ABSTRACT
Sepsis is the primary cause of acute kidney injury (AKI) and is associated with high mortality rates. Growing evidence suggests that noncoding RNAs are vitally involved in kidney illnesses, whereas the role of circular RNAs (circRNAs) in sepsis-induced AKI (SAKI) remains largely unknown. In this present study, caecal ligation and puncture (CLP) in mice was performed to establish an SAKI model. The expression of circRNAs and mRNAs was analysed using circRNA microarray or next-generation sequencing. The results revealed that the expressions of 197 circRNAs and 2509 mRNAs were dysregulated. Validation of the selected circRNAs was performed by qRT-PCR. Bioinformatics analyses and chromatin immunoprecipitation demonstrated that NF-κB/p65 signalling induced the upregulation of circC3, circZbtb16, and circFkbp5 and their linear counterparts by p65 transcription in mouse tubular epithelial cells (mTECs). Furthermore, competitive endogenous RNA (ceRNA) networks demonstrated that some components of NF-κB signalling were potential targets of these dysregulated circRNAs. Among them, Tnf-α was increased by circFkbp5 through the downregulation of miR-760-3p in lipopolysaccharide (LPS)-stimulated mTECs. Knocking down circFkbp5 inhibited the p65 phosphorylation and apoptosis in injured mTECs. These findings suggest that the selected circRNAs and the related ceRNA networks provide new knowledge into the fundamental mechanism of SAKI and circFkbp5/miR-760-3p/Tnf-α axis might be therapeutic targets.
Introduction
Acute kidney injury (AKI) is a major public health problem featuring fast decrease of renal function and even acute renal failure [Citation1]. The driving causes of AKI comprise sepsis, nephrotoxins, and renal ischaemia-reperfusion injury (IRI) [Citation2]. The kidney is the most commonly injured organ, and the injury is induced by a dysregulated host response to infection during sepsis [Citation3,Citation4]. Sepsis accounts for over 50% of AKI cases, and 60% of patients hospitalized with sepsis develop AKI [Citation5,Citation6]. Sepsis-induced AKI (SAKI) is associated with high morbidity and mortality rates [Citation7]. Although endeavours have been committed to investigating the pathogenesis of SAKI, its mechanisms remain unclear, with issues regarding the lack of effective and specific therapies [Citation3,Citation4].
Although the pathogenesis of SAKI is complicated, renal tubular damage, characterized by inflammation and cell apoptosis, has gained wide attention [Citation8]. Upon sepsis, the inflammatory responses directly lead to tubular epithelial cell (TEC) injury [Citation9]. Moreover, damaged TECs can regulate and trigger intrarenal inflammation, amplifying the renal inflammatory response and causing more TECs apoptosis, thus forming a vicious circle that promotes SAKI progression [Citation8,Citation9]. NF-κB signalling plays a critical role in the inflammatory response and apoptosis of TECs during SAKI [Citation10]. In classical NF-κB signalling, the circulated pathogen-associated molecular patterns (PAMPs), including endotoxin lipopolysaccharide (LPS), activate the toll-like receptors on TECs followed by the degradation of IκBα. Therefore, p65 and p50, the dimers of NF-κB, translocate into the nucleus to stimulate the transcription of inflammatory genes such as Tnf-α, Il-1β, and Mcp-1 [Citation11]. These secretory cytokines aggravate the inflammatory response and enhance the apoptosis of TECs by binding the receptors of cell membranes, which induce SAKI [Citation6].
Circular RNAs (circRNAs) are a new cluster of noncoding RNAs produced by back-splicing an individual precursor mRNA (pre-mRNA) [Citation12,Citation13]. These emerging covalently closed circRNAs have recently been regarded as plenteous, stable, and conserved among different species and being differentially expressed across different cell types, organs, and disease stages [Citation14,Citation15]. Increasing evidence has demonstrated that circRNAs have multiple biological functions. For instance, circRNAs are broadly involved in modulating gene expression as microRNA (miRNA) sponges [Citation16], serving as protein-protein interaction components [Citation17], and regulating transcription and splicing [Citation18]. Furthermore, some circRNAs can be translated into functional proteins [Citation19,Citation20]. To date, limited evidence has correlated circRNAs with the injury of TECs in SAKI [Citation21] however, the global profiles of circRNAs in kidneys with SAKI are lacking. In this study, we adopted the caecal ligation and puncture (CLP) model, the mouse model closest to the clinical symptoms of SAKI [Citation8,Citation22], to profile circRNA expression patterns in the renal cortex of SAKI mice. Dysregulated circRNAs were validated and analysed using bioinformatics tool. For a better understanding of the molecular mechanism of these dysregulated circRNAs and the potential circRNAs-mRNAs regulation models, transcriptomic analysis was also performed. The results indicated that NF-κB signalling was highly activated in the kidneys of SAKI mice. Some studies have reported that dysregulated circRNAs induce the activation of NF-κB signalling in vascular smooth muscle cells and nasopharyngeal carcinoma [Citation23,Citation24]. However, whether NF-κB signalling leads to aberrant expression of circRNAs in TECs is unclear. We found that some circRNAs were upregulated owing to the transcriptional regulation of p65 in injured TECs, which may help explain the molecular mechanisms underlying the upregulation of circRNAs.
In the current study, we utilized high-throughput circRNA microarray and transcriptome sequencing to determine alterations in circRNA and mRNA expression profiles in the renal cortex of CLP mice. Gene ontology (GO) and Kyoto Encyclopedia of Genes and Genomes (KEGG) pathway enrichment analyses were performed to predict the biological functions in which dysregulated circRNAs or mRNAs were involved in SAKI. Further mechanisms of the circRNA-miRNA-mRNA axis were explored using bioinformatics tools. The pathogenic effect of circFkbp5/miR-760-3p/Tnf-α on NF-κB signalling activation and apoptosis of TECs was further explored.
Materials and methods
Animal models of SAKI
The in vivo experiments in this research conformed to a protocol which was reviewed and approved by Animal Care and Use Ethics Committee of Southern Medical University (Guangzhou, China). Experimental animal (C57BL/6, male, 7–8 weeks old, 20–22 g) were sold by the Animal Laboratory Center of Southern Medical University. For the evaluation of the renal function, renal pathology and dysregulated molecular biological indicators in CLP mice, 18 mice were randomly divided into three groups: normal, sham, and CLP groups (n = 6 in each group). For the high-throughput circRNA array and RNA sequencing, renal cortex samples from above-mentioned mice in sham and CLP groups were detected (n = 3 in each group). The mouse SAKI model was induced by the classic CLP treatment as previously described [Citation25]. Briefly, after a midline incision, a segment (15 mm) from the bottom tip of the caecum was located and ligated with 4–0 silk and then punctured through with a 21-g needle. The caecum of mouse in sham group was located without ligation. The mice in normal group were anaesthetised without other treatment. After the operation, all mice received fluid resuscitation with normal saline (50 ml/kg) by subcutaneous injection.
Measurement of serum creatinine
The blood samples from CLP and Sham mice were centrifuged (3000× g, 10 minutes, 4 °C). For kidney function assessment, serum creatinine was evaluated with a creatinine (sarcosine oxidase) assay kit (Jiancheng Bioengineering Institute, Nanjing, China).
Cell cultivation and treatment
The immortalized human tubular epithelial cell line HK2 (ATCC, Manassas, VA, USA) was commercially available. The immortalized mTECs (mouse kidney proximal tubular epithelial cell line) was a gift from prof. Hui-Yao Lan from The Chinese University of Hong Kong. Cells were cultured in DMEM/F12 (Invitrogen) supplemented with 10% FBS (Invitrogen) at 37 °C in humidified 5% CO2. LPS (10 μg/mL, Sigma Aldrich) was used to stimulate TECs to mimic a cellular sepsis model. For depletion of p65 or circFkbp5, mTECs were transfected with siRNAs targeting p65 mRNA or circFkbp5 (Genepharma, Suzhou, China) with Opti-MEM and Lipofectamine 2000 (Invitrogen) under the guidance of the manufacturer’s instructions. JSH-23 (10 μM, Selleck) or sc-3060 (10 μM, Santa Cruz), two inhibitors to block NF-κB nuclear translocation were separately used to treat cells 12 h before LPS stimulation.
CircRNA microarray
Total RNAs were extracted from three pairs of renal cortex tissues of CLP and Sham mice using TRIzol (Invitrogen). Treatment and molecular hybridization of the total RNAs were conducted according to the Arraystar’s standard protocols. In brief, total RNA was digested by RNAse R (Epicentre, Madison, Wisconsin) to erasure linear RNAs and enrich circular RNAs. The enriched circular RNAs were then amplified and transcribed into fluorescent cRNA using a random priming method (Arraystar Super RNA Labelling Kit; Aksomics, Shanghai, China). These fluorescent cRNAs were hybridized onto the Mouse circRNA Array V2 (Arraystar), containing probes targeting 14,236 circRNA. A Scanner (G2505C, Agilent) was applied to capture images of the arrays. Agilent Feature Extraction software (version 11.0.1.1) was used to analyse the obtained array images. Then we used the R software Limma package to perform quantile normalization and subsequent data. Expression changes of circRNAs (≥ 2-fold) in SAKI mice were screened out and identified by Volcano Plot filtering.
Transcriptomic analysis and functional enrichment analysis
High-throughput sequencing was performed by Seqhealth Technology (Wuhan, China). Briefly, total RNAs from the renal cortex sample were extracted using the TRIzol reagent (Thermo Fisher Scientific, MA, USA). The quantity and quality of RNAs were examined using a NanoDrop spectrophotometer (Thermo Fisher Scientific). The RNA integrity was examined by 1.5% agarose gel electrophoresis and quantified with a QubitTM RNA Broad Range Assay kit (Q10210, Life Technologies). Then, a sequencing library was constructed with a kit (DR08402, Seqhealth Technology) using total RNAs (2 μg) as templates. After checking using Trimmomatic (version 0.36), low-quality reads of the original data were dumped. The processed data were parallel to the mouse reference genome (GRCm38/mm10) using the STRA software version 2.5.3a with default parameters.
Bioinformatics analysis
Gene Ontology (GO) analysis and Kyoto Encyclopaedia of Genes and Genomes (KEGG) pathway analyses were performed with R toolset. In particular, the potential functions and KEGG analysis of the dysregulated circRNAs were predicted according to the host genes.
Immunoblotting analysis
Kidney tissues and cultured cells were lysed in RIPA buffer (Beyotime) supplemented with inhibitors for protease and phosphatase (Thermo Fisher Scientific). BCA protein quantitation kit (Thermo Fisher Scientific) was used to measure protein concentration.
Immunoblotting assays were performed as described previously [Citation26]. The commercially available antibodies applied are as follows: anti-phospho-p65 (Ser) (#3033, Cell Signalling Technology), anti-p65(#8242, Cell Signalling Technology), anti-Tnf-α (#3707, Cell Signalling Technology) and anti-β-actin (#3700, Cell Signalling Technology). Image J software was used to analyse Western blot images quantitatively.
Mimics and inhibitor of miR-760-3p
The specific mimics and inhibitor were synthesized by Genepharma (Suzhou, China). Based on the manufacturer’s specification, the miR-760-3p mimics or inhibitors were transfected using Lipofectamine 2000 (Invitrogen).
Luciferase reporter assays
For verification of the miR-760-3p binding ability to circFkbp5 and TNF-α. The sequences of circFkbp5, TNF-α-3’UTR and their miR-760-3p binding sites mutants were synthesized and cloned to psiCHECK2 vector (Hanbio Biotechnology, Wuhan, China), respectively named as circFkbp5-wt, circFkbp5-Mut, TNF-α-wt, TNF-α-Mut. The laboratory protocol was guided by the manufacturer’s instructions of the Dual-Luciferase Reporter Assay kit (Promega).
Cell apoptosis assays
Cell apoptosis assays were performed using the FITC Annexin V staining Kit II (BD Biosciences, Franklin Lakes, NJ, USA). The samples were analysed with BD FACS Canto™ II software.
RNA extraction and real-time quantitative RT-PCR (qRT-PCR)
Total RNAs of samples were extracted with the TRIzol reagent. The purity and integrity of the RNA samples were tested with Nanodrop (Thermo Fisher Scientific). For the detection of the expression levels of mRNA and circRNA, reverse transcription (RT)-PCR was conducted using the HiScript® II 1st Strand cDNA Synthesis Kit (Vazyme, R211), then qRT-PCR experiment was performed with ChamQ Universal SYBR qPCR Master Mix (Vazyme, Q711). The expression levels of miRNA were detected with A Mir-X miRNA First-Strand Synthesis and TB Green qRT-PCR Kit (Takara Bio, Japan) in accordance with the manufacturer’s instructions. U6 was applied as the internal control. For relative miRNA expression, miRNA expression was calculated as 2−ΔCT (ΔCT=CTmiR-CTU6). The specific primer pairs for RT-PCR are listed in Supplementary Table S8.
Periodic acid-schiff (PAS) staining
PAS staining was accomplished using a PAS staining kit (Solarbio, Beijing, China) to assess the severity of SAKI. The degree of renal tubular injury in mouse kidney sections was evaluated to determine the acute tubular injury. The tubular injury was defined and evaluated as previously described [Citation27].
TUNEL assay
In order to perform terminal deoxynucleotidyl transferase dUTP nick end labelling (TUNEL), a commercially available kit was used as instructed by the manufacturer (G7130, Promega). To assess the TUNEL+ TECs in pathological sections, each kidney sample was evaluated for the percentage of TUNEL+ TECs in the total quantity of TECs according to 10 randomly selected fields (400×).
ChIP assay
Chromatin immunoprecipitation (ChIP) analysis was carried out as described previously [Citation27]. Briefly, mTECs were treated by 10 μg/mL LPS (Sigma Aldrich) for 3 h. Then, 1% formaldehyde was added to the culture dish for cross-linked reaction at 37°C for 10 minutes, followed by the neutralization reaction with the addition of glycine, then the lysates were collected and sonicated to fracture the DNA into 250- to 600-bp fragments. Antibodies against p65 were reacted equally with DNA from different samples. A normal Rabbit IgG (Cell Signaling Technology) was used to prepare the negative control sample. The precipitated DNA fragments were used to amplify the NF-κB binding sequences with specific primers through qRT-PCR. The specific primer pairs are listed in Supplementary Table S8.
Construction of circRNA-miRNA-mRNA ceRNA regulatory network
As important components of the competitive endogenous RNA (ceRNA) network, circRNAs adversely regulate miRNA expression. First, the miRWalk and miRDB databases were used to identify miRNA-mRNA pairs. Next, circRNA/miRNA interactions were predicted using Arraystar’s homemade toolset based on TargetScan and Miranda. Finally, the ceRNA regulatory network was visualized using Cytoscape software (https://cytoscape.org/).
Statistical analyses
Means and standard deviations (means ± SDs) are used to express continuous variables. The Kolmogorov – Smirnov test and variance homogeneity with Levene’s test were used to test the normality of the data. Normally distributed continuous variables were compared using Student’s t tests (two-tailed) or One-way ANOVA with LSD post hoc tests. Statistical significance was set at P < 0.05.
Results
Establishment of SAKI mice by CLP treatment
To induce SAKI in animals, a well-known CLP mouse model was established. Consistent with previous studies [Citation28,Citation29], renal dysfunction was verified by the upregulation of serum creatinine levels in CLP mice (). TEC injury was observed by histopathological analysis 24 h after the CLP operation. The manifestations of the injury included tubular dilation, epithelial flattening, cell sloughing and coagulative necrosis (). Moreover, an increased number of TUNEL+ TECs in the kidneys of CLP mice was observed, indicating that TEC apoptosis occurred during sepsis (). Particularly, we observed no significant differences in renal function and pathology in sham mice, as compared with that in normal mice. Therefore, we used sham mice as controls for CLP in the subsequent experiments.
Figure 1. Renal dysfunction, elevated acute tubular injury score and apoptosis following CLP-induced sepsis. (a) elevation of serum creatinine in mice at 24 h post-CLP treatment. (b&c) Representative images (b) and quantification data (c) of periodic acid-schiff staining in kidneys from mice subjected to CLP at 24 h post-injury. (d&e) Representative images of TUNEL staining showing an increased percentage of TECs apoptosis in the kidneys from CLP mice (d), and the quantification data (e). The data are presented as means ± SDs. One-way ANOVA with LSD post hoc tests were used for the comparisons of 3 groups (n = 6 mice in each group). ***P < 0.001.
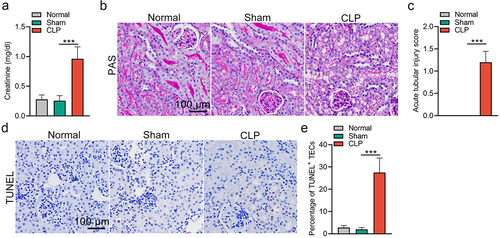
CircRNA expression profiles in the renal cortex of CLP mice
To identify dysregulated circRNAs in CLP mice, high-throughput circRNA microarray assays were performed to detect the expression levels of 14,236 circRNAs in the kidneys of Sham and CLP mice (n = 3 in each group). A Box plot was created to visualize the distribution of intensities from the normalized datasets, confirming that the circRNA expression profiles were almost indistinguishable in the six samples (). We further analysed the size of the identified circRNAs and discovered that the length of most candidates was approximately 250 nucleotides (nt) (). The circRNAs were characterized by identifying their source. Consistent with the findings from a study conducted in a cisplatin-induced AKI mouse model [Citation30], most of the circRNAs in this study were back-spliced products of exons, while lower percentages were transcribed from introns and intergenic sequences (; Supplementary Table S1). Using a volcano plot, we demonstrated the significant differential expression of circRNAs between Sham and CLP mice (). Subsequently, as illustrated in , hierarchical clusters analysis was performed on the differentially expressed circRNAs in the six samples based on their expression levels. As compared to Sham mice, 197 differentially expressed circRNAs (P < 0.05, ≥ 2-fold) in the kidney samples from CLP mice were identified, in which 31 circRNAs were upregulated, and 166 circRNAs were downregulated (; Supplementary Table S1). A list of the top 20 differentially expressed circRNAs is provided in Supplementary Table S2. Except for chromosome Y and the mitochondria genome, the differentially expressed candidates on mouse chromosomes originated from nearly all chromosomes ().
Figure 2. CircRNA expression profiles in the kidney in response to CLP treatment. (a) the distribution of the total expression of the circRNAs in CLP and sham mice. (b) the length distribution of circRNAs was shown in the column chart. (c) the origin of the dysregulated circRNAs in CLP mice. (d) volcano plot showing the differentially expressed circRNAs in CLP mice versus sham mice (n = 3 mice in each group). (e) clustered heatmap of the differentially expressed circRNAs in kidney samples from CLP and sham mice (n = 3 mice in each group). (f) the distribution of up-and down-regulated circRNAs on the chromosome was shown in the column chart. (g) gene ontology analysis on parental genes of the aberrantly expressed circRNAs. The horizontal axis shows the annotated functions of the target genes. The vertical axis shows the number of genes in each cluster. (h) Kyoto Encyclopedia of genes and Genomes analysis on parental genes of the differentially expressed circRNAs.
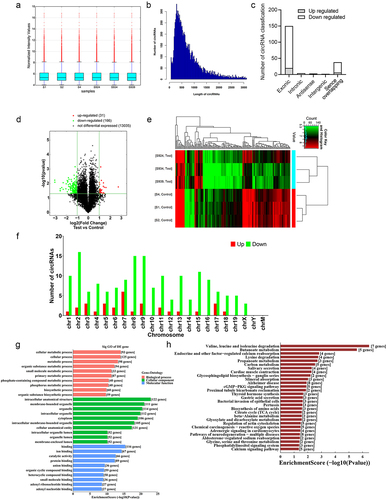
GO and pathway analyses of the parental genes of differentially expressed circRnas
Previous studies suggested that the molecular functions of circRNAs may be consistent with those of their parental genes [Citation31,Citation32]. Therefore, we used the database for annotation, visualization, and integrated discovery (DAVID) [Citation33,Citation34] to investigate the potential functions of the differentially regulated circRNAs. The Gene Ontology (GO) terms are divided into three categories: cellular component (CC), biological process (BP), and molecular function (MF). The results regarding CC showed that the related parental genes were contained in intracellular anatomical structures, membrane-bound organelles, and organelles (; Supplementary Table S3). The results of MF analysis demonstrated that binding, ion binding, and catalytic activity were the most enriched terms (; Supplementary Table S3). For BP, the most enriched term was cellular metabolic processes (; Supplementary Table S3). Furthermore, KEGG pathway analysis [Citation35] revealed 29 pathways that may be correlated with the dysregulated circRNAs in SAKI (; Supplementary Table S4). In KEGG pathway analysis, the most enriched pathways were valine, leucine, and isoleucine degradation (; Supplementary Table S4).
Aberrant activation of NF-κB signaling in the injured TECs of CLP mice
To identify parallel dysregulated mRNAs in the kidneys of Sham and CLP mice, we performed global gene expression profiling using RNA sequencing technology. As compared to Sham mice, 2509 dysregulated mRNAs (P < 0.05, ≥ 2-fold) in the kidney samples from CLP mice were identified, in which 1383 mRNAs were upregulated and 1126 mRNAs were downregulated (). The dysregulated mRNAs are listed in Supplementary Table S5. Pathway analyses demonstrated that inflammation, apoptosis, and NF-κB signalling-related genes were significantly enriched (). Consistently, the upregulation of phosphorylated p65 () and elevated expression of proinflammatory factors () were observed in the renal cortex of CLP mice. These data suggest that NF-κB signalling is highly activated in SAKI, leading to the dysregulated expression of circRNAs.
Figure 3. Aberrant activation of NF-κB signalling in injured TECs from CLP mice. (a) clustered heatmap of the differentially expressed mRNAs in kidney samples from CLP and sham mice (n = 3 mice in each group). (b) the scatter plot showed the differentially expressed mRNAs. (c) volcano plot analysis of the differentially expressed mRNA profiles between CLP mice and sham mice. (d) KEGG pathway analysis showed the top 20 enriched pathways of upregulated mRNAs in the renal cortex from CLP mice, as compared to that from sham mice. (e&f) Western blot analysis for p65 and p-p65 in renal cortex from CLP or sham mice (e), and the quantitative analysis results (f). (g) expression of pro-inflammatory factors in renal cortex from mice subjected to CLP. The data are presented as means ± SDs. A two-tailed Student’s t test was used for the comparison of 2 groups (n = 6 mice in each group in e, f and g). ***P < 0.001.
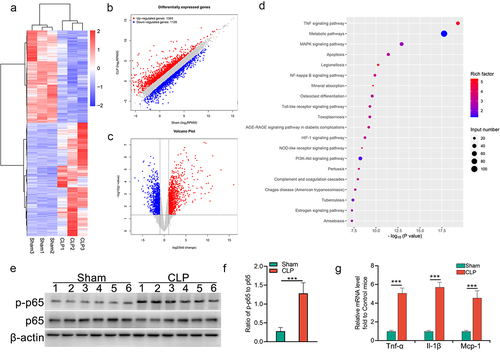
NF-κB/p65 signaling induces circRNAs dysregulation in injured TECs
To verify the circRNA microarray results, we randomly chose three circRNAs that were not only differentially expressed between Sham and CLP mice but were also conserved between humans and mice to promote their clinical translation in future research. Among these three chosen circRNAs, in comparison to sham mice, two circRNAs (circPsen1 and circGrm8) were up-regulated, and one circRNA (circNpr3) was downregulated in CLP mice. To distinguish them from their linearized mRNA transcripts, all circRNAs were amplified using divergent primers (). The qRT-PCR assay demonstrated that, compared to sham mice, the expression levels of circPsen1 and circGrm8 were elevated in CLP mice, whereas circNpr3 was downregulated (). Therefore, the qRT-PCR results were in agreement with the circRNA microarray data ().
Figure 4. NF-κB/p65 signalling induces circRNAs dysregulation in injured TECs. (a) schematic depiction of the amplification process of circRNA from back-splicing with the aid of divergent primers. (b-d) the relative expression levels of circPsen1, circGrm8, and circNpr3 were detected by the qRT-PCR assay in renal cortex from CLP or sham mice. β-actin was used as a normalizing gene for analysis of specific gene expression. (e) the comparison between circRNA microarray and qRT-PCR results. (f-h) the relative expression levels of circPsen, circGrm8, and circNpr3 were assayed by qRT-PCR in HK2 cells induced by LPS stimulation, with or without NF-κB inhibition by JSH-23 or sc-3060. A two-tailed Student’s t test was used for the comparison of 2 groups. One-way ANOVA with LSD post hoc tests were used for the comparisons of 3 groups. n = 6 mice in each group in b, c, d. ***P < 0.001. For f, g, h, i, j, and k, *P < 0.05, **P < 0.01, ***P < 0.001 versus cells treated with PBS, ###P < 0.001 versus LPS-stimulated cells which were pre-treated with DMSO.
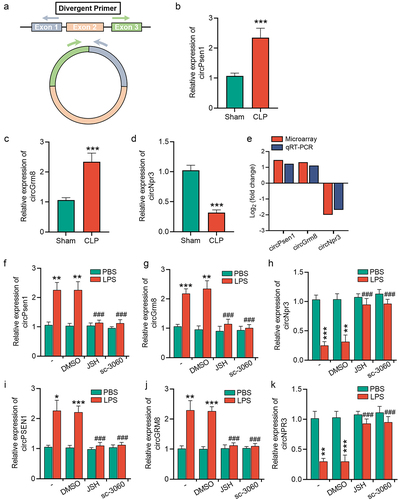
To explore whether the expression levels of these circRNAs were dysregulated upon NF-κB signalling activation, a classical NF-κB activator, LPS, was used to stimulate mTECs. The expression of circPsen1, circGrm8, and circNpr3 was examined in LPS-stimulated mTECs using a qRT-PCR assay, which demonstrated that the changes in these three circRNAs were in accordance with in vivo experiments (). We also investigated whether NF-κB signalling was responsible for the alterations in these three circRNAs. JSH-23 and sc-3060, two inhibitors for NF-κB nuclear translocation, abolished the stimuli-induced upregulation of circPsen1 and circGrm8, whereas they inhibited the stimuli-induced downregulation of circNpr3 (). Similar results were obtained using an immortalized human tubular epithelial cell line (HK2) (), indicating these circRNAs may serve as potential clinical therapeutic targets.
NF-κB/p65 signaling induces upregulation of circRNAs and their linear counterparts by p65 transcription
By a combined analysis of circRNA microarray profiles and transcriptomic sequencing data, we found that four circRNAs, in accordance with linearized mRNAs from their parental genes, were upregulated in the renal cortex of CLP mice, compared with sham mice (). According to a previous study, the expression levels of circRNA, pre-mRNAs, and mRNA from the same host gene can be simultaneously elevated in a transcription factor-dependent manner [Citation36]. Therefore, we investigated whether these four circRNAs and their parental linearized RNAs were upregulated by p65 transcription. Expression of the linear and circular forms of these four genes was examined by qRT-PCR using the corresponding primer sets (). Interestingly, depletion of p65 inhibited the LPS-induced upregulation of pre-mRNA, mature mRNA, and circular forms of the C3, Zbtb16, and Fkbp5 genes in mTECs (). In addition, we found that neither Itgam mRNA nor circItgam were elevated in LPS-stimulated mTECs (), which might be attributed to the fact that Itgam is mainly expressed in immune cells but not in tubular epithelial cells.
Figure 5. NF-κB/p65 signalling induces upregulation of circRNAs and their linear counterparts by p65 transcription. (a) a venn diagram showing the linear and circular transcription product of four genes (C3, Zbtb16, Fkbp5, and Itgam) were upregulated in renal cortex from CLP mice, as revealed by transcriptome sequencing and circRNA microarray. (b) schematic depiction of the amplification process of circRNA, mRNA, and pre-mRNA of each gene. (c-f) qRT-PCR showing the relative expression of the circular and linear transcript products of C3 (c), Zbtb16 (d), Fkbp5 (e), and Itgam (f) in mTECs stimulated with LPS for 24 h. (g-i) ChIP assays showing that p65 bound to the κB-binding site in the promoter regions of C3, Zbtb16, and Fkbp5 under LPS stimulation (10 μg/mL, 3 h) in mTECs. A two-tailed Student’s t test was used for the comparison of 2 groups. One-way ANOVA with LSD post hoc tests were used for the comparisons of 3 groups. n = 3 in each group. *P < 0.05, **P < 0.01, ***P < 0.001.
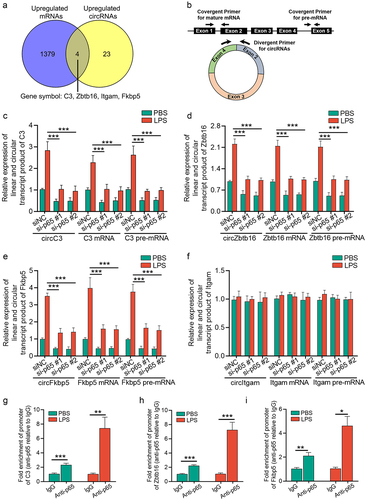
To further confirm that p65 transcribed circC3, circZbtb16, and circFkbp5, we predicted the κB binding sites located at the promoter of their pre-mRNA by JASPAR database [Citation37], which was further confirmed by chromatin immunoprecipitation (ChIP) assay using an anti-p65 antibody ().
Prediction of circRNAs/miRNAs/NF-κB signaling axes in CLP mice
Based on the ceRNA hypothesis [Citation38], circRNAs can sequester miRNAs to elevate the expression of target mRNAs and serve as molecular sponges. Because the NF-κB signalling pathway was one of the most obvious enriched pathways in kidney samples from CLP mice (). To construct the potential circRNAs/miRNAs/NF-κB signalling network in SAKI, firstly, we selected 21 NF-κB pathway-related genes upregulated in kidney samples from CLP mice using mRNA sequencing analysis (Supplementary Table S6). Secondly, we predicted the miRNAs that bind to these 21 genes by considering the intersection of the miRNAs presented in both the miRWalk [Citation38] and miRDB databases [Citation39]. Thirdly, to identify circRNAs with miRNA response elements, the candidate miRNAs in step two were further analysed using a Arraystar- invented miRNA software [Citation40], according to both TargetScan and Miranda (Supplementary Table S1). Finally, the interactions among ten circRNAs, ten miRNAs, and six NF-κB signalling-related genes are shown in (Supplementary Table S7).
Figure 6. Prediction of circRNAs/miRNAs/NF-κB signalling axes in CLP mice. the network includes ten circRNAs, ten miRNAs, and six NF-κB signalling-associated mRNAs, which were constructed and visualized by cytoscape software. The ovals represented circRNAs, the rhombuses represented miRNAs and the hexagons represented mRNAs. The connections among circFkbp5/miR-760-3p/Tnf-α axis and circElmod2/miR-23a-3p/Tnfaip3 axis were specific, which were marked with a dotted line.
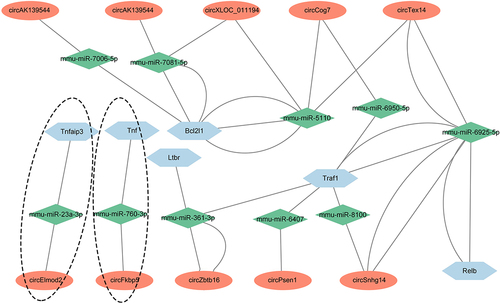
CircFkbp5, a downstream target of NF-κB signaling, promotes TECs apoptosis via sponging miR-760-3p
Based on the ceRNA network constructed above, the connections among circFkbp5/miR-760-3p/Tnf-α axis and circElmod2/miR-23a-3p/Tnfaip3 axis were specific. Moreover, since circFkbp5 was a downstream target of NF-κB signalling (), we investigated if circFkbp5/miR-760-3p/Tnf-α axis regulates NF-κB signalling via a positive feedback loop. The expression level of miR-760-3p was reduced (). In contrast, the mRNA levels of Tnf-α were increased in LPS-stimulated mTECs (). Consistently, knocking down circFkbp5 promoted miR-760-3p expression and decreased Tnf-α expression (). To further validate the association between circFkbp5 and miR-760-3p or the association between Tnf-α and miR-760-3p, we constructed various luciferase reporters containing the 200–500-nt sequence of circFkbp5 or 1113–1413-nt sequence of Tnf-α, which included wild-type (wt) or mutated miR-760-3p binding sequences (). Reporter plasmids were transfected with miR-760-3p mimics into the mTECs. The results demonstrated that miR-760-3p mimics reduced the reporter activity of the construct with circFkbp5-wt sequence or Tnf-α-wt seuqence (). Furthermore, the expression of Tnf-α and the phosphorylation of p65 were inhibited by silencing RNAs (siRNAs) targeting circFkbp5, which was reversed by the miR-760-3p inhibitor in LPS-stimulated mTECs (). Tnf-α is a pro-apoptotic factor of TECs during AKI [Citation41]. Therefore, we tested the role of circFkbp5/miR-760-3p/Tnf-α axis in LPS-induced apoptosis of mTECs. We found that circFkbp5 knockdown reduced LPS-induced mTECs apoptosis, which was reversed by the miR-760-3p inhibitor ().
Figure 7. CircFkbp5, a downstream target of NF-κB signalling, promotes TECs apoptosis via sponging miR-760-3p. (a&b) qRT-PCR showing the relative expression of miR-760-3p and Tnf-α mRNA. (c) schematic illustration of circFkbp5-wt, Tnf-α-wt, circFkbp5-mut, and Tnf-α-mut luciferase reporter vectors. (d&e) luciferase assays in mTECs co-transfected with WT or mutated luciferase reporter vectors of circFkbp5 (d) and Tnf-α (e), together with different doses of miR-760-3p mimics. (f&d) Western blot analysis for p65 and p-p65 (f) or Tnf-α (g) in LPS-stimulated-mTECs transfected with si-circFkbp5 or miR-760-3p inhibitor. (h&i) the effects of si-circFkbp5 or miR-760-3p inhibitor on apoptosis in LPS-stimulated-mTECs (h), and the quantification data (i). A two-tailed Student’s t test was used for the comparison of 2 groups. One-way ANOVA with LSD post hoc tests were used for the comparisons of 3 groups. n = 3 in each group. **P < 0.01, ***P < 0.001. n.s. indicates not significant.
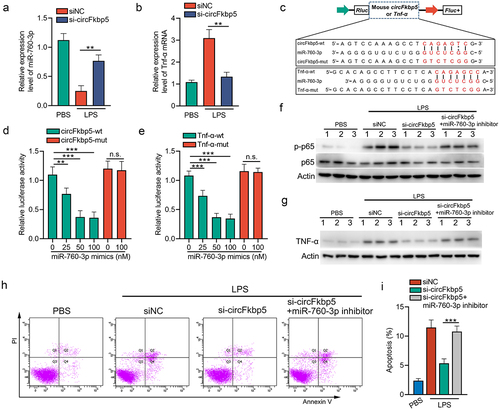
Discussion
SAKI is a global health threat that can cause shock and even death [Citation5]. Hence, elucidating the underlying mechanism of SAKI and screening for potential therapeutic targets is significant. Our study revealed that the expression profiles of circRNAs and mRNAs in control and sepsis-induced kidneys were distinct. A total of 197 dysregulated circRNAs and 2509 dysregulated mRNAs were identified in the SAKI mice. Additionally, we performed DAVID analysis to identify the significantly enriched GO terms and pathways associated with these dysregulated circRNAs or mRNAs. We validated three dysregulated circRNAs using qRT-PCR and ChIP analysis and found that circC3, circZbtb16, circFkbp5, and their linear counterparts are downstream targets of p65. Bioinformatics tools were used to construct the circRNA/miRNA regulatory networks. Among these networks, we found that circFkbp5/miR-760-3p/Tnf-α promotes the apoptosis of injured TECs through activating NF-κB signalling. As shown by these results, dysregulated circRNAs may be involved in the pathogenesis of SAKI and consequently become promising drug targets.
A minority (approximately 2%) of the mammalian transcriptome translates into proteins; in other words, most transcripts cannot. Therefore, they are termed noncoding RNAs (ncRNAs) [Citation42]. Although many ncRNAs play diverse regulatory roles, such as gene expression modulation, transcription regulation, and post-translational modifications, their specific capacities remain to be elucidated. Previous studies have demonstrated that circRNAs are generated by back-splicing the same parent genes as the mRNAs [Citation19]. CircRNAs have attracted increasing attention owing to their abundant expression, stability, and high conservation among humans and rodents [Citation43]. CircRNAs may be involved in fine-tuning renal function in response to CLP. However, their roles remain to be explored. Recent evidence has indicated that circRNAs modulate the functions of parental genes to regulate the pathophysiological processes of various diseases [Citation31,Citation32]. Consequently, dysregulated circRNAs may play a significant role in SAKI at the cellular and molecular levels.
The discovery of potential therapeutic targets for SAKI is difficult because of the lack of well-recognized animal models. LPS-induced systemic inflammation and CLP-induced polymicrobial infection models are widely used to study the pathogenesis of SAKI [Citation44]. In the CLP model, a small amount of normal saline, administered once to replace postsurgical losses, promoted a hyperdynamic phase that mimicked early human sepsis. However, in the typical LPS model, septic mice do not exhibit this hyperdynamic phase [Citation44,Citation45]. Therefore, we chose the CLP-induced sepsis model to investigate potential therapeutic circRNAs. By the way, the CLP-induced sepsis model, but not the LPS-induced SAKI model, was widely performed as the animal model to simulate clinical SAKI [Citation46,Citation47]. According to our findings, host genes associated with differentially expressed circRNAs were mainly enriched regarding cellular metabolic processes, cellular processes, and metabolic processes. More compelling evidence is required to elucidate the contribution of these circRNAs in SAKI.
Previous studies have shown that circRNAs contribute to the pathogenesis of AKI. Moreover, previous studies have reported circular RNA expression profiles in cisplatin-induced AKI, IRI-AKI [Citation30,Citation48–50], except SAKI. However, the pathogenic mechanisms underlying SAKI differ from those underlying cisplatin-induced AKI or IRI-AKI. AKI most commonly occurs in critically ill patients with sepsis. AKI is commonly caused by sepsis in critically ill patients. However, the pathophysiological mechanisms underlying SAKI remain clear. Therefore, identifying potential therapeutic targets in the circRNA layer is crucial.
The primary pathogenetic mechanism of SAKI is renal tubular damage instead of glomerular injury [Citation51]. Recent studies have reported unnatural autophagy [Citation28], excessive apoptosis [Citation8], mitochondrial dysfunction [Citation52], imbalanced inflammation [Citation9], and an adaptive response of TECs to the pathogenic mechanism of SAKI [Citation53], highlighting the role of TECs in SAKI. Therefore, the current study mainly focuses on TECs. According to unbiased transcriptome sequencing, NF-κB signalling was enriched in the renal cortex of CLP mice, which was verified by increased phosphorylation of p65. Therefore, our results and those of other researchers have demonstrated the pathogenic role of NF-κB signalling in injured TECs during SAKI [Citation9]. NF-κB signalling inhibitors were used to explore the regulatory role of NF-κB signalling in dysregulated circRNA expression. The results indicated that the inhibitors reversed the disordered expression of circPsen1, circGrm8, and circNpr3. Interestingly, when we examined the intersection of the upregulated circRNAs and mRNAs, we found that both the circular and linear forms of C3, Zbtb16, Fkbp5, and Itgam were upregulated. p65 knockdown decreased the circular and linear products of C3, Zbtb16, and Fkbp5 in LPS-stimulated mTECs, indicating that p65 directly upregulated their expression. Occupation of p65 at the promoter regions of C3, Zbtb16, and Fkbp5 in mTECs was confirmed using ChIP. Based on what we know now, this is the first study showing NF-κB signalling upregulates the expression of circRNAs by p65 transcript regulation. Finally, the ceRNA networks of dysregulated circRNAs and NF-κB signalling components were predicted, which showed that ten upregulated circRNAs might increase the expression of six NF-κB signalling-related genes by sponging ten miRNAs. Within this network, we found that circFkbp5, a downstream target of NF-κB signalling, promoted the expression of Tnf-α and the apoptosis of LPS-stimulated TECs via sponging miR-760-3p.
In conclusion, the present study identified, for the first time, comprehensive circRNA expression profiles in the renal cortex of SAKI mice compared to sham mice. The potential functional roles of dysregulated circRNAs in the pathophysiology of SAKI and circRNA/miRNA/NF-κB signalling ceRNA networks were determined. These findings suggest novel therapeutic targets for SAKI.
Author contributions
T.M. and Z.C. contributed to study concept and design, and manuscript writing. T.M. and J.W. contributed to the experiments. T.M. contributed to data analysis. Z.C. contributed to the manuscript review.
Supplemental Material
Download Zip (1.4 MB)Acknowledgments
We thank Professor H.-Y. Lan for providing the mTECs cell line for this study.
Disclosure statement
No potential conflict of interest was reported by the author(s).
Data availability statement
RNA-seq and microarray data were deposited in GEO (GSE220812 and GSE220782).
Supplementary material
Supplemental data for this article can be accessed online at https://doi.org/10.1080/15592294.2023.2278960.
Additional information
Funding
References
- Guo C, Dong G, Liang X, et al. Epigenetic regulation in AKI and kidney repair: mechanisms and therapeutic implications. Nat Rev Nephrol. 2019;15:220–17. doi: 10.1038/s41581-018-0103-6
- Ronco C, Bellomo R, Kellum JA. Acute kidney injury. Lancet. 2019;394(10212):1949–1964. doi: 10.1016/S0140-6736(19)32563-2
- Peerapornratana S, Manrique-Caballero CL, Gómez H, et al. Acute kidney injury from sepsis: current concepts, epidemiology, pathophysiology, prevention and treatment. Kidney Int. 2019;96:1083–1099. doi: 10.1016/j.kint.2019.05.026
- Fani F, Regolisti G, Delsante M, et al. Recent advances in the pathogenetic mechanisms of sepsis-associated acute kidney injury. J Nephrol. 2018;31(3):351–359. doi: 10.1007/s40620-017-0452-4
- Poston JT, Koyner JL. Sepsis associated acute kidney injury. BMJ. 2019;364:k4891. doi: 10.1136/bmj.k4891
- Emlet DR, Shaw AD, Kellum JA. Sepsis-associated AKI: epithelial cell dysfunction. Semin Nephrol. 2015;35:85–95. doi: 10.1016/j.semnephrol.2015.01.009
- Gómez H, Kellum JA. Sepsis-induced acute kidney injury. Curr Opin Crit Care. 2016;22:546–553. doi: 10.1097/mcc.0000000000000356
- Wang B, Xu J, Ren Q, et al. Fatty acid-binding protein 4 is a therapeutic target for septic acute kidney injury by regulating inflammatory response and cell apoptosis. Cell Death Dis. 2022;13:333. doi: 10.1038/s41419-022-04794-w
- Liu Z, Tang C, He L, et al. The negative feedback loop of NF-κB/miR-376b/NFKBIZ in septic acute kidney injury. JCI Insight. 2020;5: doi: 10.1172/jci.insight.142272
- Zhang Y, Song C, Ni W, et al. HSP70 ameliorates septic acute kidney injury via binding with TRAF6 to inhibit of inflammation-Mediated apoptosis. J Inflamm Res. 2022;15:2213–2228. doi: 10.2147/jir.s352717
- Płóciennikowska A, Hromada-Judycka A, Borzęcka K, et al. Co-operation of TLR4 and raft proteins in LPS-induced pro-inflammatory signaling. Cell Mol Life Sci. 2015;72(3):557–581. doi: 10.1007/s00018-014-1762-5
- Memczak S, Jens M, Elefsinioti A, et al. Circular RNAs are a large class of animal RNAs with regulatory potency. Nature. 2013;495:333–338. doi: 10.1038/nature11928
- Ashwal-Fluss R, Meyer M, Pamudurti NR, et al. circRNA biogenesis competes with pre-mRNA splicing. Molecular Cell. 2014;56:55–66. doi: 10.1016/j.molcel.2014.08.019
- Jeck WR, Sorrentino JA, Wang K, et al. Circular RNAs are abundant, conserved, and associated with ALU repeats. RNA. 2013;19:141–157. doi: 10.1261/rna.035667.112
- Salzman J, Chen RE, Olsen MN, et al. Cell-type specific features of circular RNA expression. PLoS Genet. 2013;9(9):e1003777. doi: 10.1371/journal.pgen.1003777
- Sang Y, Chen B, Song X, et al. circRNA_0025202 regulates tamoxifen sensitivity and tumor progression via regulating the miR-182-5p/FOXO3a axis in breast cancer. Mol Ther. 2021;29:3525–3527. doi: 10.1016/j.ymthe.2021.11.002
- Li B, Zhu L, Lu C, et al. circNDUFB2 inhibits non-small cell lung cancer progression via destabilizing IGF2BPs and activating anti-tumor immunity. Nat Commun. 2021;12:295. doi: 10.1038/s41467-020-20527-z
- Ma J, Du WW, Zeng K, et al. An antisense circular RNA circSCRIB enhances cancer progression by suppressing parental gene splicing and translation. Mol Ther. 2021;29:2754–2768. doi: 10.1016/j.ymthe.2021.08.002
- Wang P, Huang Z, Peng Y, et al. Circular RNA circBNC2 inhibits epithelial cell G2-M arrest to prevent fibrotic maladaptive repair. Nat Commun. 2022;13(1):6502. doi: 10.1038/s41467-022-34287-5
- Ouyang X, He Z, Fang H, et al. A protein encoded by circular ZNF609 RNA induces acute kidney injury by activating the AKT/mTOR-autophagy pathway. Mol Ther. 2022;30:3500. doi: 10.1016/j.ymthe.2022.09.021
- Ma X, Zhu G, Jiao T, et al. Effects of circular RNA Ttc3/miR-148a/Rcan2 axis on inflammation and oxidative stress in rats with acute kidney injury induced by sepsis. Life Sci. 2021;272:119233. doi: 10.1016/j.lfs.2021.119233
- Wu L, Gokden N, Mayeux PR. Evidence for the role of reactive nitrogen species in polymicrobial sepsis-induced renal peritubular capillary dysfunction and tubular injury. J Am Soc Nephrol. 2007;18:1807–1815. doi: 10.1681/asn.2006121402
- Kong P, Yu Y, Wang L, et al. Circ-Sirt1 controls NF-κB activation via sequence-specific interaction and enhancement of SIRT1 expression by binding to miR-132/212 in vascular smooth muscle cells. Nucleic Acids Res. 2019;47:3580–3593. doi: 10.1093/nar/gkz141
- Ge J, Wang J, Xiong F, et al. Epstein–barr virus–encoded circular RNA CircBART2.2 promotes immune escape of nasopharyngeal carcinoma by regulating PD-L1. Cancer Res. 2021;81(19):5074–5088. doi: 10.1158/0008-5472.can-20-4321
- Rittirsch D, Huber-Lang MS, Flierl MA, et al. Immunodesign of experimental sepsis by cecal ligation and puncture. Nat Protoc. 2009;4(1):31–36. doi: 10.1038/nprot.2008.214
- Wang P, Luo ML, Song E, et al. Long noncoding RNA lnc-TSI inhibits renal fibrogenesis by negatively regulating the TGF-β/Smad3 pathway. Sci Transl Med. 2018;10(462):eaat2039. doi: 10.1126/scitranslmed.aat2039
- Ma T, Li H, Liu H, et al. Neat1 promotes acute kidney injury to chronic kidney disease by facilitating tubular epithelial cells apoptosis via sequestering miR-129-5p. Mol Ther. 2022;30:3313–3332. doi: 10.1016/j.ymthe.2022.05.019
- Deng Z, Sun M, Wu J, et al. SIRT1 attenuates sepsis-induced acute kidney injury via Beclin1 deacetylation-mediated autophagy activation. Cell Death Dis. 2021;12:217. doi: 10.1038/s41419-021-03508-y
- Sun M, Li J, Mao L, et al. p53 deacetylation alleviates sepsis-induced acute kidney injury by promoting autophagy. Front Immunol. 2021;12:685523. doi: 10.3389/fimmu.2021.685523
- Li CM, Li M, Ye ZC, et al. Circular RNA expression profiles in cisplatin-induced acute kidney injury in mice. Epigenomics. 2019;11:1191–1207. doi: 10.2217/epi-2018-0167
- Liang WC, Wong CW, Liang PP, et al. Translation of the circular RNA circβ-catenin promotes liver cancer cell growth through activation of the wnt pathway. Genome Bio. 2019;20(1):84. doi: 10.1186/s13059-019-1685-4
- Hu X, Wu D, He X, et al. circGSK3β promotes metastasis in esophageal squamous cell carcinoma by augmenting β-catenin signaling. Mol Cancer. 2019;18:160. doi: 10.1186/s12943-019-1095-y
- Huang da W, Sherman BT, Lempicki RA. Systematic and integrative analysis of large gene lists using DAVID bioinformatics resources. Nat Protoc. 2009;4(1):44–57. doi: 10.1038/nprot.2008.211
- Huang da W, Sherman BT, Lempicki RA. Bioinformatics enrichment tools: paths toward the comprehensive functional analysis of large gene lists. Nucleic Acids Res. 2009;37:1–13. doi: 10.1093/nar/gkn923
- Kanehisa M, Furumichi M, Tanabe M, et al. KEGG: new perspectives on genomes, pathways, diseases and drugs. Nucleic Acids Res. 2017;45:D353–d361. doi: 10.1093/nar/gkw1092
- Zheng H, Huang S, Wei G, et al. CircRNA Samd4 induces cardiac repair after myocardial infarction by blocking mitochondria-derived ROS output. Mol Ther. 2022;30:3477–3498. doi: 10.1016/j.ymthe.2022.06.016
- Castro-Mondragon JA, Riudavets-Puig R, Rauluseviciute I, et al. JASPAR 2022: the 9th release of the open-access database of transcription factor binding profiles. Nucleic Acids Res. 2022;50:D165–d173. doi: 10.1093/nar/gkab1113
- Salmena L, Poliseno L, Tay Y, et al. A ceRNA hypothesis: the Rosetta Stone of a hidden RNA language? Cell. 2011;146:353–358. doi: 10.1016/j.cell.2011.07.014
- Wang X, El Naqa IM. Prediction of both conserved and nonconserved microRNA targets in animals. Bioinformatics. 2008;24:325–332. doi: 10.1093/bioinformatics/btm595
- Hu YA, Zhu Y, Liu G, et al. Expression profiles of circular RNAs in colon biopsies from Crohn’s disease patients by microarray analysis. Clinical Laboratory Analysis. 2021;35(6):e23788. doi: 10.1002/jcla.23788
- Chung H, Vilaysane A, Lau A, et al. NLRP3 regulates a non-canonical platform for caspase-8 activation during epithelial cell apoptosis. Cell Death Diff. 2016;23:1331–1346. doi: 10.1038/cdd.2016.14
- Kapranov P, Cheng J, Dike S, et al. RNA maps reveal new RNA classes and a possible function for pervasive transcription. Science. 2007;316(5830):1484–1488. doi: 10.1126/science.1138341
- He AT, Liu J, Li F, et al. Targeting circular RNAs as a therapeutic approach: current strategies and challenges. Signal Transduct Target Ther. 2021;6(1):185. doi: 10.1038/s41392-021-00569-5
- Miyaji T, Hu X, Yuen PS, et al. Ethyl pyruvate decreases sepsis-induced acute renal failure and multiple organ damage in aged mice. Kidney Int. 2003;64(5):1620–1631. doi: 10.1046/j.1523-1755.2003.00268.x
- Wang P, Ba ZF, Chaudry IH. Increase in hepatic blood flow during early sepsis is due to increased portal blood flow. Am J Physiol. 1991;261(6):R1507–1512. doi: 10.1152/ajpregu.1991.261.6.R1507
- Tsuji N, Tsuji T, Yamashita T, et al. BAM15 treats mouse sepsis and kidney injury, linking mortality, mitochondrial DNA, tubule damage, and neutrophils. J Clin Investig. 2023;133(7). doi: 10.1172/jci152401
- Privratsky JR, Ide S, Chen Y, et al. A macrophage-endothelial immunoregulatory axis ameliorates septic acute kidney injury. Kidney Int. 2023;103:514–528. doi: 10.1016/j.kint.2022.10.008
- Ding Y, Wan S, Liu W, et al. Regulation networks of non-Coding RNA-Associated ceRnas in cisplatin-induced acute kidney injury. Cells. 2022;11(19):2971. doi: 10.3390/cells11192971
- Cao Y, Mi X, Zhang D, et al. Transcriptome sequencing of circular RNA reveals a novel circular RNA-has_circ_0114427 in the regulation of inflammation in acute kidney injury. Clin Sci (Lond). 2020;134:139–154. doi: 10.1042/cs20190990
- Fang M, Liu S, Zhou Y, et al. Circular RNA involved in the protective effect of losartan on ischemia and reperfusion induced acute kidney injury in rat model. Am J Transl Res. 2019;11:1129–1144.
- Aslan A, van den Heuvel MC, Stegeman CA, et al. Kidney histopathology in lethal human sepsis. Crit Care. 2018;22(1):359. doi: 10.1186/s13054-018-2287-3
- Wang Y, Zhu J, Liu Z, et al. The PINK1/PARK2/optineurin pathway of mitophagy is activated for protection in septic acute kidney injury. Redox Biol. 2021;38:101767. doi: 10.1016/j.redox.2020.101767
- Gomez H, Ince C, De Backer D, et al. A unified theory of sepsis-induced acute kidney injury: inflammation, microcirculatory dysfunction, bioenergetics, and the tubular cell adaptation to injury. Shock. 2014;41:3–11. doi: 10.1097/shk.0000000000000052