ABSTRACT
Studies suggest that clonal plants’ ability to select habitats and forage in a heterogeneous environment is influenced by their past environment, with stress legacy potentially playing a crucial role. In this study, we examined parental ramets of Duchesnea indica Focke that were subject to either a control or lead-contaminated environment (past environment), and their newborn offspring were then transplanted into control, homogeneous lead or heterogeneous lead environment (present environment). We analysed how past and present environments affect plant growth and DNA methylation in offspring. The result shown that the DNA methylation loci composition of offspring was affected by the interaction of parental environment and offspring environment, and DNA methylation levels were higher in heterogeneous environments. Moreover, our findings indicate that offspring would thrive in the heterogeneous lead environment if they did not experience lead pollution in the past, their progeny will avoid lead toxicity by reducing underground biomass allocation. However, when the parents experienced lead stress environment, their biomass allocation strategies disappeared, and they prefer to grow in favourable patches to avoid lead-contaminated patches. We concluded that the integration of historical parental exposure to lead-contaminated and current information about their offspring’s environment are impacting plant phenotypes. It is possible that the stress legacy from the parents has been transmitted to their offspring ramets, and the stress legacy is at least partly based on heritable epigenetic variation. The phenotypic variation regulated by the stress legacy affects the growth performance, biomass allocation strategy, and even the behaviour of D. indica.
Introduction
Habitat heterogeneity is widespread in natural ecosystems and reflects environmental predictability to some extent [Citation1]. Clonal plants can sense the heterogeneity between patches and place their new ramets in appropriate patches by changing morphology, ultimately leading to habitat selection [Citation2]. This behaviour enables clonal plants to effectively utilize essential resources, improve genet fitness, and become dominant in the ecological system [Citation3,Citation4]. Clonal plants may also show a stronger ability to remember past environmental effects than non-clonal plants [Citation5]. Additionally, an increasing body of research demonstrates that the interactions of the current environment and previous environmental conditions might alter clonal plant morphology and behaviour (e.g., [Citation6–9]). It has recently been suggested that stress legacy induced by past conditions could influence the entire clonal genet [Citation10–12].
One of the most prominent possible mechanisms underlying stress legacy is epigenetic modification [Citation13,Citation14]. The alteration of epigenetic modifications can affect the phenotypes of plants, allowing them to adapt to different environmental challenges without changes to DNA sequences [Citation15]. DNA methylation variation is one of the important epigenetic modifications and is often linked to phenotypic variation [Citation16]. It has been shown to cause heritable variations in a large number of ecologically important plant traits, such as stress tolerance and phenotypic plasticity [Citation17].
Plants respond to environmental stress by developing resistance mechanisms. This resistance can be inherited across generations [Citation18]. This ability is particularly important for sessile organisms, such as plants, to adjust to spatial and temporal environmental heterogeneity [Citation19,Citation20]. Phenotypic variation and adaptive changes induced by epigenetics modification can be successfully passed on to the next generation, which may be a potential mechanism for clonal plants to rapidly occupy habitats and adapt to environmental changes over a long evolutionary period [Citation11,Citation21]. This success can be attributed to the sharing of epigenetic information between clonal generations, avoiding resetting of most environmentally induced epigenetic information during the meiosis of sexual reproduction [Citation11,Citation22]. Hence, clonal plants are considered to be prime examples of utilizing epigenetic domestication to adapt to natural selection [Citation23]. For instance, the newly emerging offspring ramets of Trifolium repens can inherit epigenetic information from their parental ramet, allowing them to adapt more effectively to environmental challenges [Citation21,Citation24]. Another example of the inheritance of phenotypic adaptability is the dwarf phenotypic Leymus chinensis, long-term overgrazing induced memory effect in morphological plasticity and physiologically adaptive photosynthetic function of clonal plants, significantly affects its clonal growth in subsequent generations [Citation25].
Studies have demonstrated that stress caused by temperature, moisture, high salt, heavy metals, and other factor influences the expression of stress response genes by altering DNA methylation, and this enhances the ability of plants to adapt to their environment (e.g., [Citation15–28]). The issue of soil contamination with heavy metals, including lead (Pb), has become increasingly problematic [Citation29]. Pb is a toxic heavy metal in contaminated soil that negatively affects plant growth and yield [Citation30]. Previous studies have shown that Duchesnea indica Focke can select between Pb-contaminated and uncontaminated patches of soil in addition to displaying different growth patterns in various environments depending on the degree of heterogeneity [Citation31]. However, it is unclear how the parental environment affects the habitat selection of offspring in heterogeneous environments in general, i.e., not specifically referring to heterogeneous environments in lead distribution. Whether stress legacy induced by the parent plays a significant role, and the mechanisms underlying specific responses of clonal plants in heterogeneous environments.
Therefore, our study investigated the role of stress legacy in the habitat selection of clonal plants D. indica in a heterogeneous Pb-polluted environment. We treated the parental ramets with either control or Pb-contaminated conditions to induce stress legacy. Then, we exposed the offspring ramets to three different environmental conditions to examine the effect of parental stress legacy on offspring growth. We hypothesize that (1) past and present Pb environments affect the growth of clonal plants, and Duchesnea indica Focke respond to heterogeneous environments with different past Pb experiences by either prolonging their stolons to escape from the stress patches or altering their biomass allocation; (2) DNA methylation induces the stress legacy and plant phenotypic variation to mediate the habitat selection mechanisms in Pb contaminated environments.
Materials and methods
Experimental species and propagation
Duchesnea indica Focke is a perennial evergreen herb that can form a clonal system through vegetative spread, with offspring ramets connected by stolons. It was shown that stolon clonal plants have a greater ability to forage for resources than those with rhizomes [Citation32]. To ensure a consistent genetic base among the ramets selected for our experiment, a single clone of D. indica was collected from Northwest University garden in Xi’an, Shaanxi province of China (34.3°N, 108.9°E, altitude 397 m) and transplanted into pots filled with soil composed of 25% sand, 25% organic matter and 50% peat. The plants were then placed in a greenhouse to allow for asexual propagation before the start of the experiment. For the experiment, we selected and replanted similar-sized ramets with no newborn offspring, which served as the parents in our study.
The experiment was conducted at Northwest University from July 13 to 13 September 2017. During the experiment, the greenhouse had a mean irradiance of 357 ± 20 μ mol m−2 s−1 at midday, a humidity of 40 ± 5%, a regulated temperature (25/18°C day/night cycle), and light regime (12-/12-h light/night cycle).
Experimental design
Similar-sized parental ramets (fresh weight = 0.88 ± 0.12 g) were planted in the centre of the plastic pot (7 cm long × 7 cm wide × 7 cm high). Half of the pots containing parental ramets were filled with Pb-contaminated soil, while the other half were filled with uncontaminated soil. Offspring ramet pots were closely arranged to parental ramet pots, and each offspring ramet pot was filled with either contaminated or uncontaminated soil, as detailed in .
Figure 1. Schematic of the experiment. The design consisted of two past environments (past env.) (control and Pb contaminated) and three Pb contaminated levels of present environments (present env.) (control, heterogeneous Pb, and homogeneous Pb) for the newborn ramets. The past environment is represented inside the red square, present environment is represented outside the red square. See the text and table 1 for experimental design details.
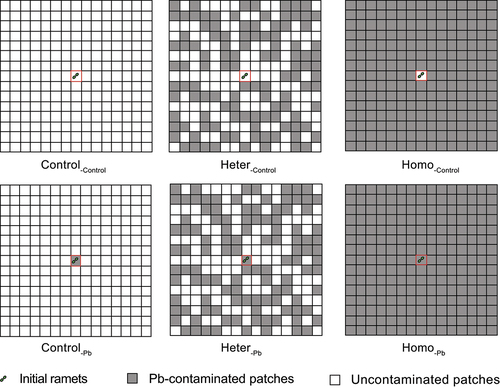
Each pot represented a habitat patch, and the quality of the patch was determined by the soil condition (Pb-contaminated or uncontaminated). The parental ramet patch was either Pb-contaminated or controlled (past environment). In total, 225 (15 columns × 15 rows) pots were used for treatment, with three treatments (n = 6 plants per treatment) based on the growing conditions of the offspring ramets (control vs homogeneous Pb vs heterogeneous Pb, representative of the present environment), as described in . The placement of the the contaminated patches for the heterogeneous Pb treatment was randomly generated using Microsoft Excel 2010 (Microsoft, US), and the layout of the containers was designed using ensure equal chances of colonization in the Pb-contaminated and uncontaminated patches.
Table 1. Different treatments conducted in the study.
After transplanting, the parental plants were cultured for two months. The plants were watered three times per week, with 80% field capacity to ensure that Pb did not leach out of the soils. The position of each experimental group was moved periodically within the greenhouse to eliminate the effects of the microenvironment.
The contaminated soil was treated with 2000 mg·kg−1 of Pb by adding Pb (CH3COO)2 solution, which had been determined to be a stress concentration for D. indica based on our preparatory experiments.
Measurement of parameters
At the end of the experiment, the stolon length of each branch, node number, and the number of offspring ramets were recorded, we used the longest stolon length as stolon length, and the entire clone for each treatment was harvested. Ramets were separated into parent aboveground, parent underground, offspring leaf, offspring stolon, and offspring root, then dried at 70°C for 72 h, and weighed. Specific stolon length (calculated as total offspring stolon length divided by total offspring stolon mass) and the ratio of root/shoot (R/S) were also determined (calculated as root mass divided by shoot mass; shoot mass contains parent aboveground mass, offspring leaf mass, and offspring stolon mass; root mass contains parent underground mass and offspring root mass).
Methylation-sensitive amplified polymorphism analyses
Methylation-sensitive amplified polymorphism analyses were used to detect methylation alterations in the cytosine modification of D. indica. Fresh, unrooted offspring leaves from each of the six treatments were collected and tested for their cytosine methylation level and methylation loci composition (the distribution of bands of the gel electrophoresis). The 30 mg dry leaves dried in silica gel were used to extract total genomic DNA by BioTeKe (Beijing, China). Then we examined DNA quality and quantity using the NanoDrop2000 (Thermo Scientific, United States). We followed the digestion, ligation reactions of endonuclease, pre-amplification, and selective amplification methods described in Quan et al. [Citation33]. The specific selective amplification primer combinations are shown in . The methylated state of each locus was scored by the two enzymatic reactions. Our analyses included three methylation states: unmethylated (1/1), hemimethylated or internal methylation ((1/0) or (0/1)), and uninformative (0/0) [Citation34–36].
Statistical analyses
Two-way ANOVA was used to test the effects of the parental environment (control vs. Pb), offspring environment (control, homogeneous Pb vs. heterogeneous Pb), and their interaction on the total biomass, R/S, leaf biomass, stolon biomass, underground biomass, and specific stolon biomass. We also included the initial size of the parental ramets and their interaction with other variables in the models. Data were transformed when needed(log) to confirm the assumptions of homoscedasticity and normality. Node number and branch number followed Poisson distribution, and the effects of parental and offspring environment and their interaction on these variables were tested using a generalized linear model with the respective distribution.
The ‘vegan’ package of R [Citation37] was used to calculate Shannon’s diversity index of each individual. The methylation level (%) was calculated by dividing MSAP bands that represent methylated 5’-CCGG sites by the total number of scored bands [Citation38]. Canonical correspondence analysis(CCA) with the capscale function in the ‘vegan’ package of R [Citation37,Citation39] was used to analyse the binary matrix of methylated state (Loci composition). Regression analysis was performed to assess how the past environment (control vs Pb) or present environment (control vs homogeneous Pb vs heterogeneous Pb) affects loci composition. The Shannon’s diversity index and methylation level were assessed using a generalized linear model. All analyses were performed using R 3.5.1.
Results
Phenotype of the whole plants
We found that only stolon length was significantly affected by the past environment. In particular, plants that had grown in a Pb-contaminated environment in the past had shorter stolon than those that had grown in a controlled environment (, ).
Figure 2. Past environment (control versus Pb) and present environment (control, heterogeneous Pb, and homogeneous Pb) on morphological and growth performance of Duchesnea indica. total biomass (a); leaf biomass(b); stolon biomass(c); root biomass(d); stolon length(e); specific stolon length(f); R/S(g); branch number(h) and node number(i) of wholegenet. Means and SE are shown. Columns sharing the same letter are not significantly different from each other at p < 0.05.
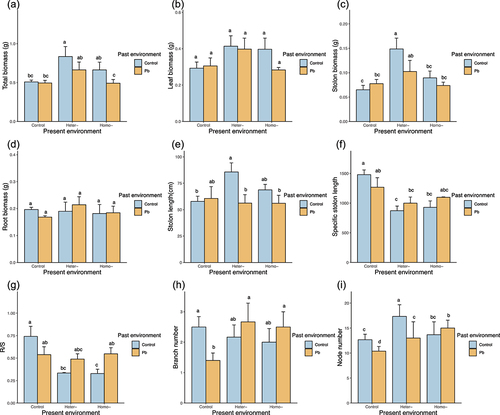
Table 2. ANOVA result for effects of past environment (control versus Pb), present environment (control, heterogeneous Pb versus homogeneous Pb), and their interaction on the morphological and growth performance of Duchesnea indica.
On the other hand, the total biomass was significantly affected by the present environment. The newborn offspring that grew in a heterogeneous Pb environment showed higher total biomass compared to those that grew in a homogeneous Pb or control environment (). This trend was also observed for stolon biomass (). Moreover, the specific stolon length was significantly higher in the control environment ().
The interaction between past and present environments significantly affected the R/S ratio. When the plants had grown in the control treatment in the past, their R/S ratio was lower in heterogeneous Pb and homogeneous Pb environments than in the control environment. However, when plants had grown in the Pb environment in the past, there were no significant differences observed between the three present environments (). None of the other plant phenotypes were significantly influenced by the past or present environment ().
Methylation of the newborn plants
In total, 257 MSAP loci were amplified from 24 newborn plant samples using 12 different primer pair combinations. Loci composition, but not diversity and methylation level, was significantly affected by parental environment. The offspring environment (control, homogeneous Pb vs. heterogeneous Pb) marginally affected Shannon’s diversity index (Iepi) and significantly affected loci composition and overall DNA methylation level(Lepi). (, off). Lepi and Iepi were found to be significantly or marginal significantly higher in ramets grown in heterogeneous Pb environment (Iepi = 4.51 ± 0.05; Lepi = 40.03 ± 1.57%) compared to control environment (Iepi = 4.28 ± 0.06; Lepi = 31.42 ± 1.73%) and homogeneous Pb subsequent environment (Iepi = 4.39 ± 0.07; Lepi = 36.72 ± 2.90%). There were significant differences between the loci composition of newborn plants grown in past and present environments (, Pa × Pr, ), so we tested the effects separately.
Table 3. ANOVA/CCA analysis results for effects of the past environment (control vs. Pb), and present environment (control, heterogeneous Pb, and homogeneous Pb) on loci composition, Shannon’s diversity index, and total DNA methylation level of offspring Duchesnea indica.
Differences in loci composition were observed when past ramets grew in Pb-contaminated environments compared to control environments (, , F = 1.91, p = 0.002). The loci composition was linearly increased when Duchesnea indica past grew in control environments, while it showed a decreasing trend in Pb past environments ().
Figure 4. Relationship between CCA1 score and CCA2 score in loci composition of offspring ramets at different past environments.
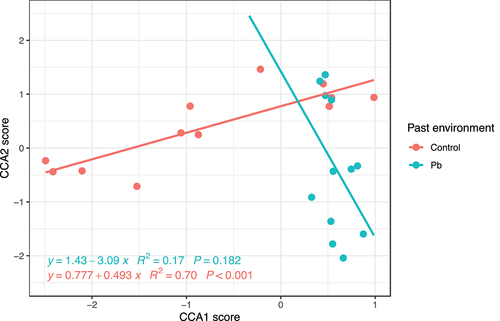
The present environment significantly affected the loci composition (F = 1.62, p = 0.002, , ). The loci composition showed a steady increase in the control treatment, but it seemed to decrease when the offspring grew in the homogeneous Pb and heterogeneous Pb treatments ().
Discussion
The phenotypic plasticity of clonal plants is influenced by environmental heterogeneity and parental environment
In our experiment, we found that plants grown in a heterogeneous environment with Pb produced more biomass than the other groups under the present environment. This finding is consistent with previous findings on the growth of clonal plants in various heterogeneous environments with different essential and non-essential resources (e.g., light, water, nutrients, copper, cadmium, lead, etc.) [Citation31,Citation40,Citation41]. Moreover, the plants in the Pb-contaminated treatment, whether heterogeneous or homogeneous, showed a lower R/S ratio. This indicates that D. indica had better growth in a Pb-contaminated environment by using an effective survival strategy to allocate more biomass to the aboveground parts and reduce the uptakes of Pb by roots, thus avoiding the toxic effects of Pb on the whole plant. However, this finding differs from previous observations on the growth of clonal plants in heterogeneous essential resources environments, where the R/S ratio was higher in nutrient patches [Citation41]. Therefore, in heterogeneous environments, the allocation of biomass to roots may depend on whether the plant grows in an essential or non-essential resource environment. Additionally, we found that under heterogeneous Pb-contaminated treatment, stolon biomass was higher, but stolon length did not change significantly. This contrasts with previous findings on plants grown in Pb-contaminated environments with concentrations three times lower than our study [Citation31], where D. indica expanded its growth architecture through longer stolons and higher aboveground biomass to accelerate risk-spreading. Thus, the concentration of Pb stress may have varying effects on plant growth.
Furthermore, our study provides evidence that clonal plants exposed to different Pb-contaminated environments in the past will produce different phenotypes during growth and development. The length of a primary stolon is a characteristic of the foraging response [Citation42]. The parental ramet that was grown in a past environment contaminated with Pb had a shorter stolon length compared to those grown in a controlled environment. This could be due to severe Pb toxicity stress of Pb, which inhibits the cell division of plants [Citation43,Citation44]. Consequently, stolon prolongation is affected. This finding is consistent with some previous studies. For example, the stoloniferous clonal plant Potentilla anserine produced shorter stolon internodes at higher soil levels of heavy metals, such as copper and nickel than in control environments [Citation45]. In addition, parental shading of Alternanthera philoxeroides inhibited early stem growth, producing shorter and thinner stolon internodes in shaded conditions [Citation6]. The stolons of Cynodon dactylon were slightly shorter under lower nutrient levels [Citation46]. However, contrary reports have suggested that clonal plants will increase stolon length in poor-quality habitats, allowing plants to ‘forage’ for the best microsites [Citation47]. Further research is necessary to clarify the phenotypic plasticity of clonal plants in various environments.
The phenotypic plasticity of clonal plants is influenced by both parental and progeny environments
Clonal plants possess the ability to exhibit phenotypic plasticity and clonal integration enables the sharing of information about environmental quality and variability between ramets [Citation48]. The quality and availability of resources for parents and their progeny can impact the development of future generations [Citation49]. Our experiment revealed that both the past and present environments affect the allocation of R/S. Specifically, offspring grown in a Pb contaminant environment, regardless of whether it was homogeneous or heterogeneous, had lower underground biomass compared to those grown in a controlled environment. In general, less root distribution may be beneficial for plant growth in contaminated soil environments. Our results suggest that there may be specialization and division of labour among clonal plant species, they would like to adjust the allocation of biomass to adapt to their environment [Citation31,Citation50,Citation51]. Interestingly, we found that when the plant’s past environment was also a Pb environment, there were no differences in biomass allocation between the three present environments (control, heterogeneous Pb, and homogeneous Pb). This could be due to clonal integration, where clonal plants can share information through their connection stolons [Citation9,Citation33,Citation52]. Parental stress legacy may enhance the clonal integration ability of the progeny, which could lead to no changes in biomass allocation. Additionally, the distance between ramets has been found to affect the duration of legacy maintenance. A study conducted on P. reptans and P. anserina revealed that the decision of where a new ramet settles depends not only on the resource experienced by the latest ramet but also on the ramets that preceded it [Citation9]. Based on theoretical considerations, parental effects are typically greater in early development, and the influence of parental effects gradually decreases as offspring can sense and respond to their environment [Citation53,Citation54].
Our study involved subjecting parents and offspring to consistent and varied environmental treatments. We found that the allocation of the R/S ratio was higher when parents and offspring were in matched environments (Control−Control and Homo−Pb) than when they grew in different Pb environments (Homo−Control and Control−Pb). Dong et al. [Citation55] found that parental effects are dependent on the offspring’s environment to some extent. Positive parental effects are amplified when the offspring’s environment is similar to their parental environment [Citation56,Citation57]. Parental effects could facilitate the preadaptation of offspring to their parental environment by changing their biomass, root carbohydrate storage accumulation, and stress tolerance ability. For instance, Arabidopsis parental plants exposed to hyperosmotic stress produced offspring with higher survival rates in high-salt conditions [Citation58]. This adaptive response is maintained across multiple generations in the presence of hyperosmotic stress but is lost in the F2 generation when the F1 offspring are raised under control conditions.
The stress legacy affect the progeny of the clonal plant D. indica
Phenotypic variation driven by environmental change is associated with DNA methylation [Citation16]. It has been shown that the parents methylation level and status of clonal plants can be stably transmitted to their offspring, thus affecting the methylation status and growth of their offspring (e.g., [Citation12,Citation15,Citation24,Citation59]). In this study, we investigated whether DNA methylation changes in the progeny of the clonal plant D. indica were regulated by both past and present environments and whether the stress legacy induced by DNA methylation in parental plants played a role in its offspring growth. Our results showed that the interaction between past and present heterogeneous environments significantly affected the DNA methylation loci composition and R:S ratio of the offspring. This suggests that parental DNA methylation leads to stress legacy and may affect the allocation of D. indica growth. Revealed that stress legacy induced by the parent environment regulates the behaviour of clonal plants in heterogeneous environments to some extent and the stress legacy is at least partly based on heritable epigenetic variation.
Many studies focused on the same or different environments of clonal plant progeny and parents [Citation6,Citation7], However, clonal plants often face heterogeneous environments in natural environments, while it’s in heterogeneous environments usually tend to perform habitat selection [Citation2,Citation41]. Also, studies have shown that DNA methylation may be involved in the habitat selection behaviour of clonal plants [Citation33,Citation42]. Our results showed that overall DNA methylation levels (Lepi) were significantly higher in ramets inhabiting heterogeneous Pb environments compared to those in homogenous environments. This suggests that DNA methylation legacy may be involved in the habitat selection of the D. indica in Pb heterogeneous environments. Furthermore, our findings showed variations in the total biomass, stolon biomass, and specific stolon length of plants in heterogeneous Pb environments compared to homogeneous ones. Heterogeneous environments enable clonal plants to experience Pb patches repeatedly, which may deeply induce plant DNA methylation legacy, and then, leading to transcriptional and physiological responses during subsequent stress [Citation60,Citation61].
Overall, the stress legacy of the past parental plant was transmitted across generations in our study, affecting the present offspring plant’s phenotypic variation, growth performance, and biomass allocation strategy. Previous studies have shown that environmental stress can induce plant methylation changes that affect plant growth and development [Citation26]. Lira-Medeiros et al. analysed the cytosine methylation levels of two mangrove populations with the same genetic background but different phenotypes growing in different environments. They found that the methylation level of mangrove trees growing in swamps was lower than that of mangrove trees growing in riverside, and the height, trunk diameter, leaf width, and leaf area of mangroves growing in marshes also decreased [Citation62]. The transgenerational effect of phenotypic plasticity has also been confirmed by other studies on clonal plants, such as dandelion under salt stress, where transgenerational DNA methylation changes cause transgenerational phenotypic changes, and the leaf morphology and R/S of its offspring are different from the control [Citation59]. Drought stress can also affect the DNA methylation level of Trifolium repens parental strains, inducing their offspring to form the legacy of drought stress, which changes the offspring phenotype [Citation12]. Besides, for clonal plants, the biggest defect is the lack of genetic diversity, which can be remedied by stress legacy induced by epigenetics [Citation23]. Compared with mutation, epigenetic modification can play an essential role in a shorter time, and phenotypic variation induced by stress legacy is particularly important in coping with rapid environmental changes [Citation8]. Future research should involve more detailed molecular and biochemical mechanisms when studying clonal stress legacy. This will better help us understand the ecological and evolutionary aspects of transgenerational effects in clonal plants.
Conclusions
Our study has shown that the parental ramets exposed to Pb contamination in the past can significantly affect the growth and behaviour of their present offspring ramets in a heterogeneous environment. The offspring’s growth (R/S) and epigenetic variation (loci composition) are affected by the integration of past and present information about environmental variability. Interestingly, we found that plants grown in a heterogeneous Pb environment exhibited better growth than those in a homogeneous environment (control and homogeneous Pb). However, contrary to our hypothesis, the results showed that Pb-contaminated parents did not adopt a longer stolon to escape from pollution; instead, they exhibited a shorter stolon length. This could be attributed to the inhibition of stolon cell division by Pb toxicity stress and the influence of stress legacy on the phenotype of progeny to a certain extent. In conclusion, our results showed that past environmental information is significant in determining the performance of present offspring ramets, which is at least partly related to the parental legacy via epigenetic variation.
Authors’ contributions
X Liu conceived and designed the experiments. SS Song performed the experiments, JX Quan and Linya Xing helped with the data analysis, JX Quan and SS Song wrote the manuscript. Others provided editorial advice. All authors read and approved the final manuscript.
Disclosure statement
No potential conflict of interest was reported by the author(s).
Data availability statement
The data that support the findings of this study are available from the corresponding author.
Additional information
Funding
References
- Oborny B. Growth rules in clonal plants and environmental predictability -- a simulation study. J Ecol. 1994;82(2):341–13. doi: 10.2307/2261302
- Hutchings MJ, John EA. The effects of environmental heterogeneity on root growth and root/shoot partitioning. Ann Bot. 2004;94(1):1–8. doi: 10.1093/aob/mch111
- Oborny B, Hubai AG. Patch size and distance: modelling habitat structure from the perspective of clonal growth. Ann Bot. 2014;114(2):389–398. doi: 10.1093/aob/mcu110
- Waters EM, Watson MA. Live substrate positively affects root growth and stolon direction in the woodland strawberry, Fragaria vesca. Front Plant Sci. 2015;6:1–10. doi: 10.3389/fpls.2015.00814
- Latzel V, Klimešová J. Transgenerational plasticity in clonal plants. Evol Ecol. 2010;24(6):1537–1543. doi: 10.1007/s10682-010-9385-2
- Dong BC, Meng J, Yu FH, et al. Effects of parental light environment on growth and morphological responses of clonal offspring. Plant Biol J. 2019;21(6):1083–1089. doi: 10.1111/plb.13001
- Guo YH, Quan JX, Wang XB, et al. Predictability of parental ultraviolet-B environment shapes the growth strategies of clonal Glechoma longituba. Front Plant Sci. 2022;13:949752. doi: 10.3389/fpls.2022.949752
- Latzel V, González APR, Rosenthal J. Epigenetic memory as a basis for intelligent behavior in clonal plants. Front Plant Sci. 2016;7:1354. doi: 10.3389/fpls.2016.01354
- Louâpre P, Bittebière AK, Clément B, et al. How past and present influence the foraging of clonal plants? PloS One. 2012;7(6):e38288. doi: 10.1371/journal.pone.0038288
- Latzel V, Münzbergová Z. Anticipatory behavior of the clonal plant Fragaria vesca. Front In Plant Sci. 2018;9:1847. doi: 10.3389/fpls.2018.01847
- Münzbergová Z, Hadincová V. Transgenerational plasticity as an important mechanism affecting response of clonal species to changing climate. Ecol Evol. 2017;7(14):5236–5247. doi: 10.1002/ece3.3105
- Quan JX, Münzbergová Z, Latzel V. Time dynamics of stress legacy in clonal transgenerational effects: a case study on Trifolium repens. Ecol Evol. 2022;12(5):e8959. doi: 10.1002/ece3.8959
- Li P, Yang H, Wang L, et al. Physiological and transcriptome analyses reveal short-term responses and formation of memory under drought stress in rice. Front Genet. 2019;10:55. doi: 10.3389/fgene.2019.00055
- Walter J, Jentsch A, Beierkuhnlein C, et al. Ecological stress memory and cross stress tolerance in plants in the face of climate extremes. Environ Exp Bot. 2013;94:3–8. doi: 10.1016/j.envexpbot.2012.02.009
- Verhoeven KJF, Jansen JJ, Van Dijk PJ, et al. Stress induced DNA methylation changes and their heritability in asexual dandelions. New Phytol. 2010;185(4):1108–1118. doi: 10.1111/j.1469-8137.2009.03121.x
- Angers B, Castonguay E, Massicotte R. Environmentally induced phenotypes and DNA methylation: how to deal with unpredictable conditions until the next generation and after. Mol Ecol. 2010;19(7):1283–1295. doi: 10.1111/j.1365-294X.2010.04580.x
- Zhang YY, Fischer M, Colot V, et al. Epigenetic variation creates potential for evolution of plant phenotypic plasticity. New Phytol. 2013;197(1):314–322. doi: 10.1111/nph.12010
- Mirouze M, Paszkowski J. Epigenetic contribution to stress adaptation in plants. Curr Opin Plant Biol. 2011;14(3):267. doi: 10.1016/j.pbi.2011.03.004
- Pigliucci M. Evolution of phenotypic plasticity: where are we going now? Trends Ecol Evol. 2005;20(9):481–486. doi: 10.1016/j.tree.2005.06.001
- van Kleunen M, Fischer M. Constraints on the evolution of adaptive phenotypic plasticity in plants. New Phytol. 2005;166(1):49–60. doi: 10.1111/j.1469-8137.2004.01296.x
- González APR, Chrtek J, Dobrev PI, et al. Stress-induced memory alters growth of clonal offspring of white clover (Trifolium repens). Am J Bot. 2016;103(9):1–8. doi: 10.3732/ajb.1500526
- Verhoeven KJF, Preite V. Epigenetic variation in asexually reproducing organisms. Evolution. 2014;68(3):644–655. doi: 10.1111/evo.12320
- Douhovnikoff V, Dodd RS. Epigenetics: a potential mechanism for clonal plant success. Plant Ecol. 2015;216(2):227–233. doi: 10.1007/s11258-014-0430-z
- González APR, Dumalasová V, Rosenthal J, et al. The role of transgenerational effects in adaptation of clonal offspring of white clover (Trifolium repens) to drought and herbivory. Evol Ecol. 2017;31(3):345–361. doi: 10.1007/s10682-016-9844-5
- Ren WB, Hu NN, Hou XY, et al. Long-term overgrazing-induced memory decreases photosynthesis of clonal offspring in a perennial grassland plant. Front Plant Sci. 2017;8:419. doi: 10.3389/fpls.2017.00419
- Chinnusamy V, Zhu JK. Epigenetic regulation of stress responses in plants. Curr Opin Plant Biol. 2009;12(2):133–139. doi: 10.1016/j.pbi.2008.12.006
- Kinoshita T, Seki M. Epigenetic Memory for stress response and adaptation in plants. Plant Cell Physiol. 2014;55(11):1859–1863. doi: 10.1093/pcp/pcu125
- Laanen P, Saenen E, Mysara M, et al. Changes in DNA methylation in Arabidopsis thaliana plants exposed over multiple generations to gamma radiation. Front Plant Sci. 2021;12:611783. doi: 10.3389/fpls.2021.611783
- Hu PJ, Li Z, Zhong DX, et al. Research progress on the phytoextraction of heavy metal contaminated soils in China. Plant Physiol J. 2014;50:577–584. in Chinese with English abstract.
- Duan DC, Yu MG, Shi JY. Research advances in uptake, translocation, accumulation and detoxification of Pb in plants. Chin J Of Appl Ecol. 2014;25:287–296. in Chinese with English abstract.
- Quan JX, Zhang XY, Song SS, et al. Clonal plant Duchesnea indica focke forms an effective survival strategy in different degrees of Pb-contaminated environments. Plant Ecol. 2018;219(11):1315–1327. doi: 10.1007/s11258-018-0881-8
- Xie XF, Song YB, Zhang YL, et al. Phylogenetic meta-analysis of the functional traits of clonal plants foraging in changing environments. PloS One. 2014;9(9):e107114. doi: 10.1371/journal.pone.0107114
- Quan JX, Latzel V, Tie D, et al. Ultraviolet B radiation triggers DNA methylation change and affects foraging behavior of the clonal plant Glechoma longituba. Front Plant Sci. 2021;12:633982. doi: 10.3389/fpls.2021.633982
- Pérez Figueroa A. MSAP: a tool for the statistical analysis of methylation-sensitive amplified polymorphism data. Mol Ecol Resour. 2013;13(3):522–527. doi: 10.1111/1755-0998.12064
- Salmon A, Clotault J, Jenczewski E, et al. Brassica oleracea displays a high level of DNA methylation polymorphism. Plant Sci. 2008;174(1):61–70. doi: 10.1016/j.plantsci.2007.09.012
- Wang MZ, Li HL, Li JM, et al. Correlations between genetic, epigenetic and phenotypic variation of an introduced clonal herb. Heredity. 2019;124(1):146–155. doi: 10.1038/s41437-019-0261-8
- Dixon P. VEGAN, a package of R functions for community ecology. J Veg Sci. 2003;14(6):927–930. doi: 10.1111/j.1654-1103.2003.tb02228.x
- Liu S, Sun K, Jiang T, et al. Natural epigenetic variation in the female great roundleaf bat (Hipposideros armiger) populations. Mol Genet Genomics. 2012;287(8):643–650. doi: 10.1007/s00438-012-0704-x
- Bonin A, Ehrich D, Manel S. Statistical analysis of amplified fragment length polymorphism data: a toolbox for molecular ecologists and evolutionists. Mol Ecol. 2007;16(18):3737–3758. doi: 10.1111/j.1365-294X.2007.03435.x
- Hutchings MJ, Wijesinghe DK. Performance of a clonal species in patchy environments: effects of environmental context on yield at local and whole-plant scales. Evol Ecol. 2008;22(3):313–324. doi: 10.1007/s10682-007-9178-4
- Xiao KY, Yu D, Wang JW. Habitat selection in spatially heterogeneous environments: a test of foraging behaviour in the clonal submerged macrophyte Vallisneria spiralis. Freshwater Biol. 2006;51(8):1552–1559. doi: 10.1111/j.1365-2427.2006.01590.x
- Tie D, Guo YH, Zhu CR, et al. Parental UV-B radiation regulates the habitat selection of clonal Duchesnea indica in heterogeneous light environments. Funct Plant Biol. 2022;49(7):600–612. doi: 10.1071/FP21253
- Pourrut B, Shahid M, Dumat C, et al. Lead uptake, toxicity, and detoxification in plants. Rev Environ Contam T. 2011;213:113–136.
- Shahid M, Pinelli E, Pourrut B, et al. Lead-induced genotoxicity to Vicia faba L. roots in relation with metal cell uptake and initial speciation. Ecotox Environ Safe. 2011;74(1):78–84. doi: 10.1016/j.ecoenv.2010.08.037
- Koivunen S, Saikkonen K, Vuorisalo T, et al. Heavy metals modify costs of reproduction and clonal growth in the stoloniferous herb Potentilla anserina. Evol Ecol. 2004;18(5–6):541–561. doi: 10.1007/s10682-004-5143-7
- Dong M, de Kroon H. Plasticity in morphology and biomass allocation in Cynodon dactylon, a grass species forming stolons and rhizomes. Oikos. 1994;70(1):99–106. doi: 10.2307/3545704
- Kleijn D, van Groenendael JM. The exploitation of heterogeneity by a clonal plant in habitats with contrasting productivity levels. J Ecol. 1999;87(5):873–884. doi: 10.1046/j.1365-2745.1999.00406.x
- Price EAC, Marshall C, Hutchings MJ. Studies of growth in the clonal herb Glechoma hederacea. I. Patterns of physiological integration J Ecol. 1992;80(1):25–38. doi: 10.2307/2261060
- Waterman R, Sultan SE. Transgenerational effects of parent plant competition on offspring development in contrasting conditions. Ecology. 2021;102(12):e03531. doi: 10.1002/ecy.3531
- Hutchings MJ, Wijesinghe DK. Patchy habitats, division of labour and growth dividends in clonal plants. Trends Ecol Evol. 1997;12(10):390–394. doi: 10.1016/S0169-5347(97)87382-X
- Wang N, Yu FH, Li PX, et al. Clonal integration affects growth, photosynthetic efficiency and biomass allocation, but not the competitive ability, of the alien invasive Alternanthera philoxeroides under severe stress. Ann Bot. 2008;101(5):671–678. doi: 10.1093/aob/mcn005
- Roiloa SR, Retuerto R. Clonal integration in Fragaria vesca growing in metal-polluted soils: parents face penalties for establishing their offspring in unsuitable environments. Ecol Res. 2012;27(1):95–106. doi: 10.1007/s11284-011-0876-6
- Auge GA, Leverett LD, Edwards BR, et al. Adjusting phenotypes via within- and across-generational plasticity. New Phytol. 2017;216(2):343–349. doi: 10.1111/nph.14495
- Uller T. Developmental plasticity and the evolution of parental effects. Trends Ecol Evol. 2008;23(8):432–8. doi: 10.1016/j.tree.2008.04.005
- Dong BC, van Kleunen M, Yu FH. Context-dependent parental effects on clonal offspring performance. Front Plant Sci. 2018;9:1824. doi: 10.3389/fpls.2018.01824
- Galloway LF, Etterson JR. Transgenerational plasticity is adaptive in the wild. Science. 2007;318(5853):1134–1136. doi: 10.1126/science.1148766
- Latzel V, Janecek Š, Doležal J, et al. Adaptive transgenerational plasticity in the perennial Plantago lanceolata. Oikos. 2014;123(1):41–46. doi: 10.1111/j.1600-0706.2013.00537.x
- Wibowo A, Becker C, Marconi G, et al. Hyperosmotic stress memory in Arabidopsis is mediated by distinct epigenetically labile sites in the genome and is restricted in the male germline by DNA glycosylase activity. eLife 5. 2016;5:e13546. doi: 10.7554/eLife.13546
- Verhoeven KJF, van Gurp TP. Transgenerational effects of stress exposure on offspring phenotypes in Apomictic dandelion. PLoS One. 2012;7(6):e38605. doi: 10.1371/journal.pone.0038605
- Ding Y, Virlouvet L, Liu N, et al. Dehydration stress memory genes of Zea mays; comparison with Arabidopsis thaliana. BMC Plant Biol. 2014;14(1):141. doi: 10.1186/1471-2229-14-141
- Virlouvet L, Avenson TJ, Du Q, et al. Dehydration stress memory: gene networks linked to physiological responses during repeated stresses of Zea mays. Front Plant Sci. 2018;9:1058. doi: 10.3389/fpls.2018.01058
- Lira-Medeiros CF, Cardoso MA, Fernandes RA, et al. Analysis of genetic diversity of two mangrove species with morphological alterations in a natural environment. Diversity. 2015;7(2):105–117. doi: 10.3390/d7020105