Abstract
Sensory integration is vital for motile organisms constantly exposed to changing surroundings. Chlamydomonas reinhardtii is a single-celled green alga found swimming in freshwater. In this type of alga, sensory input is first detected by membrane receptors located in the cell body, and then transduced to the beating cilia by membrane depolarization. Many components of the machinery associated with sensory integration in C. reinhardtii, such as chemoreceptors and repolarization-associated channels, are yet uncharacterized. TRP channels are known mediators for cellular sensing in animal cells and it has been suggested that the C. reinhardtii genome encodes for a set of TRP proteins. Here, by combining behavioral studies with electrophysiological experiments conducted on both population and single alga, we test whether TRP channel blockers affect algal swimming behavior. Our results suggest that a TRP conductance is associated to the repolarization that follows a depolarizing receptor potential, highlighting a primitive function of TRP proteins.
The TRP channel family are calcium permeable, non-selective cation channels. Thus, TRP channel opening allows for the fast entrance of sodium and calcium ions into the cell. Originally described in Drosophila melanogaster,Citation1,2 at present the 28 genes encoding for mammalian TRP channels are the most studied because of their role in sensory and pain physiology.Citation3-5 Commonly found in soil and freshwater, C. reinhardtii is a single-celled chlorophyte alga approximately 10 µm in diameter, having 2 beating flagella allowing them to swim using a breast-stroke-type motion.Citation6,7 Navigating at about 50 μm/s, they rapidly integrate multiple external stimuli to control their orientation relative to the source of the signal. After the release of the Chlamydomonas reinhardtii genome sequence,Citation8 several ion channels, including TRP channels, have been reported as probable gene products. Since C. reinhardtii possesses a fine-tuned navigation system based on calcium conductances,Citation9 the presence of putative TRP channel coding sequences in the C. reinhardtii genome make them good candidates for both the generation of the input signal and/or the control of the propagation of the sensory input (). The expression of TRP transcripts has been knocked down in C. reinhardtii by means of RNA interference (RNAi) techniques, reporting behavioral impairments. Both located at the cilia, Cr-TRPP2 participates in flagella-dependent mating behavior,Citation10 and Cr-TRP11 seems to be part of the mechanosensory response circuit.Citation11 Recently, Arias-Darraz and colleagues have cloned Cr-TRP1,Citation12 one of the most primitive versions of a TRP channel, demonstrating that the conservation of structural and functional features can be traced back early in eukaryote evolution. Given the importance of TRP channels in cellular sensing within the animal kingdom, we want to investigate their general role in the behavior of the algae. Thus, we tested the effect of known TRP channel blockers on the capacity of Chlamydomonas to swim and their ability to respond to light or chemoattractants.
Figure 1. The effect of non-specific TRP channel blockers on Chlamydomonas reinhardtii response to light. (A) Scheme depicting different conductances present in Chlamydomonas. The molecular origin of several important conductances is unknown. (B) Representative recordings of light-induced ionic current taken from a population of swimming algae. The total photo-activated current drops by 80% upon incubation with 500 μM La3+. (C) Plot summarizing 5 experiments on algae population. Lanthanum induced a significant reduction in the total current (n = 5; 100 μM P < 0.05; 500 μM P < 0.01). A single asterisk indicates a statistically significant result, P < 0.05, while a double asterisk signifies P < 0.01. (D) TRP channel blockers affect the distribution of swimming modes observed in the algae (n = 36, summarizing 2673 trajectories). Control is represented by orange bars. I = immobile; S = sinusoidal; H = helical; E = erratic. There is a significant augment in the amount of immobile algae and a reduction in erratic motion after incubation with 50 µM 2-APB (n = 12; P < 0.05 (I); P < 0.01 (E)). 500 µM RR induced a significant change in the helical-to-sinusoidal switch (n = 8; P < 0.05). (E) Mean velocity and diffusion coefficient, calculated from single alga tracking, were not significantly affected by TRP channel blockers (n = 5, 602 trajectories). (F) Bar plot showing significant alterations of the phototactic behavior, measured as the capacity of cells to swim toward low intensity light (n = 5; P < 0.05). (G) Ruthenium red (1 mM) and 2-APB (50 µM) alter the photophobic response of swimming algae. 2 × 106 algal cells were placed in 35 mm petri dishes and pictured after 20 minutes of continuous light stimulation. The pictures correspond to representative results, and polar graphs next to the pictures correspond to the averaged position of the algae (yellow shades) after light treatment. An abnormal distribution of the algae was observed when incubated with the drugs. Each polar graph was built by averaging 4 polar graphs from 5 individual experiments. Error bars correspond to SE.
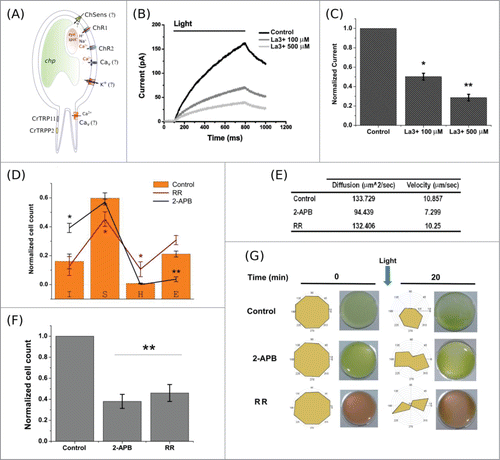
In absence of specific pharmacological tools for TRP channels, the acute use of non-specific compounds known to affect TRP channel function have provided valuable insights.Citation3,13,14 Therefore, we first tested whether the non-selective cationic channel blockers lanthanum (La3+) and ruthenium red (RR), together with 2-aminoethoxydiphenyl borate (2-APB), a non-selective TRP channel modulator (activator of TRPA1, TRPM6 and TRPVs; inhibitor of TRPC4, TRPC5, TRPC6, TRPM2, and TRPM8),Citation3 could affect algal response to light. It has been described that a photo-activated current can be recorded from intact algae populations.Citation15 Using this so-called “population method,” we observed that up to 80% of the total photo-activated current obtained from a chamber containing 1 × 106 swimming algae was blocked by incubation with 500 μM La3+ (). In darkness, C. reinhardtii swims in a preferential helical (H) mode, however, when exposed to light it switches to a sinusoidal (S) swimming mode.Citation7,16 We observed that the modes of motion of individual alga were affected by the non-specific TRP channel blockers (). While the switch between helical and sinusoidal mode was affected by 500 μM RR treatment, 50 μM 2-APB significantly increased the number of immobile (I) algae, and significantly reduced the number of erratic (E) algae, leaving the proportion of sinusoidal and helical moving cells intact. Interestingly, algae incubated with RR show a decrease in the amount of sinusoidal swimming, and an increase in the amount of helical swimming (, red line). Notably, motion intrinsic parameters such as the diffusion coefficient (D) and velocity were not greatly affected under any condition ().
Chlamydomonas perceive light using Channelrhodopsins (ChRs), and elicit either phototactic or photophobic behavior when exposed to light.Citation6,9,17 In a simple experiment, we used low-light radiation (white light; 1 × 103 erg/cm2/s−1) to induce phototaxis, observed as the accumulation of algal cells in the microscope field of view after 2 minutes of constant illumination. When quantified, we observed that this phototactic swimming was impaired by either 1 mM RR or 50 μM 2-APB treatments (). On the other hand, under intense light irradiation these algae display a well described photophobic behavior.Citation6,9 To induce the photophobic response, light (white light; 2 × 104 erg/cm2/s−1) was focused on one side of petri dishes containing free swimming cells. When the algae distribution within the petri dish was quantified using polar graphs, we observed that the treated algae were able to swim, however, their orientation during the photophobic response was modified by both 2-APB and RR (). In summary, compounds known to modulate TRP channel activity have a macroscopic effect on behavioral and functional responses of Chlamydomonas without affecting algal swimming capabilities, which rely mostly on ciliary signaling.Citation9
There are known limitations associated to pharmacological isolation of TRP channel activity. Often the drugs used are not specific enough to discriminate the non-selective TRP channel conductance from others.Citation3,14 However, some compounds have shown to be effective at discriminating TRP channel activity from other voltage-activated conductances such as Cav, Nav, and Kv among others. This is the case of N-(4-tert.butyl-phenyl)-4-(3-chloropyridin-2-yl) tetrahydropyrazine-1(2H)-carboxamide (BCTC), initially described as an effective blocker capable of differentiating TRPV1 channels from many other common ion channels and receptors,Citation18 was later found to also effectively block TRPM8 activity.Citation19 Moreover, structural analysis suggests that there is a conserved set of drug-channel interactions that might be preserved in members of TRPV and TRPM families in which most members participate in sensory-related activity.Citation14,20 Thus, because of its ability to inhibit TRP channel activity in a more specific fashion, we used BCTC as a finer pharmacological approach to look for alterations in algal swimming patterns. Incubations with BCTC (5 µM) elicit a significant loss in the amount of sinusoidal swimming cells, and a corresponding modification to the helical-to-sinusoidal switch (). This result is in contrast with the broader changes on swimming behavior caused by the TRP-channel modulator and calcium channel blocker 2-APB, or the more general calcium channel blocker ruthenium red, suggesting that BCTC blocks, as expected, a subset of TRP-like channels in the algae. Electrophysiological recordings taken from free swimming algae incubated with 5 µM BCTC (1 × 106 swimming algae) showed a significant reduction in the total current induced by continuous light stimulation (). The inhibition observed was smaller than the response detected previously for the case of 500 µM La3+, suggesting a more general effect of lanthanum over Chlamydomonas' conductances. In fact, the trivalent cations La3+ and Gd3+ were reported as blockers of ChRs and mechanosensitive channels in Chlamydomonas r.Citation21,22
We observed that BCTC did not cause a delay in development of the current (, inset), suggesting that the treatment does not affect the photosensory machinery (i.e. ChR response). To confirm this, we performed whole-cell patch clamp experiments on HEK-293T cells expressing ChR2 channels. No differences were observed upon light stimulation in the absence or presence of BCTC (30 µM; , inset). It is important to consider that the signal obtained from the population assay can be affected in 2 different ways: by the ability of the algae to trigger an action potential through activation of Channelrhodopsins, or by the ability to further propagate depolarizing potentials allowing the algae to align themselves toward the light during the pre-pulse stimulation (see Methods). Thus, we reasoned that although the algae are still able to respond to light, BCTC treatments might affect efficient algae orientation toward its target. Algal orientation toward light relies on the fact that the pseudo-eye structure, a rudimentary light-sensing organelle, is located on one side of the alga,Citation6,9 and consequently this natural asymmetry allows differential stimulation of the cilia by the depolarizing potential. We then explored the effect of BCTC on the light-dependent depolarization-repolarization cycle of the algae using loose-patch experiments to directly record membrane depolarization in algae subjected to light activation.Citation23,24 100 µs light pulses () evoked a rapid depolarization in the algal membrane with a typical decay time constant of 1.34 ± 0.2 ms. Incubations with 1 µM BCTC were enough to slow down the repolarization process (2.43 ± 0.17 ms; ). Under the assumption that the membrane can be modeled as a simple resistor-capacitor (RC) circuit, the increase in the repolarization time constant means that the BCTC-sensitive conductance represents half of the total conductance that contributes to the light-induced current. Under physiological conditions (i.e., low-sodium freshwater), Ca2+ and K+ are the major contributors to the voltage changes observed in the Chlamydomonas plasma membrane. While depolarization in freshwater is mainly driven by Ca2+ influx, a K+ efflux repolarizes the algal membrane toward the K+ equilibrium potential (EK).Citation9 An association between light- and chemosensory pathways has been reported in the past,Citation25,26 and there is experimental evidence suggesting that the potassium conductance linking these pathways corresponds to a non-selective cation conductance.Citation25-27 Thus, it would not be unreasonable to hypothesize that the BCTC-sensitive TRP conductance reported here is located downstream of the sensory input (e.g. ChR activation). Following this line of reasoning, we may anticipate that other sensory-dependent responses should converge on this conductance during the repolarization phase that follows any sensory-dependent depolarization controlling ciliary beating. To test this hypothesis, we decided to evaluate the capacity of freely swimming Chlamydomonas to keep progressive ordered movement toward a chemoattractant (tryptone) gradient. shows the coincidental effect of BCTC on the stimuli-dependent reorientation of the algae under both conditions, photo- and chemostimulation. Thus, our results show that the BCTC-sensitive conductance is required for efficient algal orientation in response to differential stimuli.
A proteome-wide search for putative TRP channel coding sequences in Chlamydomonas reinhardtii identified 21 protein candidates, including the experimentally characterized Cr-TRP1, Cr-TRP11, and Cr-TRPP2. This is in contrast to a previous study reporting 8 TRP channels,Citation11 and a recent study by our group reporting 10 TRP channels.Citation12 In the case of the latter, different assessment criteria with more stringent parameters were used to select for TRP channels. Reciprocal BLAST analysis of the 21 putative TRP channels indicates that Chlamydomonas contains TRP channels related to members of the TRPM and TRPV subfamilies: Chlamydomonas TRP5, TRP7, TRP11, TRP13, TRP16, TRP21, TRP22, TRP26, TRP27, and TRP28. In particular, our analysis suggests that TRP5 and TRP16 potentially play roles in carrying the BCTC-sensitive conductance, since they share conserved sequence motifs with TRPV1. Interestingly, TRP1 current is blocked by BCTC (IC50 = 1.03 µM).Citation12 Phylogenetic reconstruction of the TRP channel family indicates that TRP1 belongs to an evolutionary distinct branch containing TRPC and TRPM channels.Citation12 Therefore, it is not unreasonable to suggest that Chlamydomonas TRPC-like channels are also implicated in the transduction of environmental cues, since TRPC channels share conserved sequence motifs common to TRPM and TRPV channels. Our analysis identifies Chlamydomonas TRP1, TRP2, TRP3, TRP6, TRP8, TRP10, TRP15, TRP23, and TRP24 as being related to members of the TRPC subfamily. From all the putative channels mentioned, and based on our sequence analysis, we narrow it down to TRP1, TRP5, and TRP16 as being the strongest candidates to serve as repolarization-associated channels. Further cellular, biochemical, and genetic studies are required to characterize the cellular location of TRP16, and the function of these gene transcripts.
Taken together, our results support the hypothesis that Chlamydomonas TRP channels, distant relatives of TRPC, TRPM or TRPV subtypes, carry an important fraction of the potassium outward current that may follow the initial depolarization caused by the activation of sensory receptors, therefore helping in the regeneration of the resting membrane potential. Such TRP channel-dependent control of the sensory input, downstream of receptor activation, has been proposed before for mammalian TRPM channels such as the taste-associated channel TRPM5,Citation28 and TRPM1 channels involved in photosensation.Citation29 Although the BCTC concentrations used in the loose-patch experiments were 10 to 100 times larger than the calculated IC50 for BCTC-dependent inhibition of rat TRPV1 (35 nM)Citation18 and human TRPM8 (143 ± 19 nM),Citation20 they were close enough to the calculated IC50 for Cr-TRP1 (1.03 µM),Citation12 suggesting that this monovalent permeating channel might be part of the repolarizing mechanism in the unicellular alga. Although the possibility persists that in addition to TRPs, other not yet tested BCTC-sensitive conductances may contribute to the repolarization process described here, it is unlikely that calcium or potassium channels present in the algae act as targets of BCTC.Citation18
The swimming algae have the ability to switch between 2 regimes, the self-synchronous regime the algae usually uses while swimming in the dark,Citation7,30 and the light-induced sinusoidal-directed swimming mode.Citation16,30 The former is characterized by long periods of synchronization interrupted by brief asynchrony, allowing helical motion in the dark. On the other hand, the latter defines phototactic mode, that is based on opposite and cell-controlled amplitude modulations of flagellar motion. The signal that triggers the switch between these 2 regimes might be associated with the repolarization that follows sensitive excitability. In the new condition, induced by BCTC, with a slower repolarization process, the cells cannot maintain the balance in the frequency of depolarization events reaching the cilia (necessary to gate Cav conductances), and therefore are unable to sustain sinusoidal swimming under light conditions. Instead, the algae have a tendency to switch back to the intrinsic helical mode for brief periods and become erratic.Citation7 However, asynchronies associated with helical motion are not implemented in phototaxis.Citation30 Thus, it appears that the algae cannot properly orient themselves in relation to the source of the signal, unless swimming in sinusoidal mode. We propose that Chlamydomonas TRP channels located in the cell body are involved in repolarization of the algal membrane, and that TRP channels located in the flagellar/primary cilia have other functions. It is worth noting that RNAi suppression of TRP11 did not affect phototactic or photophobic responses.Citation12 The scheme in depicts a scenario in which a subset of Chlamydomonas TRP channels are associated to the K+ repolarization current following H+/Ca2+-dependent depolarization by Channelrhodopsins, restoring EK allowing for timely and consistent posterior depolarization of the algal plasma membrane.
Methods
Cell culture
C. reinhardtii strains CC-124 mt– (wild-type) and CC-503 cw92 mt+ (cell wall deficient mutant) were maintained in Sueoka's mediumCitation31 at 18°C, under constant agitation, and light/dark periods of 12 h. C. reinhardtii gametes were prepared by incubating vegetative cells with a nitrate-free media overnight in the dark. Recordings were made on fresh C. reinhardtii gametes in Sueoka's bath media. HEK-293T cells were cultured in DMEM (Gibco) supplied with 10% FBS (Gibco). Cells were plated on poly-L-lysine coated coverslips and transfected using Lipofectamine 2000 (Invitrogen) according to the manufacturer's instructions. Recordings on HEK-293T cells were performed 24 to 48 h after transfection.
Video microscopy of swimming algae
Algal behavior was recorded on a Nachet upright microscope equipped with a CCD camera, using a 20X objective. An acrylic chamber with a glass coverslip at the bottom was used for the recordings. For every experiment, 1 × 106 algal cells were placed on the side of the chamber and allowed to swim freely for 2 minutes. To assess the phototactic response (recruitment experiment), the microscope halogen light (1 × 103 erg/cm2s−1) was used as stimulus and the total amount of cells in the field of view was counted in sequential images taken every 30 s. To calculate diffusion and velocity, all movies were recorded at 20 Hz on transmitted light configuration. Algal tracking and trajectory analysis were performed using a custom-made ImageJ plugin.
Photophobic and chemosensing behavior
Petri dishes containing 2 × 106 algal cells were imaged before and after the light or chemical stimulation. For the case of the photophobic response, the light source (2 × 104 erg/cm2s−1) illuminated one side of the dish containing the freely swimming algae, making them swim in the opposite direction. For the case of the chemosensory response we used the chemical attractant tryptone. We prepared agar pellets containing 20% tryptone and deposited them into the petri dish at time zero. Color images were thresholded to select the optically dense (green) area containing algae, and then plotted in a polar graph representing algal positioning with respect to the dish geometry. We averaged the behavioral results (final overall position) coming from different dishes.
Electrophysiology. Electrophysiological recordings of algae population
The experiments were performed as described previously by the group of Oleg Sineschekov.Citation15 A 1 × 1.5 cm acrylic chamber containing 1 × 106 algae was used to record the total current elicited by a flash of light. Two platinum electrodes were placed along each of the larger sides of the chamber. Electrodes were connected to a custom-made current amplifier. Two LED light sources were used, one parallel (pre-pulse aligning flash) and the other perpendicular (test flash) to the electrodes. The aligning flash was pulsed 10 times (100 ms) allowing all algae to orient toward one side of the chamber, then, the test flash eliciting the algae response once current vectors were aligned, was recorded. Data was digitized with a Digidata 1200 (Axon Instruments). WinWCP software was used to control light stimulation and to perform the current recording. Loose-patch experiments on single alga
We used similar conditions to the ones described previously for the ‘suction pipette technique’.Citation23,24 However, we used one pipette and applied constant negative pressure. Seals were formed by using 10- to 30-MΩ borosilicate pipettes (Warner Instruments) with a tip diameter of about 2 µm, with an access resistance of <50 MΩ. Cells were “sucked” into the recording pipette until the seal resistance reached 200- to 400-MΩ. Changes in the membrane potential (Em) were recorded on an Axopatch 200B amplifier (Molecular Devices), allowing for the measurement of unclamped membrane current. Cells were stimulated using a blue LED (465 nm; Phillips) digitally controlled by a Digidata 1320 (Molecular Devices). Trains of 10 light pulses, 100 µs each, stimulated the algae while Em was recorded. The traces were further averaged to generate one single train. A single exponential decay function was fitted to the repolarization phase of the trace. Measurements were performed at 20°C.
Recording solutions
The experiments in freely moving algae were performed in Sueoka's medium. Loose-patch experiments were performed using a Na+ pipette solution containing (in mM): 130 NaCl, 5 KCl, and 20 HEPES, pH 7.4.
Data analysis
Unless stated, group data are presented as means ± SE. Statistical comparisons were made using analysis of variance (one-way ANOVA) and the Bonferroni mean test. Statistical comparisons for algal behavior were made using the Wilcoxon test. A value of P = 0.05 was taken to be statistically significant.
Identification of TRP-Like Channels from Chlamydomonas reinhardtii
BLAST version 2.2.28+ was used to perform BLASTP searches against the proteome of Chlamydomonas reinhardtii, downloaded from Phytozome version 9.1.Citation32,33 Protein sequences corresponding to the 28 mammalian TRP channels functionally annotated in the International Union of Basic and Clinical Pharmacology database (http://www.guidetopharmacology.org/GRAC/FamilyDisplayForward?familyId=78) were downloaded: TRPA1, TRPC1, TRPC3-7, TRPM1-8, TRPML1-3, TRPP1-3, and TRPV1-6 (Homo sapiens), and TRPC2 (Mus musculus); together with NompC (Drosophila melanogaster) and TRPY1 (Saccharomyces cerevisiae). Only the transmembrane domain was used as query for BLASTP searches. Putative TRP channel candidates were selected based on reciprocal BLASTP searches and visual inspection of a sequence alignment with the corresponding best reciprocal hit, generated using MAFFT.33
Accession Numbers
Sequence data from this article can be found in the GenBank/EMBL libraries under the following accession numbers: Cr-TRP1, JX173491.1, ABG54260.1, Phytozome Cre10.g452950.t1.1; Cr-TRP2, Phytozome Cre16.g655950.t1.1; Cr-TRP3, Phytozome Cre07.g327750.t1.1; Cr-TRP5, Phytozome Cre09.g398400.t1.2; Cr-TRP6, Phytozome Cre07.g334300.t1.1; Cr-TRP7, Phytozome Cre08.g358575.t1.1; Cr-TRP8, Phytozome Cre03.g145147.t1.1; Cr-TRP10, Phytozome Cre16.g658900.t1.1; Cr-TRP11, AB596979.1, Phytozome Cre07.g341350.t1.2; Cr-TRP13, Phytozome Cre03.g175050.t1.1; Cr-TRP15, Phytozome Cre09.g397142.t1.1; Cr-TRP16 Phytozome Cre06.g278226.t1.1; Cr-TRP21, Phytozome Cre10.g434600.t1.2; Cr-TRP22, Phytozome Cre02.g112200.t1.2; Cr-TRP23, Phytozome Cre09.g390578.t1.1; Cr-TRP24, Phytozome Cre01.g029400.t1.2; Cr-TRP26, Phytozome Cre12.g529850.t1.1; Cr-TRP27, Phytozome Cre12.g493050.t1.2; Cr-TRP28, Phytozome Cre10.g422750.t1.1; and Cr-TRPP2, AV395567.1, Phytozome Cre17.g715300.t1.2.
Disclosure of Potential Conflicts of Interest
No potential conflicts of interest were disclosed.
Acknowledgments
We thank the members of the Brauchi laboratory for their invaluable help and thoughtful contributions. L. Arias-Darraz is a CONICYT fellow.
Funding
This work was supported by FONDECYT grants 11070190 and 1110906 (SB), and FONDECYT 3140233 (CKC). The Brauchi Laboratory is part of CISNe and PDBC-UACh.
References
- Cosens DJ, Manning A. Abnormal electroretinogram from a Drosophila mutant. Nature 1969; 224:285-7; PMID:5344615; http://dx.doi.org/10.1038/224285a0
- Montell C, Rubin GM. Molecular characterization of the Drosophila trp locus: a putative integral membrane protein required for phototransduction. Neuron 1989; 2:1313-23; PMID:2516726; http://dx.doi.org/10.1016/0896-6273(89)90069-X
- Clapham DE. Transient Receptor Potential (TRP) Channels. In: Encyclopedia of Neuroscience, vol 9, Squire LR (ed), Oxford, UK: Academic Press; 2009; pp 1109-33.
- Damann N, Voets T, Nilius B, Anima I. TRPs in our senses. Curr Biol 2008; 18:880-9; PMID:18812089; http://dx.doi.org/10.1016/j.cub.2008.07.063
- Julius D. TRP channels and pain. Ann Rev Cell Dev Biol 2013; 29:355-84; PMID:24099085; http://dx.doi.org/10.1146/annurev-cellbio-101011-155833
- Harris EH. Chlamydomonas as a model organism. Annu Rev Plant Physiol Plant Mol Biol 2001; 52:363-406; PMID:11337403; http://dx.doi.org/10.1146/annurev.arplant.52.1.363
- Polin M, Tuval I, Drescher K, Gollub JP, Goldstein RE. Chlamydomonas swims with two “gears” in a eukaryotic version of run-and-tumble locomotion. Science 2009; 325:487-90; PMID:19628868; http://dx.doi.org/10.1126/science.1172667
- Merchant SS, Prochnik SE, Vallon O, Harris EH, Karpowicz SJ, Witman GB, Terry A, Salamov A, Fritz-Laylin LK, Maréchal-Drouard L, et al. The Chlamydomonas genome reveals the evolution of key animal and plant functions. Science 2007; 318:245-50; PMID:17932292; http://dx.doi.org/10.1126/science.1143609
- Hegemann P. Algal sensory photoreceptors. Annu Rev Plant Biol 2008; 59:167-89; PMID:18444900; http://dx.doi.org/10.1146/annurev.arplant.59.032607.092847
- Huang K, Diener DR, Mitchell A, Pazour GJ, Witman GB, Rosenbaum JL. Function and dynamics of PKD2 in Chlamydomonas reinhardtii flagella. J Cell Biol 2007; 179:501-14; PMID:17984324; http://dx.doi.org/10.1083/jcb.200704069
- Fujiu K, Nakayama Y, Iida H, Sokabe M, Yoshimura K. Mechanoreception in motile flagella of Chlamydomonas. Nat Cell Biol 2011; 13:630-2; PMID:21478860; http://dx.doi.org/10.1038/ncb2214
- Arias-Darraz L, Cabezas D, Colenso CK, Alegría-Arcos M, Bravo-Moraga F, Varas-Concha I, Almonacid DE, Madrid R, Brauchi S. A transient receptor potential ion channel in Chlamydomonas shares key features with sensory transductio-associated TRP channels in mammals. Plant Cell 2015; 27:177-88; PMID:25595824; http://dx.doi.org/10.1105/tpc.114.131862
- Latorre R, Zaelzer C, Brauchi S. Structure-functional intimacies of transient receptor potential channels. Q Rev Biophys 2009; 42:201-46; PMID:20025796; http://dx.doi.org/10.1017/S0033583509990072
- Steinberg X, Lespay-Rebolledo C, Brauchi S. A structural view of ligand-dependent activation in thermoTRP channels. Front Physiol 2014; 5:171; PMID:24847275; http://dx.doi.org/10.3389/fphys.2014.00171
- Sineshchekov OA, Govorunova EG, Dér A, Keszthelyi L, Nultsch, W. Photoinduced electric currents in carotenoid-deficient Chlamydomonas mutants reconstituted with retinal and its analogs. Biophys J 1994; 66:2073-84; PMID:8075341; http://dx.doi.org/10.1016/S0006-3495(94)81002-1
- Rüffer U, Nultsch W. High-speed cinematographic analysis of the movement of Chlamydomonas. Cell Motil 1985; 5:251-63; http://dx.doi.org/10.1002/cm.970050307
- Govorunova EG, Sineshchekov OA, Hegemann P. Desensitization and dark recovery of the photoreceptor current in Chlamydomonas reinhardtii. Plant Physiol 1997; 115:633-42; PMID:12223832
- Valenzano KJ, Grant ER, Wu G, Hachicha M, Schmid L, Tafesse L, Sun Q, Rotshteyn Y, Francis J, Limberis J, et al. N-(4-tertiarybutylphenyl)-4- (3-chloropyridin-2-yl) tetrahydropyrazine-1(2H)-carbox-amide (BCTC), a novel, orally effective vanilloid receptor 1 antagonist with analgesic properties: I. in vitro characterization and pharmacokinetic properties. J Pharmacol Exp Ther 2003; 306:377-86; PMID:12721338; http://dx.doi.org/10.1124/jpet.102.045674
- Behrendt H-J, Germann T, Gillen C, Hatt H, Jostock R. Characterization of the mouse cold-menthol receptor TRPM8 and vanilloid receptor type-1 VR1 using a fluorometric imaging plate reader (FLIPR) assay. Br J Pharmacol 2004; 141:737-45; PMID:14757700; http://dx.doi.org/10.1038/sj.bjp.0705652
- Weil A, Moore SE, Waite NJ, Randall A, Gunthorpe MJ. Conservation of functional and pharmacological properties in the distantly related temperature sensors TRVP1 and TRPM8. Mol Pharmacol 2005; 68:518-27; PMID:15911692
- Tanimoto S, Sugiyama Y, Takahashi T, Ishizuka T, Yawo H. Involvement of glutamate 97 in ion influx through photo-activated channelrhodopsin-2. Neurosci Res. 2013; 75(1):13-22; PMID:22664343; http://dx.doi.org/10.1016/j.neures.2012.05.008
- Yoshimura K. Mechanosensitive Channels in the Cell Body of Chlamydomonas. J. Membrane Biology 1998; 166:149-155; PMID:9841739; http://dx.doi.org/10.1007/s002329900456
- Harz H, Hegemann P. Rhodopsin-regulated calcium currents in Chlamydomonas. Nature 1991; 351:489-91; http://dx.doi.org/10.1038/351489a0
- Holland EM, Braun FJ, Nonnengässer C, Harz H, Hegemann P. The nature of rhodopsin-triggered photocurrents in Chlamydomonas. I. Kinetics and influence of divalent ions. Biophys J 1996; 70:924-31; PMID:8789109; http://dx.doi.org/10.1016/S0006-3495(96)79635-2
- Govorunova EG, Sineshchekov O. Integration of photo- and chemosensory signaling pathways in Chlamydomonas. Planta 2003; 216:535-40; PMID:12520346
- Govorunova EG, Sineshchekov OA. Chemotaxis in the green flagellate alga Chlamydomonas. Biochemistry (Mosc) 2005; 70:717-25; PMID:16097934; http://dx.doi.org/10.1007/s10541-005-0176-2
- Nonnengässer C, Holland EM, Harz H, Hegemann P. The nature of rhodopsin-triggered photocurrents in Chlamydomonas. II. Influence of monovalent ions. Biophys J 1996; 70:932-8; PMID:8789110; http://dx.doi.org/10.1016/S0006-3495(96)79636-4
- Liman ER. TRPM5 and taste transduction. Handb Exp Pharmacol 2007; 287-98; PMID:17217064
- Shen Y, Heimel JA, Kamermans M, Peachey NS, Gregg RG, Nawy S. A transient receptor potential-like channel mediates synaptic transmission in rod bipolar cells. J Neurosci 2009; 29:6088-93; PMID:19439586; http://dx.doi.org/10.1523/JNEUROSCI.0132-09.2009
- Rüffer U, Nultsch W. Flagellar coordination in Chlamydomonas cells held on micropipettes. Cell Motil Cytoskelet 1998; 41:297-307; PMID:9858155; http://dx.doi.org/10.1002/(SICI)1097-0169(1998)41:4<297::AID-CM3>3.0.CO;2-Y
- Harris EH. The Chlamydomonas Sourcebook, vol I, 2nd Harris EH (ed), Elsevier; 2009.
- Altschul SF, Gish W, Miller W, Myers EW, Lipman DJ. Basic local alignment search tool. J Mol Biol 1990; 215:403-410; PMID:2231712; http://dx.doi.org/10.1016/S0022-2836(05)80360-2
- Goodstein DM, Shu S, Howson R, Neupane R, Hayes RD, Fazo J, Mitros T, Dirk, W, Hellsten U, Putnam N, Rokhsar DS. Phytozome: a comparative platform for green plant genomics. Nucl Acids Res 2012; 40:D1178-86; PMID:22110026; http://dx.doi.org/10.1093/nar/gkr944
- Katoh K, Standley DM. MAFFT multiple sequence alignment software Version 7: Improvements in performance and usability. Mol Biol Evol 2013; 30:772-80; PMID:23329690; http://dx.doi.org/10.1093/molbev/mst010