Abstract
The endoplasmic reticulum (ER) is a multifunctional eukaryotic organelle where the vast majority of secretory proteins are folded and assembled to achieve their correct tertiary structures. The lumen of the ER and Golgi apparatus also provides an environment for numerous glycosylation reactions essential for modifications of proteins and lipids, and for cell wall biosynthesis. These glycosylation reactions require a constant supply of cytosolically synthesized substrate precursors, nucleotide sugars, which are transported by a group of dedicated nucleotide sugar transporters (NST). Recently, we have reported on the identification of a novel ER-localized NST protein, ROCK1, which mediates the transport of UDP-linked acetylated hexosamines across the ER membrane in Arabidopsis. Interestingly, it has been demonstrated that the activity of ROCK1 is important for the regulation of cytokinin-degrading enzymes, cytokinin oxidases/dehydrogenases (CKX), in the ER and, thus, for cytokinin responses. In this addendum we will address the biochemical and cellular activity of the ROCK1 transporter and its phylogenetic relation to other NST proteins.
One of the essential roles of the endoplasmic reticulum (ER) and the Golgi apparatus in eukaryotic cells is to facilitate the transfer of sugars to proteins, lipids, proteoglycans and polysaccharides of the plant cell wall. These diverse glycosylation reactions are mediated by families of glycosyltransferase enzymes and require nucleotide-activated sugar donors as substrates. Most nucleotide sugars, however, are synthesized in the cytosol (that is on the “wrong” side of the membrane) and have to be transported across the ER or Golgi membrane due to their polar character. This transport is mediated by dedicated transporter proteins called nucleotide sugar transporters (NSTs) belonging to the NST/triose-phosphate translocator family. Sequence analyses indicate that this family is probably conserved in all eukaryotes, and comprises 30 members in humanCitation1 and ∼50 members in Arabidopsis.Citation2 Considering also rare and plant-specific nucleotide sugars, it can be estimated that about 30 different nucleotide sugar metabolites are produced in plants.Citation3 Nevertheless, only few of the main nucleotide sugars have been studied in depth regarding the molecular mechanisms of their transport. Additionally, substrate specifities of the corresponding NSTs have only been insufficiently analyzed.
Typical methods to examine plant NST function and substrate specificity have been heterologous complementation tests and direct transport assays with NSTs in isolated microsomal vesicles or reconstituted into proteoliposomes. Since many nucleotide sugars are not available as radiolabeled substrates for biochemical analysis of transport activity, and several NSTs with multi-substrate specificity have been characterized more recently,Citation1,2 the identification of physiological substrates of individual NSTs poses a considerable challenge.
ROCK1 is an NST modulating cytokinin responses in Arabidopsis
In our recent publication, we described the identification of REPRESSOR OF CYTOKININ DEFICIENCY1 (ROCK1) in a genetic screen for suppressor alleles of cytokinin deficiency exercised by overexpression of cytokinin-degrading enzymes, cytokinin oxidases/dehydrogenases (CKXs).Citation4 Our transport assays demonstrated that ROCK1 mainly transports UDP-N-acetylglucosamine (UDP-Glc-NAc) and UDP-N-acetylgalactosamine (GalNAc), and displays low relative activity toward UDP-glucose (Glc).Citation4 Whereas transport of UDP-GlcNAc and UDP-GalNAc has been described in several eukaryotic systems,Citation1 transport of these nucleotide sugars has not been revealed in plants to date. Although the experimental evidence for ROCK1-mediated transport of UDP-GlcNAc and UDP-GalNAc is highly reliable due to low background activity for these substrates in the yeast mutant strain used in the heterologous transport assays,Citation5 the significance of incremental transport of UDP-Glc in ROCK1-containing microsomal vesicles remains unclear due to the high background for this nucleotide sugar. It would be interesting to assess the full transport activity of ROCK1, for example by the new biochemical approach developed by Rautengarten et al.Citation2
Phylogenetic analysis revealed that ROCK1 represents a distinct member of the NST Arabidopsis family () and constitutes a rather isolated clade of NST together with other 4 proteins. Even within this clade, ROCK1 shares low sequence homology with other NSTs and has no obvious sequence paralog in Arabidopsis. Transport activities and physiological function of the remaining NSTs from the group IIIa are largely unexplored. AT5G41760 and AT4G35335 are the closest relatives of ROCK1, sharing about 20% sequence identity to ROCK1. Only AT5G41760 has been analyzed experimentally, and it was shown to be able to transport CMP-sialic acid.Citation6 However, its physiological function remains unclear as sialic acid is probably not a component of plant glycoconjugates.Citation7,8
Figure 1. Unrooted phylogenetic tree of Arabidopsis thaliana NST proteins. Clades are generally numbered as proposed previouslyCitation2 with differences in clustering in groups II and III. Numbers at nodes represent the bootstrap values. The scale bar represents the number of substitutions per site.
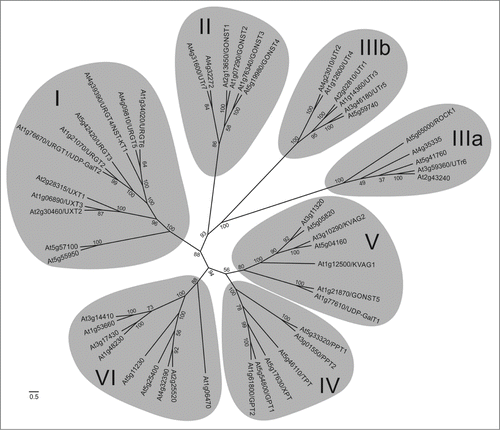
Phylogenetic comparison of all Arabidopsis and human NSTs (solute carrier family 35; SLC35)Citation1 revealed that Arabidopsis clade IIIa is evolutionarily related to subfamily SLC35A (), and that the sequence identity between ROCK1 and several NSTs of this subfamily is higher than between ROCK1 and Arabidopsis NSTs. Based on overall sequence homology, SLC35A3, a previously characterized human Golgi UDP-GlcNAc transporter, is most similar to ROCK1 (23% sequence identity).Citation9 This is interesting because the level of sequence homology between NSTs and their substrate preferences usually does not correlate.Citation10 However, in contrast to SLC35A3,Citation11 ROCK1 is localized to ER and apparently does not play a relevant role during formation of complex N-glycans.Citation4 This suggests that UDP-GlcNAc, which is indispensable for the formation of hybrid and complex N-glycans on secreted and membrane proteins in Golgi,Citation12 is transported by another NST. Hence, this also implies that NSTs with same or similar transport capacities would only have limited functional overlap in the cell. This could be partly due to targeting of individual NSTs to different compartments. However, other mechanisms, such as substrate channeling to a specific glycosyltransferase through direct interaction with an NST, could provide further functional specificity,Citation13,14 Apart from SLC35A3, transport of UDP-GlcNAc in human cells is mediated by 2 other distantly related NSTs with low sequence identity, SLC35B4 and SLC35D2.Citation1 SLC35B4 is similar to Arabidopsis NSTs group IIIb, comprising characterized transporters of UDP-Glc and UDP-galactose (Gal)Citation15,16 and their uncharacterized homologs (). SLC35D2 is phylogenetically related to UDP-Glc/UDP-Gal NST UTR7Citation17 from clade II, which also includes characterized and putative GDP-mannose transporters.Citation18,19 It remains to be tested whether any of the Arabidopsis NSTs from these 2 clades have a transport affinity toward UDP-GlcNAc.
Figure 2. Unrooted phylogenetic tree of Arabidopsis thaliana and human NST proteins. Numbers at nodes represent the bootstrap values. Arrows and asterisks indicate NSTs transporting UDP-GlcNAc and UDP-GalNAc, respectively. Clades are numbered according to .
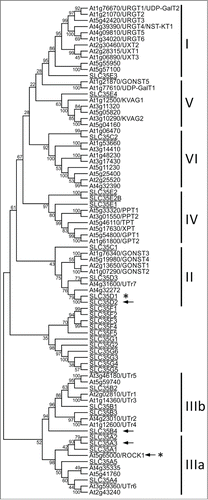
Blast similarity searches of available plant genomes revealed usually a single ROCK1 ortholog in other plant species (). Exceptions were plants with more complex genomes. For example, 2 ROCK1-like NSTs (with 91% sequence identity to each other) are encoded in maize which experienced a relatively recent genome duplication,Citation20 and 4 copies (92–93% amino acid identity to ROCK1 and more than 94% to each other) are present in the allotetraploid genome of oilseed rape Brassica napus. With respect to the evolutionary history of ROCK1, clear sequence orthologs were found in non-vascular land plants, green algae, brown algae and diatoms with about 60, 40, 36 and 37% identity, respectively (). No clear orthologous sequences were detected in available red algae genomes. Interestingly, a single putative ROCK1 ortholog (37% identity) was also found in the choanoflagellate Monosiga brevicollis, which represents the closest known relative of metazoans (animals).Citation21 However, we did not detect any obvious ROCK1 ortholog in Dictyostelium discoideum, fungi, or metazoans. The sequence identities of the closest NST homologs from these organisms were clearly lower (below 25%). Phylogenetic analysis supported the hypothesis that ROCK1 and its putative orthologs are phylogenetically separated from animal homologs including human SLC35A3 (), and that ROCK1 could have acquired a specific function early in the plant evolutionary lineage. We have shown previouslyCitation4 that ROCK1 localization is controlled by the C-terminal dilysine motif.Citation22 Intriguingly, this motif is conserved in most of the putative ROCK1 orthologs in the green plant lineage but is absent in the more diverging homologs (), suggesting that ER retention of ROCK1 could play a role during evolutionary specialization.
Figure 3. Unrooted phylogenetic tree of ROCK1 and homologous NSTs from representative species of selected phylogenetic groups. Proteins are named according to . Numbers at nodes represent the bootstrap values. The scale bar represents the number of substitutions per site.
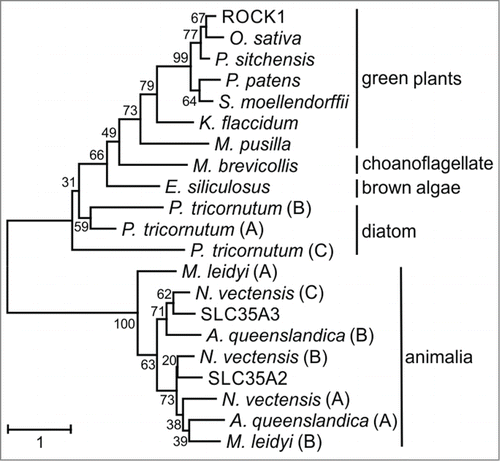
Figure 4. The location of the dilysine motifs in the carboxyl termini of ROCK1 and homologous NSTs from representative species. Canonical dilysine -K(X)KXX motifsCitation22 are underlined.
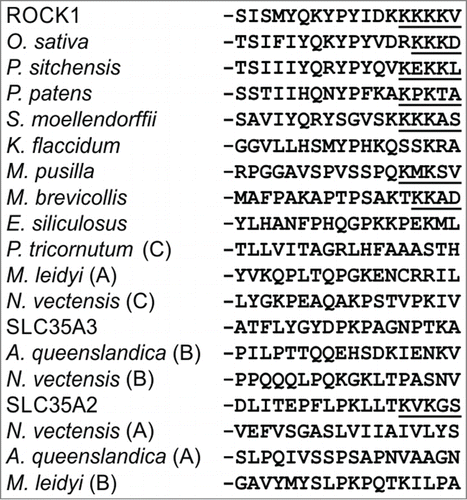
Table 1. ROCK1 sequence homologs from selected plant species and other species representing a specific phylogenetic group.
Biological function of ROCK1 transport activity
First insights into the physiological function of ROCK1 have been gained from the analysis of molecular causes for the suppression of cytokinin deficiency by rock1 mutation. We have shown that the rock1 mutation reduces CKX enzymatic activity in 35S:CKX1 plants by affecting CKX1 protein levels, indicating that ROCK1 plays a role in maintaining the CKX function to lower cytokinin responses.Citation4 How is UDP-GlcNAc/UDP-GalNAc transport to the ER mechanistically connected to the regulation of CKX? Experiments using a 26S proteasome inhibitor have revealed that CKX1, a secretory protein largely localized to the ER, is degraded by the cytosolic proteasome machinery in a process called ER-associated protein degradation (ERAD).Citation23 ERAD represents a conserved cellular route to withdraw proteins from the ER that fail to attain their native conformation and are recognized by the “proof-reading” mechanisms of ER quality control.Citation24 The hypothesis that ROCK1 may actually represent an important component of ER quality control has been supported by the genetic suppression of a partially misfolded and ER-retained mutant variant of brassinosteroid cell surface receptor BRI1Citation25 by rock1.Citation4
Notwithstanding this genetic evidence, the precise molecular effect of ROCK1 on quality control in the ER is currently unclear. A possible model to explain the physiological function of ROCK1 predicts that UDP-GlcNAc and/or UDP-GalNAc are substrates for posttranslational protein modification(s), which in turn may represent functional determinants for quality control and ERAD, with CKX1 being a direct target of this modification. However, in contrast to animals, where GlcNAc and GalNAc are used for luminal protein O-glycosylation on Ser/Thr residues,Citation26 nothing is known about the function of these sugars in the plant ER and this issue will require further research. Moreover, beside the protein modification hypothesis, it cannot be currently ruled out that ROCK1 plays also a role in lipid modification. It has been reported recently that an unusual mannose modification of glycosphingolipids is relevant for regulating salicylic acid responses and shoot development in Arabidopsis.Citation14 Interestingly, the presence of GlcNAc in plant glycosphingolipids has been discussed.Citation27 It is also noteworthy, that the lipid composition of the ER membrane has been reported to contribute to the unfolded protein response.Citation28
We have shown that rock1 mutant plants are characterized by a strongly increased size and activity of the inflorescence meristem, indicating a negative regulatory role of ROCK1 in shoot meristem development. Elevated levels of endogenous cytokinins and cytokinin response genes in rock1 inflorescences suggest that ROCK1 regulates the cytokinin activity in meristematic cells. Furthermore, as very similar changes in inflorescence meristem activity have been described for the ckx3 ckx5 mutant,Citation29 it is plausible that CKX isoforms expressed specifically in the shoot meristem are direct targets of ROCK1 under physiological conditions. However, this needs to be tested experimentally.
A further developmental process requiring ROCK1 activity is pollen development. Dobritsa and colleagues have previously identified ROCK1 as THIN-EXINE 2 (TEX2) in a genetic screen to identify factors important for the formation of the exine, the outer plant pollen wall.Citation30 The pollen of tex2 plants are glossy, sticky and have an abnormally thin exine. Although a link between cytokinin activity and pollen development has been proposed,Citation31 it remains open whether ROCK1-mediated regulation of CKX proteins is also involved in this developmental process or whether ROCK1 activity is required in another way, such as, for example, for delivering the sugar substrates for pollen wall formation.
Methods
Sequences of Arabidopsis and human NSTs were obtained from the Aramemnon database (http://aramemnon.botanik.uni-koeln.de/) and trivial names assigned according to Rautengarten et al.Citation2 Human NST sequences were derived from the HGNC database (http://www.genenames.org/). Putative ROCK1 homologs from representative species were identified by BLASTP searches against the non-redundant protein databases at NCBI and genome sequencing projects of K. flaccidum (http://www.plantmorphogenesis.bio.titech.ac.jp/˜algae_genome_project/kl-ebsormidium/) and M. leidyi (http://research.nhgri.nih.gov/mnemiopsis/). Se-quences showing the highest similarity were included into the analysis. For each phylogenetic tree, the respective sequences were aligned using Clustal OmegaCitation32 on the EMBL-EBI server with default parameters. Phylogenetic analysis was conducted using the maximum likelihood method and MEGA6.Citation33 The Le-Gascuel-model with frequenciesCitation34 was estimated as the optimal substitution model for all performed maximum likelihood analyses. The initial trees for heuristic search were obtained by applying the neighbor-joining method to a matrix of pairwise distances estimated using a JTT model.Citation35 A discrete gamma distribution was used to model evolutionary rate differences among sites (4 categories). For the analysis, all positions with less than 95% site coverage were eliminated. The reliability of interior branches was assessed with 1000 bootstrap resamplings. The cladogram in was generated using FigTree v1.4.2 (http://tree.bio.ed.ac.uk/software/figtree/).
Disclosure of Potential Conflicts of Interest
No potential conflicts of interest were disclosed.
Acknowledgment
We thank Antonia Niedobitek for proofreading.
Funding
We acknowledge financial support from the Deutsche Forschungsgemeinschaft (WE 4325/2–1).
References
- Hadley B, Maggioni A, Ashikov A, Day CJ, Haselhorst T, Tiralongo J. Structure and function of nucleotide sugar transporters: current progress. Comput Struct Biotechnol J 2014; 10:23-32; PMID:25210595; http://dx.doi.org/10.1016/j.csbj.2014.05.003
- Rautengarten C, Ebert B, Moreno I, Temple H, Herter T, Link B, Doñas-Cofré D, Moreno A, Saéz-Aguayo S, Blanco F, et al. The Golgi localized bifunctional UDP-rhamnose/UDP-galactose transporter family of Arabidopsis. Proc Natl Acad Sci USA 2014; 111:11563-8; PMID:25053812; http://dx.doi.org/10.1073/pnas.1406073111
- Bar-Peled M, O'Neill MA. Plant nucleotide sugar formation, interconversion, and salvage by sugar recycling. Annu Rev Plant Biol 2011; 62:127-55; PMID:21370975; http://dx.doi.org/10.1146/annurev-arplant-042110-103918
- Niemann MCE, Bartrina I, Ashikov A, Weber H, Novák O, Spíchal L, Strnad M, Strasser R, Bakker H, Schmülling T, et al. Arabidopsis ROCK1 transports UDP-GlcNAc/UDP-GalNAc and regulates ER protein quality control and cytokinin activity. Proc Natl Acad Sci USA 2015; 112:291-6; PMID:25535363; http://dx.doi.org/10.1073/pnas.1419050112
- Ashikov A, Routier F, Fuhlrott J, Helmus Y, Wild M, Gerardy-Schahn R, Bakker H. The human solute carrier gene SLC35B4 encodes a bifunctional nucleotide sugar transporter with specificity for UDP-xylose and UDP-N-acetylglucosamine. J Biol Chem 2005; 280:27230-5; PMID:15911612; http://dx.doi.org/10.1074/jbc.M504783200
- Bakker H, Routier F, Ashikov A, Neumann D, Bosch D, Gerardy-Schahn R. A CMP-sialic acid transporter cloned from Arabidopsis thaliana. Carbohydr Res 2008; 343:2148-52; PMID:18258224; http://dx.doi.org/10.1016/j.carres.2008.01.010
- Zeleny R, Kolarich D, Strasser R, Altmann F. Sialic acid concentrations in plants are in the range of inadvertent contamination. Planta 2006; 224:222-7; PMID:16395581; http://dx.doi.org/10.1007/s00425-005-0206-8
- Séveno M, Bardor M, Paccalet T, Gomord V, Lerouge P, Faye L. Glycoprotein sialylation in plants? Nat Biotechnol 2004; 22:1351-2; PMID:15529151; http://dx.doi.org/10.1038/nbt1104-1351
- Ishida N, Yoshioka S, Chiba Y, Takeuchi M, Kawakita M. Molecular cloning and functional expression of the human Golgi UDP-N-acetylglucosamine transporter. J Biochem 1999; 126:68-77; PMID:10393322; http://dx.doi.org/10.1093/oxfordjournals.jbchem.a022437
- Berninsone PM, Hirschberg CB. Nucleotide sugar transporters of the Golgi apparatus. Curr Opin Struct Biol 2000; 10:542-7; PMID:11042451; http://dx.doi.org/10.1016/S0959-440X(00)00128-7
- Maszczak-Seneczko D, Sosicka P, Olczak T, Jakimowicz P, Majkowski M, Olczak M. UDP-N-acetylglucosamine transporter (SLC35A3) regulates biosynthesis of highly branched N-glycans and keratan sulfate. J Biol Chem 2013; 288:21850-60; PMID:23766508; http://dx.doi.org/10.1074/jbc.M113.460543
- von Schaewen A, Sturm A, O'Neill J, Chrispeels MJ. Isolation of a mutant Arabidopsis plant that lacks N-acetyl glucosaminyl transferase I and is unable to synthesize Golgi-modified complex N-linked glycans. Plant Physiol 1993; 102:1109-18; PMID:8278542; http://dx.doi.org/10.1104/pp.102.4.1109
- Seifert GJ, Barber C, Wells B, Dolan L, Roberts K. Galactose biosynthesis in Arabidopsis: genetic evidence for substrate channeling from UDP-D-galactose into cell wall polymers. Curr Biol 2002; 12:1840-5; PMID:12419184; http://dx.doi.org/10.1016/S0960-9822(02)01260-5
- Mortimer JC, Yu X, Albrecht S, Sicilia F, Huichalaf M, Ampuero D, Michaelson LV, Murphy AM, Matsunaga T, Kurz S, et al. Abnormal glycosphingolipid mannosylation triggers salicylic acid-mediated responses in Arabidopsis. Plant Cell 2013; 25:1881-94; PMID:23695979; http://dx.doi.org/10.1105/tpc.113.111500
- Reyes F, León G, Donoso M, Brandizzí F, Weber APM, Orellana A. The nucleotide sugar transporters AtUTr1 and AtUTr3 are required for the incorporation of UDP-glucose into the endoplasmic reticulum, are essential for pollen development and are needed for embryo sac progress in Arabidopsis thaliana. Plant J 2010; 61:423-35; PMID:19906043; http://dx.doi.org/10.1111/j.1365-313X.2009.04066.x
- Norambuena L, Nilo R, Handford M, Reyes F, Marchant L, Meisel L, Orellana A. AtUTr2 is an Arabidopsis thaliana nucleotide sugar transporter located in the Golgi apparatus capable of transporting UDP-galactose. Planta 2005; 222:521-9; PMID:15891899; http://dx.doi.org/10.1007/s00425-005-1557-x
- Handford M, Rodríguez-Furlán C, Marchant L, Segura M, Gómez D, Alvarez-Buylla E, Xiong G-Y, Pauly M, Orellana A. Arabidopsis thaliana AtUTr7 encodes a Golgi-localized UDP-glucose/UDP-galactose transporter that affects lateral root emergence. Mol Plant 2012; 5:1263-80; PMID:22933714; http://dx.doi.org/10.1093/mp/sss074
- Baldwin TC, Handford MG, Yuseff M-I, Orellana A, Dupree P. Identification and characterization of GONST1, a Golgi-localized GDP-mannose transporter in Arabidopsis. Plant Cell 2001; 13:2283-95; PMID:11595802; http://dx.doi.org/10.1105/tpc.13.10.2283
- Handford MG, Sicilia F, Brandizzi F, Chung JH, Dupree P. Arabidopsis thaliana expresses multiple Golgi-localised nucleotide-sugar transporters related to GONST1. Mol Genet Genomics 2004; 272:397-410; PMID:15480787; http://dx.doi.org/10.1007/s00438-004-1071-z
- Schnable PS, Ware D, Fulton RS, Stein JC, Wei F, Pasternak S, Liang C, Zhang J, Fulton L, Graves TA, et al. The B73 maize genome: complexity, diversity, and dynamics. Science 2009; 326:1112-5; PMID:19965430; http://dx.doi.org/10.1126/science.1178534
- King N, Westbrook MJ, Young SL, Kuo A, Abedin M, Chapman J, Fairclough S, Hellsten U, Isogai Y, Letunic I, et al. The genome of the choanoflagellate Monosiga brevicollis and the origin of metazoans. Nature 2008; 451:783-8; PMID:18273011; http://dx.doi.org/10.1038/nature06617
- Jackson MR, Nilsson T, Peterson PA. Identification of a consensus motif for retention of transmembrane proteins in the endoplasmic reticulum. EMBO J 1990; 9:3153-62; PMID:2120038
- Römisch K. Endoplasmic reticulum-associated degradation. Annu Rev Cell Dev Biol 2005; 21:435-56; PMID:16212502; http://dx.doi.org/10.1146/annurev.cellbio.21.012704.133250
- Ellgaard L, Helenius A. Quality control in the endoplasmic reticulum. Nat Rev Mol Cell Biol 2003; 4:181-91; PMID:12612637; http://dx.doi.org/10.1038/nrm1052
- Jin H, Yan Z, Nam KH, Li J. Allele-specific suppression of a defective brassinosteroid receptor reveals a physiological role of UGGT in ER quality control. Mol Cell 2007; 26:821-30; PMID:17588517; http://dx.doi.org/10.1016/j.molcel.2007.05.015
- Bennett EP, Mandel U, Clausen H, Gerken TA, Fritz TA, Tabak LA. Control of mucin-type O-glycosylation: a classification of the polypeptide GalNAc-transferase gene family. Glycobiology 2012; 22:736-56; PMID:22183981; http://dx.doi.org/10.1093/glycob/cwr182
- Cacas J-L, Buré C, Furt F, Maalouf J-P, Badoc A, Cluzet S, Schmitter J-M, Antajan E, Mongrand S. Biochemical survey of the polar head of plant glycosylinositolphosphoceramides unravels broad diversity. Phytochemistry 2013; 96:191-200; PMID:23993446; http://dx.doi.org/10.1016/j.phytochem.2013.08.002
- Volmer R, van der Ploeg K, Ron D. Membrane lipid saturation activates endoplasmic reticulum unfolded protein response transducers through their transmembrane domains. Proc Natl Acad Sci USA 2013; 110:4628-33; PMID:23487760; http://dx.doi.org/10.1073/pnas.1217611110
- Bartrina I, Otto E, Strnad M, Werner T, Schmülling T. Cytokinin regulates the activity of reproductive meristems, flower organ size, ovule formation, and thus seed yield in Arabidopsis thaliana. Plant Cell 2011; 23:69-80; PMID:21224426; http://dx.doi.org/10.1105/tpc.110.079079
- Dobritsa AA, Geanconteri A, Shrestha J, Carlson A, Kooyers N, Coerper D, Urbanczyk-Wochniak E, Bench BJ, Sumner LW, Swanson R, et al. A large-scale genetic screen in Arabidopsis to identify genes involved in pollen exine production. Plant Physiol 2011; 157:947-70; PMID:21849515; http://dx.doi.org/10.1104/pp.111.179523
- Kinoshita-Tsujimura K, Kakimoto T. Cytokinin receptors in sporophytes are essential for male and female functions in Arabidopsis thaliana. Plant Signal Behav 2011; 6:66-71; PMID:21301212; http://dx.doi.org/10.4161/psb.6.1.13999
- Sievers F, Wilm A, Dineen D, Gibson TJ, Karplus K, Li W, Lopez R, McWilliam H, Remmert M, Soding J, et al. Fast, scalable generation of high-quality protein multiple sequence alignments using Clustal Omega. Mol Syst Biol 2011; 7:539; PMID:21988835; http://dx.doi.org/10.1038/msb.2011.75
- Tamura K, Stecher G, Peterson D, Filipski A, Kumar S. MEGA6: molecular evolutionary genetics analysis version 6.0. Mol Biol Evol 2013; 30:2725-9; PMID:24132122; http://dx.doi.org/10.1093/molbev/mst197
- Le SQ, Gascuel O. An improved general amino acid replacement matrix. Mol Biol Evol 2008; 25:1307-20; PMID:18367465; http://dx.doi.org/10.1093/molbev/msn067
- Jones DT, Taylor WR, Thornton JM. The rapid generation of mutation data matrices from protein sequences. Comput Appl Biosci 1992; 8:275-82; PMID:1633570