Abstract
Ascorbate (AsA)–glutathione (GSH) cycle metabolism has been regarded as the most important defense mechanism for the resistance of plants under stress. In this study the influence of salicylic acid (SA) was studied on ascorbate-glutathione pathway, S-assimilation, photosynthesis and growth of mustard (Brassica juncea L.) plants subjected to 100 mM NaCl. Treatment of SA (0.5 mM) alleviated the negative effects of salt stress and improved photosynthesis and growth through increase in enzymes of ascorbate-glutathione pathway which suggest that SA may participate in the redox balance under salt stress. The increase in leaf sulfur content through higher activity of ATP sulfurylase (ATPS) and serine acetyl transferase (SAT) by SA application was associated with the increased accumulation of glutathione (GSH) and lower levels of oxidative stress. These effects of SA were substantiated by the findings that application of SA-analog, 2,6, dichloro-isonicotinic acid (INA) and 1 mM GSH treatment produced similar results on rubisco, photosynthesis and growth of plants establishing that SA application alleviates the salt-induced decrease in photosynthesis mainly through inducing the enzyme activity of ascorbate-glutathione pathway and increased GSH production. Thus, SA/GSH could be a promising tool for alleviation of salt stress in mustard plants.
Abbreviations
ATPS | = | ATP-sulfurylase |
AsA-GSH | = | Ascorbate-glutathione |
APX | = | Ascorbate peroxidase |
CAT | = | Catalase |
Cys | = | Cysteine |
DAS | = | Days after sowing |
DHA | = | Dehydroascorbate |
DHAR | = | Dehydroascorbate reductase |
Fv/Fm | = | maximal PS II photochemical efficiency |
GR | = | Glutathione reductase |
GSH | = | Reduced glutathione |
GSSG | = | Oxidized glutathione |
ROS | = | Reactive oxygen species |
RuBP | = | ribulose 1, 5-bisphosphate |
gs | = | stomatal conductance |
S | = | sulfur |
SAT | = | Serine acetyl transferase |
TBARS | = | Thiobarbituric acid reactive substances |
WUE | = | water use efficiency |
Introduction
Salt stress is one of the most widespread abiotic stresses which adversely affects plant processes at physiological, biochemical and molecular level and reduces plant productivity.Citation1,2 Salinization is rapidly increasing on a global scale and currently effects more than 10% of arable land resulting in greater than 50% decline in the average yields of major crops.Citation3 Salt stress induces oxidative stress through the over production of reactive oxygen species (ROS) that trigger lipid peroxidation, damage to photosynthetic pigments and disturbance in mineral nutrient status.Citation4,5 The inhibitory effect of salt stress on photosynthetic rate may involve stomatal or non-stomatal limitations and inhibition of photochemical processesCitation6 by disturbing the water balance in plants, homeostasis of Na+ and Cl− ions and nutrient uptake.Citation7
In order to cope with the excess ROS and maintain redox homeostasis, plants have evolved several adaptive mechanisms including highly efficient antioxidant enzymes, such as superoxide dismutase (SOD), ascorbate peroxidase (APX), glutathione reductase (GR) and dehydroascorbate reductase (DHAR) and also non-enzymatic antioxidants, such as ascorbate (AsA) and glutathione (GSH).Citation8 AsA and GSH are abundant metabolites participating in many protective mechanisms in leaves, and also react with different ROSCitation8,9 and its high efficiency is responsible for the alleviation of oxidative stress under abiotic stress.Citation10 In addition to its central task as an antioxidant, the role of GSH in plant metabolism concerns with the signaling of sulfur status, resistance to xenobiotics, heavy metal tolerance and pathogen response.Citation11,12 It also maintains redox homeostasis in cells.Citation13 The potential of GSH as antioxidant is related to the activity of glutathione reductase (GR), which catalyzes the regeneration of GSH from oxidized glutathione (GSSG). GSH has been shown to act as a signal molecule for S status of plantsCitation14 and is sensitive to ATP-sulfurylase (ATPS), the first enzyme in S-assimilatory pathway.Citation15 Sulfur assimilation pathway enzymes have been found to be involved in the regulation of GSH synthesis. Therefore, the activity of ATP-sulfurylase (ATPS) may influence GSH and Cys content in plant cell as it is a constituent of sulfur. In this context the mechanism of ion homeostasis, GSH synthesis and antioxidant enzymes are influenced by plant growth regulator under salt stress.
Salicylic acid (SA) is a phenolic compound, required in signal transduction cascades, involved in plant defense mechanisms in response to stress.Citation16 SA acts as a plant growth regulator and plays an important role in modulating the plant responses to abiotic stresses such as salinityCitation17,18, droughtCitation19 and heatCitation20 stress. Recently, Nazar et al.Citation17 reported that SA alleviated salt stress by improving photosynthetic characteristics and increased salt tolerance by inducing antioxidant metabolism in Vigna radiata. The roles of glutathione and GSH/thiol disulphide status in signal transduction cascade and modulation of phytohormones signaling pathways have been shown.Citation21 The amelioration of salt stress by SA has been reported, but the information on the effect of SA in the alleviation of salt stress by modulating the enzymes of AsA-GSH, S-assimilating enzyme and GSH content is not available. The objective of the research work undertaken was to test the hypothesis that SA protects photosynthesis through increase in the activity of AsA-GSH enzymes, ATPS activity and increased production of GSH content alleviates adverse effects of salt stress on photosynthesis and growth and increases antioxidant enzymes in mustard (Brassica juncea L.) cv. Pusa Jai Kisan. The results on SA effects were compared with the effects of biologically active SA analog, 2,6, dichloro-isonicotinic acid (INA) and exogenous GSH. The INA and GSH have been used in studies for confirming the effects of SA on GSH, respectively. The cultivar Pusa Jai Kisan has been shown to be a sulfur efficient type of mustard.Citation22
Results
Effect of SA on the accumulation of ions and oxidative stress
In order to assess the influence of SA in alleviation of salt-induced oxidative stress, we analyzed leaf Na+ and Cl− content and oxidative stress in terms of TBARS and H2O2 following treatment of 0.5 mM SA in presence or absence of 100 mM NaCl. The plants grown with NaCl showed higher leaf Na+ and Cl− content and oxidative stress in comparison to control (). Plant subjected to salt stress showed increased content of TBARS and H2O2. The content of TBARS and H2O2 in plants grown with salt increased by 2.8-times and 3.5-times, in comparison to their respective control (). Plants treated with 0.5 mM SA resulted in reduced leaf Na+ and Cl− content of plants grown with or without NaCl, but more conspicuously in plants grown with NaCl. SA treatment to salt stressed plants lowered Cl− content to half and reduced Na+ content to 36.1% compared to NaCl -treated plants.
Figure 1. Content of Na+, Cl−, TBARS and H2O2 in leaves of mustard (Brassica juncea L.) cv. Pusa Jai Kisan grown with 100 mM NaCl and treated with foliar 0.5 mM SA at 30 DAS. Data are presented as treatments mean ± SE (n = 4). Data followed by the same letter are not significantly different by LSD test at P < 0.05. TBARS; thiobarbituric acid reactive substances.
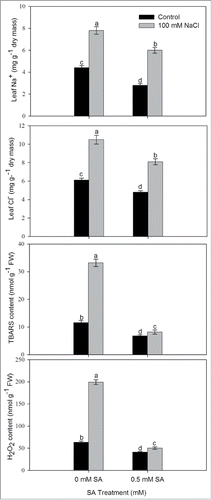
SA enhances antioxidant metabolism in plants under salt stress
To determine whether SA is involved in the alleviation of salt-induced oxidative stress through its effect on antioxidant metabolism, we examined the activity of antioxidant enzymes following treatment of 0.5 mM SA in presence or absence of 100 mM NaCl. The treatment of 100 mM NaCl increased the activity of antioxidant enzymes (DHAR, APX and GR) compared to the control plants (). Salt stress increased dehydroascorbate reductase (DHAR), ascorbate peroxidase (APX) and glutathione reductase(GR) by 29.5%, 216.7% and 78.9%, respectively compared to control. Application of 0.5 mM SA without NaCl resulted in increase in the activity of DHAR, APX and GR, respectively compared to control whereas, application of 0.5 mM SA plus NaCl maximally increased the activity of DHAR, APX and GR by 54.1%, 247.5% and 110.5%, respectively compared to control.
Figure 2. Activity of dehydroascorbate reductase, ascorbate reductase and glutathione reductase in leaves of mustard (Brassica juncea L.) cv Pusa Jai Kisan grown with 100 mM NaCl and treated with foliar 0.5 mM SA at 30 DAS. Data are presented as treatments mean ± SE (n = 4). Data followed by same letter are not significantly different by LSD test at P < 0.05.
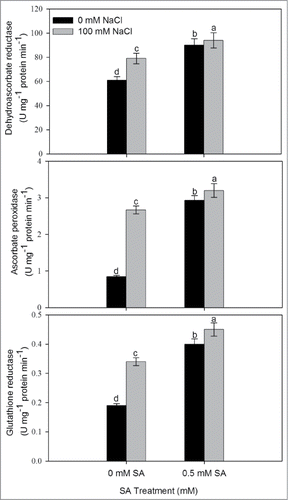
SA increases ATP-sulfurylase, SAT activity, and content of S and Cysteine
To assess the importance of SA in sulfur assimilation we analyzed activity of ATP-sulfurylase and SAT, and content of S and Cys. Salt stress increased the activity of ATPS and SAT and Cys content by 29.5%, 23.3% and 70.1%, respectively, whereas S content decreased by 32.1% compared to control. Application of 0.5 mM SA in presence of NaCl further increased the activity of ATPS and SAT, and content of Cys by 87.1%, 75.8% and 128.2%, respectively compared to control. Maximum S content was recorded with 0.5 mM SA under non-saline condition. Similarly, an increase of 62.6% in S content was noted with 0.5 mM SA under salt stress in comparison to control ().
Figure 3. Activity of ATP-S, SAT, S content and Cysteine content in leaves of mustard (Brassica juncea L.) cv. Pusa Jai Kisan grown with 100 mM NaCl and treated with foliar 0.5 mM SA at 30 DAS. Data are presented as treatments mean ± SE (n = 4). Data followed by same letter are not significantly different by LSD test at P < 0.05. ATP-S; ATP-sulfurylase, SAT; Serine acetyl transferase.
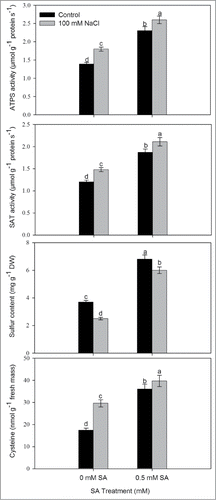
Response of SA on AsA–GSH pools and their redox status
The plants grown with NaCl resulted in decreased AsA content and increased DHA content in comparison to control. SA application at 0.5 mM resulted in increased AsA (reduced) content and AsA/DHA ratio by 5.2% and 49.6%, respectively, in comparison to control (). Similarly, content of GSH and GSSG also increased under salt stress. Supplementation of 0.5 mM SA further increased the reduced GSH by 34.0% and GSH/GSSG ratio by 28.0% in comparison to control. In contrast, GSSG was reduced as compared to control ().
Figure 4. Content of AsA, DHA, and ratio of AsA/DHA in leaves of mustard (Brassica juncea L.) cv Pusa Jai Kisan grown with 100 mM NaCl and treated with foliar 0.5 mM SA at 30 DAS. Data are presented as treatments mean ± SE (n = 4). Data followed by same letter are not significantly different by LSD test at P < 0.05. AsA; reduced ascorbate, DHA; dehydroascorbate.
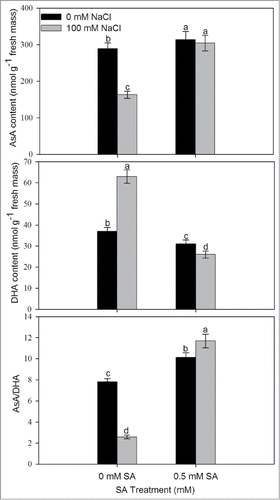
Figure 5. Content of GSH, GSSG, and ratio of GSH/GSSG in leaves of mustard (Brassica juncea L.) cv. Pusa Jai Kisan grown with 100 mM NaCl and treated with foliar 0.5 mM SA at 30 DAS. Data are presented as treatments mean ± SE (n = 4). Data followed by same letter are not significantly different by LSD test at P < 0.05. GSH; reduced glutathione, GSSG; oxidized glutathione.
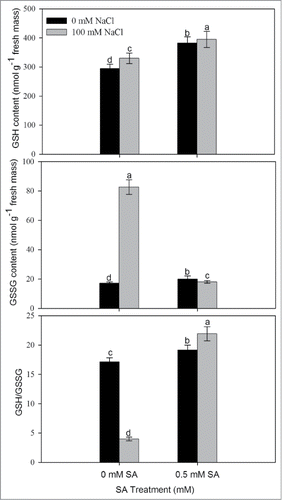
SA promotes photosynthetic and growth parameters under salt stress
Salt stress reduced maximal PS II photochemical efficiency, Rubisco activity and gas exchange parameters compared to control. Photosynthetic attributes were improved by the application of SA compared to control under no salt stress (). Application of 0.5 mM SA on NaCl-grown plants completely alleviated the NaCl effects and increased the maximal PS II photochemical efficiency, Rubisco activity and water-use efficiency (WUE) by 2.3%, 23.0% and 6.6%, respectively, compared to control. Salt stress significantly reduced net photosynthesis, stomatal conductance and intercellular CO2 concentration by 40.0%, 26.4% and 41.3%, respectively, compared to control. However, SA treatment to salt stressed plants reduced the negative effects on photosynthetic attributes, and SA application limited the decreases in the above characteristics to 22.1%, 19.2% and 24.9%, respectively, in comparison to control (). The growth characteristic, leaf area and plant dry mass decreased significantly under salt stress. In the presence of salt stress, the decrease in leaf area and plant dry mass was maximally overcome by 15.8% and 43.1% by the supplementation of SA.
Table 1. Maximal PSII photochemical efficiency, Rubisco (μmol CO2 mg−1 protein min−1), water-use efficiency (μmol mol−1), stomatal conductance (mmol CO2 m−2 s−1), intercellular CO2 concentration (μmol CO2 mol−1), net photosynthesis (μmol CO2 m−2 s−1), leaf area (cm2 plant−1) and plant dry mass (g plant−1) of mustard (Brassica juncea L) cv. Pusa Jai Kisan at 30 DAS. Plants were grown with/without salt stress and treated with foliar 0.5 mM SA. Data are presented as treatments mean ± SE (n = 4). Data followed by same letter are not significantly different by LSD test at P < 0.05
Comparative effect of SA, SA-analog (INA) and GSH on rubisco, photosynthesis and plant dry mass under salt stress
To substantiate the effects of SA in alleviation of the adverse effects of salt stress on photosynthesis and growth, we examined the effects of 0.5 mM SA-analog (INA) and 1 mM GSH given along with 100 mM NaCl and compared its efficacy in salt stress alleviation by 0.5 mM SA. It was observed that 0.5 mM INA and 1 mM GSH resulted in similar effects to SA treatment on the content of rubisco, photosynthesis and plant dry mass under no stress and salt stress condition as compared to control, indicating a relationship between GSH and SA in salt stress alleviation ().
Figure 6. Rubisco activity, photosynthetic rate and plant dry mass in mustard (Brassica juncea L.) cv Pusa Jai Kisan grown with 100 mM NaCl and treated with foliar 0.5 mM SA or INA or 1 mM GSH at 30 DAS. Data are presented as treatments mean ± SE (n = 4). Data followed by same letter are not significantly different by LSD test at P < 0.05.
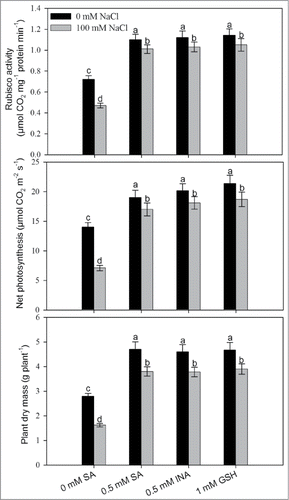
Discussion
The importance of phytohormones in augmenting productivity has been recognized since long. However, the recognition of salicylic acid as a plant growth regulator in sustainability of crop production has received attention in recent years. Hence, the aim of the reported research undertaken was to gain insight into the SA-induced mechanisms for protection of photosynthesis and growth under salt stress. In this study, it was observed that SA application promoted photosynthesis under no stress condition, and alleviated the negative effect of salt stress through enhancing the enzyme activity of AsA-GSH, sulfur assimilation enzymes and GSH synthesis.
Salt stress-induced reduction in photosynthesis has been linked with the changes in photosynthesizing tissue, toxicity of excessive Na+ and Cl− and reduction in translocation of assimilates to the sink and results in increasing the production of ROS leading to inhibition in photosynthetic and growth characteristics. Supplementation of 0.5 mM SA resulted in the protection of photosynthesis under salt stress which was associated with the decreased content of Na+ and Cl− ions. The reduced contents of Na+ in leaves indicated that Na+ was retained in the roots, perhaps by a mechanism involving regulation of water content and lowered the oxidative stress. The oxidative stress measured in terms of TBARS and H2O2 in salt-stressed plants, was found reduced by SA treatment. The results are consistent with the other studies showing that application of SA decreased Na+ concentration in roots and shoots of Zea mays seedlings and resulted in an increase of nutrients content.Citation23,24 Jayakannan et al.Citation25 have also shown that SA pretreatment in Arabidopsis did not decrease Na+ entry into roots, but reduced Na+ accumulation in the shoot. Application of SA has been shown to influence plant growth by promoting protective reactions involving photosynthetic pigments and membrane integrity.Citation26,27
Of the strategies capable of counteracting salt stress and its detoxification, the pivotal role of AsA–GSH pools and the coordination between AsA–GSH regenerating enzymes with other enzymatic antioxidants should be studied. Activities of enzymes in the ascorbate glutathione cycle are increased under salt stress suggesting a requirement for increased activity of the cycle under these conditions. Polle and RennenbergCitation28 reported that AsA acts as an efficient scavenger for oxidative compounds. Further, the capability of the AsA–GSH regenerating enzyme system comprising MDHAR, DHAR, and GR, and the maintenance of AsA, DHA, GSH, and GSSG poolsCitation29 may contribute to controlling salinity stress. In the present study, the induction of antioxidants mechanisms for the protection against ROS includes DHAR, APX and GR in response to salt stress which together helped in the scavenging of H2O2 into H2O and O2 in plantsCitation30 and subsequently resulted in GSH production on application of SA. These results imply that SA may provide protection against the oxidative stress by maintaining the redox balance. Both AsA and GSH are required in plants to maintain the intergrity of the photosynthetic membranes and an active AsA–GSH pathway under oxidative stress.Citation30,31,32 In addition, Kocsy et al.Citation33 suggested that the change of AsA/DHA and GSH/GSSG was more important in cell resistance against ROS compared with AsA or GSH content. Under salt stress decline in AsA/DHA and GSH/GSSG ratios was reversed by SA application. Hence, SA-treated plants, maintained elevated levels of AsA and GSH as well as high redox ratios of AsA/DHA and GSH/GSSG could be associated with salt tolerance and contributed to prevent oxidative injury in these plants. The increased rate of GSH (GSH/GSSG) was associated with the GR activity which was up regulated maximally under salt stress in SA-treated plants. The S-assimilatory pathway also regulates the synthesis of GSH and is connected to the enzymes of ascorbate-glutathione (AsA-GSH) pathway. Thus, S-assimilation regulated antioxidant system and helped in eliminating H2O2 and repairing cellular injury.Citation34 It has been reported that withdrawal of S from the medium leads to decreased levels of sulfate and GSH in Arabidopsis and canola plants tissues resulting in the induction of sulfate transporter systems and ATPS activity.Citation35,36 The inhibition in the activity of ATPS1 and APR results in the inhibition of GSH synthesis.Citation37,38,39 Supplementation of 0.5 mM SA results in the substantial increase in ATP sulfurylase, serine acetyl transferase activity and Cys content under salt stress and helps in reversing the effects of NaCl−induced ROS on photosynthesis. This might be due to SA application which helped to protect photosynthesis through increase in ascorbate-glutathione metabolism and sulfur assimilation enzymes, which would enhance the production of glutathione and increase antioxidant enzymes activity (). Freeman et al.Citation40 showed that elevation of free SA levels in Arabidopsis, both genetically and by exogenous feeding, enhanced the specific activity of serine acetyl transferase, leading to elevated glutathione and increased Ni resistance. Hacham et al.Citation41 studied that GSH might have a role in response to biotic stress by initiating defense responses and modifying plants' growth and development. The report on the effect of SA on the AsA-GSH metabolism and S assimilation enzymes in mustard under salt stress is not available in the literature. Khan et al.Citation42 have already reported that higher ATPS activity in mustard is necessary for the maintenance of optimal GSH levels required for the proper functioning of ascorbate–glutathione pathway. In our experiment, SA induces tolerance under salt stress and maintained the high intracellular redox status of AsA-GSH pools,which was tuned with a good coordination between AsA-GSH regenerating enzymes. Overall, the thiol-disulphide redox state serves as a marker of stress and cell viability, with important impacts upon the signaling mechanism during abiotic stress.Citation43 In sulfur-sufficient plants higher levels of thiol compounds were more able to remove the toxic effects of salt stress and were more tolerant.Citation44
Figure 7. Schematic representation showing SA application results in improved photosynthesis and growth under salt stress in mustard involving ascorbate-glutathione metabolism and S assimilation. AsA, ascorbate; APS, adenosine 5´-phosphosulfate; APX, ascorbate peroxidase; DHA, dehydroascorbate; DHAR, dehydroascorbate reductase; GR, glutathione reductase; GSH, reduced glutathione; GSSG, oxidised glutathione; MDHA, mono dehydroascorbate SA, salicylic acid; SO3−2, sulfite; S2−, sulfide.
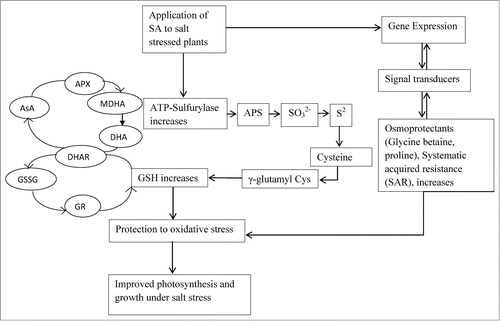
Photosynthesis is one of the most important processes and its efficiency is drastically reduced under salt stressCitation45 as shown by the inhibition of Fv/Fm and Rubisco. Miteva et al.Citation46 reported that salt stress reduced the rubisco level by approximately 80% in barley seedlings. Such a kind of salt stress might imply the effect of salt at the level of transcription, translation or gene regulation.Citation47 Arfan et al.Citation48 observed that foliar spray of SA might have affected certain metabolic factors in carbon uptake or fixation of Rubisco enzyme, and/or photosynthetic carbon reduction cycle. The increment in photosynthesis and plant growth by SA under salt stress is attributed to increased S-assimilation and higher allocation of N and S to Rubisco protein and increased availability of CO2 for Rubisco.This might be responsible for its favorable effect on plant growth and development. MakinoCitation49 established a positive relationship of leaf Rubisco with photosynthesis in most of the plants and became beneficial for the survival of plants under stressful conditions. Furthermore, it was observed that SA improved the photosynthetic attributes, PS II activity, water-use efficiency, plant dry mass and lead area.
Taken together, we infer that the higher tolerance of mustard was due to its lower content of Na+ and Cl− and oxidative stress and higher activity of AsA-GSH metabolism and S assimilation enzymes. These traits helped to reduce the oxidative stress and remove ROS efficiently. Conclusively, it may be said that application of 0.5 mM SA alleviated the adverse effects of salt stress and improved photosynthetic capacity by inducing the enzymes of AsA-GSH pathway, sulfur assimilation enzymes through meeting out the demand for GSH synthesis. Higher activity of S-assimilation enzymes together with GSH results in better antioxidative protection and adaptation against the salt stress which protected the photosynthetic apparatus and maintained photosynthessis.
Materials and Methods
Plant material and growth conditions
Mustard (Brassica juncea L.) cv Pusa Jai Kisan were raised from surface sterilized seeds and sown in 23-cm diameter earthen pots filled with acid-washed sand. Plants grown in pots were kept in a greenhouse under natural day/night conditions with photosynthetically active radiation (PAR>900 μmol m−2s−1 and average day/night temperature of 33/20 ± 2°C in the Department of Botany, Jamia Hamdard University, Delhi, India. Plants (2 per pot) were subjected to either 0 (control) or 100 mM NaCl. Pots were saturated daily with 300 mL of 100 mM NaCl in the form of modified full strength Hoagland's nutrient solution and the control group of plants were fed with 300 mL nutrient solution. Salicylic acid was dissolved in absolute ethanol then added drop wise to water (ethanol/water: 1/1000 v/v). In experiments, treatments of SA or/and SA analog (INA) at 0.5 mM concentration and 1 mM GSH were applied at 15d after sowing (DAS) on the foliage of 0 and 100 mM NaCl−grown plants. The concentration of SA was selected based on our earlier findings.Citation17 A control group of plants grown without NaCl and sprayed with ethanol/water: 1/1000 v/v was maintained. A surfactant teepol (0.5%) was added with the control and SA treatments solution. The volume of the spray was 25 mL per pot. The experiment followed a completely randomized block design and the number of replicates for each treatment was 4 (n = 4). Measurements were done at 30 DAS and care was taken to select same age of leaves for the determinations.
Estimation of content of ions, lipid peroxidation and H2O2 content
The content of Na+ and Cl− was determined in the digested plant samples using Tri acid mixture (TAM), which is a mixture of nitric acid, sulfuric acid and perchloric acid in the ratio of 10:5:4. The content of Na+ was estimated using flame photometer (Khera-391: Khera Instruments, New Delhi), whereas Cl− content was determined by titration against 0.02 N silver nitrate solution using 5% K2CrO4 as indicator.
The level of lipid peroxidation in leaves was determined by estimating the content of thiobarbituric acid reactive substances (TBARS) as described by Dhindsa et al.Citation50 Fresh leaf tissues (0.5 g) were ground in 0.25% 2-thiobarbituric acid in 10% trichloroacetic acid using mortar and pestle. After heating at 95°C for 30 min, the mixture was quickly cooled on ice bath and centrifuged at 10,000 × g for 10 min. The absorbance of the supernatant was read at 532 nm and corrected for non-specific turbidity by subtracting the absorbance of the same at 600 nm. The content of TBARS was calculated using the extinction coefficient (155 mM−1 cm−1).
The content of H2O2was determined following the method of Okuda et al.Citation51 Fresh leaf tissues (50 mg) were ground in an ice cold 200 mM perchloric acid. After centrifugation at 1200 × g for 10 min, perchloric acid of the supernatant was neutralized with 4 M KOH. The insoluble potassium perchlorate was eliminated by centrifugation at 500 × g for 3 min. The reaction was started by the addition of peroxidase and the increase in absorbance was recorded at 590 nm for 3 min.
Assay of antioxidant enzymes
Fresh leaf tissues (200 mg) were homogenized with an extraction buffer containing 0.05% (v/v) Triton X-100 and 1% (w/v) polyvinylpyrrolidone (PVP) in potassium-phosphate buffer (100 mM, pH 7.0) using chilled mortar and pestle. The homogenate was centrifuged at 15,000 × g for 20 min at 4°C. The supernatant obtained after centrifugation was used for the assay of glutathione reductase (GR; EC 1.6.4.2) enzyme. For the assay of ascorbate peroxidase (APX; EC 1.11.1.11), extraction buffer was supplemented with 2 mM ascorbate. DHAR activity was measured by the method of Nakano and AsadaCitation52 by the formation of ascorbate at 265 nm. The reaction mixture contained 25 mmol L−1 phosphate buffer (pH 7.0), 2.5 mmol L−1 reduced glutathione, 0.4 mmol L−1 dehydroascorbate, and the enzyme extract.
Activity of APX was determined by adopting the method of Nakano and AsadaCitation52 by recording the decrease in absorbance of ascorbate at 290 nm. The assay mixture contained phosphate buffer (50 mM, pH 7.0), 0.1 mM EDTA, 0.5 mM ascorbate, 0.1 mM H2O2 and the enzyme extract. Activity of APX was calculated by using the extinction coefficient 2.8 mM−1 cm−1. One unit of enzyme was the amount necessary to decompose 1 μmol of substrate per min at 25°C.
Activity of GR was determined by the method of Foyer and HalliwellCitation53 by monitoring the glutathione dependent oxidation of NADPH at 340 nm. The assay mixture contained phosphate buffer (25 mM, pH 7.8), 0.5 mM oxidized glutathione (GSSG), 0.2 mM NADPH and the enzyme extract. The activity of GR was calculated by using extinction coefficient 6.2 mM−1 cm−1. One unit of enzyme was the amount necessary to decompose 1 μmol of NADPH per min at 25°C.
Assay of ATPS, SAT, contents of S and Cys
Activity of ATP-sulfurylase (ATPS) was measured in leaves by the method of Lappartient and TouraineCitation35. Fresh leaf tissues (1 gm) was ground at 4°C in a buffer consisting of 10 mM Na2EDTA, 20 mM Tris-HCl (pH 8.0), 2 mM dithiothreitol (DTT), and 0.01 g mL−1 PVP, using 1:4 (w/v) tissue to buffer ratio. The homogenate was centrifuged at 20,000 × g for 10 min at 4°C. The supernatant was used for in vitro ATP-sulfurylase assay. The enzyme activity was measured using molybdate-dependent formation of pyrophosphate. The reaction was initiated by adding 0.1 mL of extract to 0.5 mL of the reaction mixture, which contained 7 mM MgCl2, 5 mM of Na2MoO4, 2 mM of Na2ATP, and 0.032 units mL−1 of sulfate-free inorganic pyrophosphate in 80 mM Tris-HCl buffer (pH 8.0). Another aliquot from the same extract was added to the same reaction mixture except that Na2MoO4 was absent. Incubations were carried out at 37°C for 15 min, after which phosphate was determined on a spectrophotometer.
Activity of SAT in the leaf extract was determined by the method of Kredich and Tomkins.Citation54 Fresh leaf tissues (0.5 g) were ground with a chilled mortar and pestle in 2 mL of ice cold extraction buffer Tris–HCl 100 mM (pH 8.0), 100 mM KCl, 20 mM MgCl2, 1% Tween 80, and 10 mM DTT. The samples were centrifuged at 12,000 g for 10 min at 4°C. The supernatant obtained was used for SAT assay. The enzyme reaction mixture contained 0.1 mM acetyl CoA, 50 mM Tris–HCl (pH 7.6), 1 mM 5′5′-dithiobis-2-nitrobenzoic acid (DTNB), 1 mM EDTA, and 1 mM L-serine in 1 mL. Subsequent to reaction initiation by addition of enzyme at 25°C, the rate was estimated by monitoring the increase in absorbance at 412 nm and calculated using an extinction coefficient of 13, 600. A blank containing all materials except L-Serine was run simultaneously and subtracted from the reaction rate obtained with L-serine.
Content of S was determined in leaf samples digested in a mixture of concentrated HNO3 and 60% HClO4 (85:1,v/v) using the turbidimetric method of Chesnin and Yien.Citation55
The content of cysteine in leaves was determined spectrophotometrically, adopting the method of Giatonde.Citation56 Fresh leaf (0.5 g) was homogenized in 5% (w/v) ice-cold perchloric acid. The final volume of 4 mL g−1 of plant tissue was used. The suspension was centrifuged at 2800 × g for 1 h at 5°C, and the supernatant was filtered through Whatman no. 30 paper. The filtrate (1 mL) was treated with acid ninhydrin reagent. The extinction was read at 580 nm, and the amount of cysteine was calculated with reference to a calibration curve obtained under similar conditions for standard cysteine.
Determination of AsA and GSH content
The method described by Law et al.Citation57 was employed for the determination of reduced (AsA) and oxidized (DHA). The assay was based on the reduction of Fe3+ to Fe2+ by AsA in acidic solution. The Fe2+ forms complex with bipyridal, giving pink color that absorbs at 525 nm. Total AsA was determined through reduction of DHA to AsA by dithiothreitol.
Glutathione was estimated following the method of Anderson.Citation58 Fresh leaf (0.5 g) was homogenized in 2 mL of 5% (w/v) sulfosalicylic acid under cold conditions. The homogenate was centrifuged at 10,000 × g for 10 min. To 0.5 mL of the supernatant, 0.6 mL of 100 mM (pH 7.0) phosphate buffer and 40 μL of DTNB were added. After 2 min, the absorbance was read at 412 nm with a UV-vis spectrophotometer. GSSG was determined in presence of 2-vinylpyridine and GSH content was calculated as the difference between total glutathione and GSSG.
Photosynthesis and growth attributes
The maximal PS II photochemical efficiency (Fv/Fm) of the fully expanded second leaf from top of plant was determined with the help of chlorophyll fluorometer (OS-30p; OptiSciences, Inc., Hudson, USA).
Rubisco activity was determined spectrophotometrically by adopting the method of UsudaCitation59 by monitoring NADH oxidation at 30°C at 340 nm during the conversion of 3- phosphoglycerate to glycerol 3-phosphate after the addition of enzyme extract to the assay medium. For enzyme extraction, leaf tissue (1.0 g) was homogenized using a chilled mortar and pestle with ice-cold extraction buffer containing 0.25 M Tris–HCl (pH 7.8), 0.05 M MgCl2, 0.0025 M EDTA and 37.5 mg DTT. The homogenate was centrifuged at 4°C at 10,000 × g for 10 min. The resulting supernatant was used to assay the enzyme. The reaction mixture contained 100 mM Tris–HCl (pH 8.0), 40 mM NaHCO3, 10 mM MgCl2, 0.2 mM NADH, 4 mM ATP, 5 mM DTT, 1 U of glyceraldehydes 3-phosphodehydrogenase and 1 U of 3-phosphoglycerate kinase and 0.2 mM ribulose 1,5-bisphosphate (RuBP). Protein was estimated according to BradfordCitation60 using bovine serum albumin as standard. Water-use efficiency (WUE) was calculated as the ratio of photosynthesis to stomatal conductance to avoid effects of small differences in vapor pressure between measurementsCitation61.
Stomatal conductance (gs) and intercellular CO2 concentration (Ci) and net photosynthesis (Pn), were measured in fully expanded uppermost leaves of plants in each treatment using infra red gas analyzer (CI-340, Photosynthesis System, BioScience, USA). The measurements were done between 11.00 and 12.00 h at light saturating intensity and at 370 ± 5 μmol mol−1 atmospheric CO2 concentrations.
Plants were uprooted carefully from the pots, washed to remove dust. Leaf area was measured with a leaf area meter (LA 211, Systronics, New Delhi, India). Dry mass of plants was recorded after drying the sample in a hot air oven at 80°C till constant weight.
Statistical analysis
Data were statistically analyzed using analysis of variance (ANOVA) by SPSS statistics (ver.17.0), and presented as treatment mean ± SE (n = 4). Least significant difference (LSD) was calculated for the significant data at P < 0.05. Bars showing the same letter are not significantly different by LSD test at P < 0.05.
Disclosure of Potential Conflicts of Interest
No potential conflicts of interest were disclosed.
Acknowledgment
The authors are thankful the Head, Department of Botany, Jamia Hamdard University, New Delhi for providing research facilities.
Funding
The first author is thankful to the University Grant Commission, New Delhi, for funding in the form of Dr. D.S. Kothari Postdoctoral Fellowship (File No. F.4-2/2006(BSR)/13-711/2012(BSR)).
References
- Tester M, Davenport R. Na+ tolerance and Na+ transport in higher plants. Ann Bot 2003; 91:503-27; PMID:12646496; http://dx.doi.org/10.1093/aob/mcg058
- Munns R. Comparative physiology of salt and water stress. Plant Cell Environ 2002; 25:239-50; PMID:11841667; http://dx.doi.org/10.1046/j.0016-8025.2001.00808.x
- Wang X, Fan P, Song H, Chen X, Li X, Li Y. Comparative proteomic analysis of differentially expressed proteins in shoots of Salicornia europaea under different salinity. J Proteome Res 2009; 8:3331-45; PMID:19445527; http://dx.doi.org/10.1021/pr801083a
- Halliwell B, Gutteridge JMC. Free Radicals in Biology and Medicine. Clarendon, Oxford, 1985
- Turan S, Tripathy BC. Salt and genotype impact on antioxidative enzymes and lipid peroxidation in two rice cultivars during de-etiolation. Protoplasma 2013; 250:209-22; PMID:22434153; http://dx.doi.org/10.1007/s00709-012-0395-5
- Steduto P, Albrizio R, Giorio P, Sorrentino G. Gas exchange response and stomatal and non-stomatal limitations to carbon assimilation of sunflower under salinity. Environ Exp Bot 2000; 44:243-55; PMID:11064044; http://dx.doi.org/10.1016/S0098-8472(00)00071-X
- Munns R, Tester M. Mechanisms of salinity tolerance. Annu Rev Plant Biol 2008; 9:651-81; http://dx.doi.org/10.1146/annurev.arplant.59.032607.092911
- Noctor G, Arisi ACM, Jouanin L, Kunert KJ, Rennenberg H, Foyer CH. Glutathione: biosynthesis, metabolism, and relationship to stress tolerance explored in transformed plants. J Exp Bot 1998; 49:623-47
- Kumar B, Singla-Pareek SL, Sopory SK. Glutathione homeostasis: crucial for abiotic stress tolerance in plants. In: Pareek A, Sopory SK, Bohnert HJ, Govindjee, ed. Abiotic Stress Adaptation in Plants: Physiological, Molecular and Genomics Foundation. The Netherlands: Springer, 2010:263-82
- Asada K. Production and scavenging of reactive oxygen species in chloroplasts and their functions. J Plant Physiol 2006; 141:391-96; http://dx.doi.org/10.1104/pp.106.082040
- Tausz M, Sircelj H, Grill D. The glutathione system as a stress marker in plant ecophysiology: is a stress-response concept valid? J Exp Bot 2004; 55:1955-62; PMID:15234995; http://dx.doi.org/10.1093/jxb/erh194
- Han Y, Mhamdi A, Chaouch S, Noctor G. Regulation of basal and oxidative stress triggered jasmonic acid-related gene expression by glutathione. Plant Cell Environ 2013; 36:1135-46; PMID:23210597; http://dx.doi.org/10.1111/pce.12048
- Szalai G, Kellos T, Galiba G, Kocsy G. Glutathione as an antioxidant and regulatory molecule in plants under abiotic stress conditions. J Plant Growth Regul 2009; 28:66-80; http://dx.doi.org/10.1007/s00344-008-9075-2
- Kopriva S, Rennenberg H. Control of sulphate assimilation and glutathione synthesis: interaction with N and C metabolism. J Exp Bot 2004; 55:1831-42; PMID:15286142; http://dx.doi.org/10.1093/jxb/erh203
- Yi H, Galant A, Ravilious G, Preuss M, Jez J. Sensing sulfur conditions: simple to complex protein regulatory mechanisms in plant thiol metabolism. Mol Plant 2010; 3:269-79; PMID:20080815; http://dx.doi.org/10.1093/mp/ssp112
- Bartoli CG, Casalongué CA, Simontacchi M, Marquez-Garcia B, Foyer CH. Interactions between hormone and redox signalling pathways in the control of growth and cross tolerance to stress. Environ Exp Bot 2013; 94:73-88; http://dx.doi.org/10.1016/j.envexpbot.2012.05.003
- Nazar R, Iqbal N, Syeed S, Khan NA. Salicylic acid alleviates decreases in photosynthesis under salt stress by enhancing nitrogen and sulfur assimilation and antioxidant metabolism differentially in two mungbean cultivars. J Plant Physiol 2011; 168:807-15; PMID:21112120; http://dx.doi.org/10.1016/j.jplph.2010.11.001
- Li G, Peng X, Wei L, Kang G. Salicylic acid increases the contents of glutathione and ascorbate and temporally regulates the related gene expression in salt-stressed wheat seedlings. Gene 2013; 529:321-25; PMID:23948081; http://dx.doi.org/10.1016/j.gene.2013.07.093
- Yazdanpanah S, Baghizadeh A, Abbassi F. The interaction between drought stress and salicylic and ascorbic acids on some biochemical characteristics of Satureja hortensis. Afr J Agr 2011; 6:798-807
- Khan MIR, Iqbal N, Masood A, Per TS, Khan NA. Salicylic acid alleviates adverse effects of heat stress on photosynthesis through changes in proline production and ethylene formation. Plant Signal Behav 2013; 8:263-74; http://dx.doi.org/10.4161/psb.22478
- Noctor G, Mhamdi A, Chaouch S, Han Y, Neukermans J, Marquez-Garcia B, Queval G, Foyer CH. Glutathione in plants: an integrated overview. Plant Cell Environ 2012; 35:454-84; http://dx.doi.org/10.1111/j.1365-3040.2011.02400.x
- Nazar R, Khan NA, Anjum NA. ATP-sulfurylase activity, photosynthesis, and shoot dry mass of mustard (Brassica juncea L.) cultivars differing in sulfur accumulation capacity. Phosynth 2008; 46:279-82
- Gunes A, Inal A, Alpaslan M, Cicek N, Guneri E, Eraslan F, Guzelordu T. Effects of exogenously applied salicylic acid on the induction of multiple stress tolerance and mineral nutrition in maize (Zea mays L.). Arch Agron Soil Sci 2005; 51:687-95; http://dx.doi.org/10.1080/03650340500336075
- Gunes A, Inal A, Alpaslan M, Eraslan F, Bagci EG, Cicek N. Salicylic acid induced changes on some physiological parameters symptomatic for oxidative stress and mineral nutrition in maize (Zea mays L.) grown under salinity. J Plant Physiol 2007; 164:728-36; PMID:16690163; http://dx.doi.org/10.1016/j.jplph.2005.12.009
- Jayakannan M, Bose J, Babourina O, Rengel Z, Shabala S. Salicylic acid improves salinity tolerance in Arabidopsis by restoring membrane potential and preventing salt-induced K+ loss via a GORK channel. J Exp Bot 2013; 64:2255-68; PMID:23580750; http://dx.doi.org/10.1093/jxb/ert085
- El-Tayeb MA. Response of barley grains to the interactive effect of salinity and salicylic acid. Plant Growth Regul 2005; 45:215-24; http://dx.doi.org/10.1007/s10725-005-4928-1
- Miura K, Tada Y. Regulation of water, salinity, and cold stress responses by salicylic acid. Front Plant Sci 2014; 5:1-12; http://dx.doi.org/10.3389/fpls.2014.00004
- Polle A, Rennenberg H. Significance of antioxidants in plant adaptation to environmental stress. In: Fowden L, Mansfield T, Stoddart J, ed. Plant Adaptation to Environmental Stress. London: Chapman and Hall, 1993:264-73
- Hossain MA, Ismail MR, Kamal Uddin M, Islam MZ, Ashrafuzzaman M. Efficacy of ascorbate-glutathione cycle for scavenging H2O2 in two contrasting rice genotypes during salinity stress. Aust J Crop Sci 2013; 7:1801-08.
- Munné-Bosch S, Alegre L. The function of tocopherols and tocotrienols in plants. Crit Rev Plant Sci 2002; 21:31-57; http://dx.doi.org/10.1080/0735-260291044179
- Noctor G, Foyer CH, Ascorbate and glutathione: keeping active oxygen under control. Annu Rev Plant Physiol Plant Mol Biol 1998; 49:249-729; PMID:15012235; http://dx.doi.org/10.1146/annurev.arplant.49.1.249
- Anjum NA, Umar S, Ahmad A, Iqbal M, Khan NA. Sulphur protects mustard (Brassica campestris L.) from cadmium toxicity by improving leaf ascorbate and glutathione. Plant Growth Regul 2008; 54:271-79; http://dx.doi.org/10.1007/s10725-007-9251-6
- Kocsy G, Galiba G, Brunold C. Role of glutathione in adaptation and signaling during chilling and cold acclimation in plants. Plant Physiol 2001; 113:158-64; http://dx.doi.org/10.1034/j.1399-3054.2001.1130202.x
- Han, Y, Chaouch S, Mhamdi A, Queval G, Zechmann B, Noctor G. Arabidopsis mutants points to novel roles for glutathione in coupling H2O2 to activation of salicylic acid accumulation and signaling. Antioxid Redox Signal 2013; 18:2106-121; http://dx.doi.org/10.1089/ars.2012.5052
- Lappartient AG, Touraine B. Demand-driven control of root ATP sulfurylase activity and SO42− uptake in intact canola. Plant Physiol 1996; 111:147-57; PMID:12226281
- Lappartient AG, Vidmar JJ, Leustek T, Glass AD, Touraine B. Inter-organ signaling in plants: regulation of ATP sulfurylase and sulfate transporter genes expression in roots mediated by phloem-translocated compound. Plant J 1999; 18:89-95; PMID:10341446; http://dx.doi.org/10.1046/j.1365-313X.1999.00416.x
- Buchner P, Stuiver CEE, Westerman S, Wirtz M, Hell R, Hawkesford MJ, De Kok LJ. Regulation of sulfate uptake and expression of sulfate transporter genes in Brassica oleracea as affected by atmospheric H2S and pedospheric sulfate nutrition. Plant Physiol 2004; 136:3396-408; PMID:15377780; http://dx.doi.org/10.1104/pp.104.046441
- Herschbach C, Rennenberg H. Influence of glutathione (GSH) on net uptake of sulfate and sulfate transport in tobacco plants. J Exp Bot 1994; 45:1069-76; http://dx.doi.org/10.1093/jxb/45.8.1069
- Vauclare P, Kopriva S, Fell D, Suter M, Sticher L, von Ballmoos P, Krähenbühl U, Op den Camp R, Brunold B. Flux control of sulphate assimilation in Arabidopsis thaliana: adenosine 5'-phosphosulphate reductase is more susceptible than ATP sulphurylase to negative control by thiols. Plant J 2002; 31:729-40; PMID:12220264; http://dx.doi.org/10.1046/j.1365-313X.2002.01391.x
- Freeman JL, Garcia D, Kim D, Hopf A, Salt DE. Constitutively elevated salicylic acid signals glutathione mediated nickel tolerance in Thlaspi nickel hyperaccumulators. Plant Physiol 2005; 137:1082-91; PMID:15734913; http://dx.doi.org/10.1104/pp.104.055293
- Hacham Y, Koussevitzky S, Kirma M, Amir R. Glutathione application affects the transcript profile of genes in Arabidopsis seedlings. J Plant Physiol 2014; 171:1444-51; PMID:25077999; http://dx.doi.org/10.1016/j.jplph.2014.06.016
- Khan NA, Nazar R, Anjum NA. Growth, photosynthesis and antioxidant metabolism in mustard (Brassica juncea L.) cultivars differing in ATP-sulfurylase activity under salinity stress. Sci Hort 2009; 122:455-60; http://dx.doi.org/10.1016/j.scienta.2009.05.020
- Suzuki N, Koussevitzky S, Mittler R, Miller G. ROS and redox signal ling in the response of plants to abiotic stress. Plant Cell Environ 2012; 35:259-70; PMID:21486305; http://dx.doi.org/10.1111/j.1365-3040.2011.02336.x
- Astolfi S, Zuchi S. Adequate S supply protects barley plants from adverse effects of salinity stress by increasing thiol contents. Acta Physiol Plant 2013; 35:175-81; http://dx.doi.org/10.1007/s11738-012-1060-5
- Chaves MM, Costa JM, Madeira-Sabo NJ. Recent advances in photosynthesis under drought and salinity. Adv Bot Res 2011; 57:49-104; http://dx.doi.org/10.1016/B978-0-12-387692-8.00003-5
- Miteva TS, Zhelev NZh, Popova LP. Effect of salinity on the synthesis of ribulose-1,5-bisphosphate carboxylase/oxygenase in barley leaves. J Plant Physiol 1992; 140:46-51; http://dx.doi.org/10.1016/S0176-1617(11)81055-2
- Ziska LH, Seemann JR, DeJong TM. Salinity induced limitation ns on photosynthesis in Prunus salicina, a deciduous tree species. Plant Physiol 1990; 93:864-70; PMID:16667594; http://dx.doi.org/10.1104/pp.93.3.864
- Arfan M, Athar HR Ashraf M. Does exogenous application of salicylic acid through the rooting medium modulate growth and photosynthetic capacity in two differently adapted spring wheat cultivars under salt stress? J Plant Physiol 2007; 164:685-94; PMID:16884826; http://dx.doi.org/10.1016/j.jplph.2006.05.010
- Makino A. Photosynthesis, grain yield, and nitrogen utilization in rice and wheat. Plant Physiol 2011; 155:125-9; PMID:20959423; http://dx.doi.org/10.1104/pp.110.165076
- Dhindsa RH, Plumb-Dhindsa P, Thorpe TA. Leaf senescence correlated with increased level of membrane permeability, lipid peroxidation and decreased level of SOD and CAT. J Exp Bot 1981; 32:93-101; http://dx.doi.org/10.1093/jxb/32.1.93
- Okuda T, Masuda Y, Yamanka A, Sagisaka S. Abrupt increase in the level of hydrogen peroxide in leaves of winter wheat is caused by cold treatment. Plant Physiol 1991; 97:1265-7; PMID:16668520; http://dx.doi.org/10.1104/pp.97.3.1265
- Nakano Y, Asada K. Hydrogen peroxide is scavenged by ascorbate specific peroxidase in spinach chloroplasts. Plant Cell Physiol 1981; 22:867-80
- Foyer CH, Halliwell B. The presence of glutathione and glutathione reductase in chloroplasts: a proposed role in ascorbic acid metabolism. Planta 1976; 133:21-25; PMID:24425174; http://dx.doi.org/10.1007/BF00386001
- Kredich NM, Tomkins GM. The enzymic synthesis of L-cysteine in Escherichia coli and Salmonella typhimurium. J Biol Chem 1966; 21:4955-65
- Chesnin L, Yien CH. Turbidimetric determination of available sulphates. Soil Sci Soc Am Proc 1950; 15:149-51; http://dx.doi.org/10.2136/sssaj1951.036159950015000C0032x
- Giatonde MK. A spectrophotometric method for the direct determination of cysteine in the presence of other naturally occurring amino acids. Biochem J 1967; 104:627-33; PMID:6048802
- Law ME, Charles SA, Halliwell B. Glutathione and ascorbic acid in spinach (Spinacia oleracea) chloroplasts: The effect of hydrogen peroxide and of paraquat. Biochem J 1983; 210:899-903; PMID:6307273
- Anderson ME. Determination of glutathione and glutathione disulfides in biological sample. Methods Enzymol 1985; 113:548-70; PMID:4088074; http://dx.doi.org/10.1016/S0076-6879(85)13073-9
- Usuda H. The activation state of ribulose 1,5-bisphosphate carboxylase in maize leaves in dark and light. Plant Cell Physiol 1985; 26:1455-63
- Bradford MM. A rapid and sensitive method for the quantitation of microgram quantities of proteins utilising the principle of protein–dye binding. Anal Biochem 1976; 72:248-54; PMID:942051; http://dx.doi.org/10.1016/0003-2697(76)90527-3
- Von Cammerer S, Farquhar GD. Some relationship between the biochemistry of photosynthesis and the gas exchange of leaves. Planta 1981; 153:376-87; PMID:24276943; http://dx.doi.org/10.1007/BF00384257