Countries. CA A Cancer J Clin. 2021;71(3):209–12. doi: 10.3322/caac.21660
- Sylvester RJ, van der Meijden APM, Oosterlinck W, et al. Predicting recurrence and progression in individual patients with stage Ta T1 bladder cancer using EORTC risk tables: a combined analysis of 2596 patients from seven EORTC trials. European Urology. 2006;49(3):466–5. doi: 10.1016/j.eururo.2005.12.031 discussion 475-7.
- Roignant JY, Soller M. M(6)a in mRNA: an ancient mechanism for fine-tuning gene expression. Trends Genet. 2017;33(6):380–390. doi: 10.1016/j.tig.2017.04.003
- Desrosiers R, Friderici K, Rottman F. Identification of methylated nucleosides in messenger RNA from Novikoff hepatoma cells. Proc Natl Acad Sci U S A. 1974;71(10):3971–3975. doi: 10.1073/pnas.71.10.3971
- He PC, He C. M(6) a RNA methylation: from mechanisms to therapeutic potential. Embo J. 2021;40(3):e105977. doi: 10.15252/embj.2020105977
- He L, Li H, Wu A, et al. Functions of N6-methyladenosine and its role in cancer. Mol Cancer. 2019;18(1):176. doi: 10.1186/s12943-019-1109-9
- Liu T, Wei Q, Jin J, et al. The m6A reader YTHDF1 promotes ovarian cancer progression via augmenting EIF3C translation. Nucleic Acids Res. 2020;48(7):3816–3831. doi: 10.1093/nar/gkaa048
- Yang X, Zhang S, He C, et al. METTL14 suppresses proliferation and metastasis of colorectal cancer by down-regulating oncogenic long non-coding RNA XIST. Mol Cancer. 2020;19(1):46. doi: 10.1186/s12943-020-1146-4
- Chen Y, Peng C, Chen J, et al. WTAP facilitates progression of hepatocellular carcinoma via m6A-HuR-dependent epigenetic silencing of ETS1. Mol Cancer. 2019;18(1):127. doi: 10.1186/s12943-019-1053-8
- Ruan DY, Li T, Wang Y-N, et al. FTO downregulation mediated by hypoxia facilitates colorectal cancer metastasis. Oncogene. 2021;40(33):5168–5181. doi: 10.1038/s41388-021-01916-0
- Xu Y, Ye S, Zhang N, et al. The FTO/miR-181b-3p/ARL5B signaling pathway regulates cell migration and invasion in breast cancer. Cancer Commun (Lond). 2020;40(10):484–500. doi: 10.1002/cac2.12075
- Huang J, Sun W, Wang Z, et al. FTO suppresses glycolysis and growth of papillary thyroid cancer via decreasing stability of APOE mRNA in an N6-methyladenosine-dependent manner. J Exp Clin Cancer Res. 2022;41(1):42. doi: 10.1186/s13046-022-02254-z
- Su T, Wang Y-P, Wang X-N, et al. The JAK2/STAT3 pathway is involved in the anti-melanoma effects of brevilin a. Life Sci. 2020;241:117169. doi: 10.1016/j.lfs.2019.117169
- Jin Y, Kim YH, Park JY, et al. Methyllucidone inhibits STAT3 activity by regulating the expression of the protein tyrosine phosphatase MEG2 in DU145 prostate carcinoma cells. Bioorg Med Chem Lett. 2018;28(5):853–857. doi: 10.1016/j.bmcl.2018.02.012
- Zou S, Tong Q, Liu B, et al. Targeting STAT3 in Cancer Immunotherapy. Mol Cancer. 2020;19(1):145. doi: 10.1186/s12943-020-01258-7
- Wang X, Lu Z, Gomez A, et al. N6-methyladenosine-dependent regulation of messenger RNA stability. Nature. 2014;505(7481):117–120. doi: 10.1038/nature12730
- Sun T, Wu R, Ming L. The role of m6A RNA methylation in cancer. Biomed Pharmacother. 2019;112:108613. doi: 10.1016/j.biopha.2019.108613
- Chen X, Hua W, Huang X, et al. Regulatory role of RNA n(6)-methyladenosine modification in bone biology and osteoporosis. Front Endocrinol. 2019;10:911. doi: 10.3389/fendo.2019.00911
- Sun D, Yang H, Fan L, et al. M6a regulator-mediated RNA methylation modification patterns and immune microenvironment infiltration characterization in severe asthma. J Cell Mol Med. 2021;25(21):10236–10247. doi: 10.1111/jcmm.16961
- Wang J, Wang K, Liu W, et al. M6a mRNA methylation regulates the development of gestational diabetes mellitus in Han Chinese women. Genomics. 2021;113(3):1048–1056. doi: 10.1016/j.ygeno.2021.02.016
- Chen J, Du B. Novel positioning from obesity to cancer: FTO, an m(6)A RNA demethylase, regulates tumour progression. J Cancer Res Clin Oncol. 2019;145(1):19–29. doi: 10.1007/s00432-018-2796-0
- Relier S, Ripoll J, Guillorit H, et al. FTO-mediated cytoplasmic m(6)Am demethylation adjusts stem-like properties in colorectal cancer cell. Nat Commun. 2021;12(1):1716. doi: 10.1038/s41467-021-21758-4
- Huang H, Wang Y, Kandpal M, et al. FTO-Dependent N 6 -methyladenosine modifications inhibit ovarian cancer stem cell self-renewal by blocking camp signaling. Cancer Res. 2020;80(16):3200–3214. doi: 10.1158/0008-5472.CAN-19-4044
- Zhou G, Yan K, Liu J, et al. FTO promotes tumour proliferation in bladder cancer via the FTO/miR-576/CDK6 axis in an m6A-dependent manner. Cell Death Discov. 2021;7(1):329. doi: 10.1038/s41420-021-00724-5
- Yang S, Wei J, Cui Y-H, et al. M(6)a mRNA demethylase FTO regulates melanoma tumorigenicity and response to anti-PD-1 blockade. Nat Commun. 2019;10(1):2782. doi: 10.1038/s41467-019-10669-0
- Wang C, Li L, Li M, et al. FTO promotes Bortezomib resistance via m6A-dependent destabilization of SOD2 expression in multiple myeloma. Cancer Gene Ther. 2023;30(4):622–628. doi: 10.1038/s41417-022-00429-6
- Tao L, Mu X, Chen H, et al. FTO modifies the m6A level of MALAT and promotes bladder cancer progression. Clin Transl Med. 2021;11(2):e310. doi: 10.1002/ctm2.310
- Zhang X, Sai B, Wang F, et al. Hypoxic BMSC-derived exosomal miRnas promote metastasis of lung cancer cells via STAT3-induced EMT. Mol Cancer. 2019;18(1):40. doi: 10.1186/s12943-019-0959-5
- Wang B, Liu T, Wu J-C, et al. STAT3 aggravates TGF-β1-induced hepatic epithelial-to-mesenchymal transition and migration. Biomed Pharmacother. 2018;98:214–221. doi: 10.1016/j.biopha.2017.12.035
- Li YL, Wu L-W, Zeng L-H, et al. ApoC1 promotes the metastasis of clear cell renal cell carcinoma via activation of STAT3. Oncogene. 2020;39(39):6203–6217. doi: 10.1038/s41388-020-01428-3
- Narimatsu M, Maeda H, Itoh S, et al. Tissue-specific autoregulation of the stat3 gene and its role in interleukin-6-induced survival signals in T cells. Mol Cell Biol. 2001;21(19):6615–6625. doi: 10.1128/MCB.21.19.6615-6625.2001
- Yang J, Chatterjee-Kishore M, Staugaitis SM, et al. Novel roles of unphosphorylated STAT3 inoncogenesis and transcriptional regulation. Cancer Res. 2005;65(3):939–947. doi: 10.1158/0008-5472.939.65.3
- Lee H, Jeong AJ, Ye SK. Highlighted STAT3 as a potential drug target for cancer therapy. BMB Rep. 2019;52(7):415–423. doi: 10.5483/BMBRep.2019.52.7.152
- Yu H, Lee H, Herrmann A, et al. Revisiting STAT3 signalling in cancer: new and unexpected biological functions. Nat Rev Cancer. 2014;14(11):736–746. doi: 10.1038/nrc3818
- Wong ALA, Hirpara JL, Pervaiz S, et al. Do STAT3 inhibitors have potential in the future for cancer therapy? Expert Opin Investig Drugs. 2017;26(8):883–887. doi: 10.1080/13543784.2017.1351941