abstract
The mechanisms underlying correlations between ploidy level and cell size in eukaryotes remain unclear. Recently, we showed that cell length was higher in tetraploid than in diploid dark-grown Arabidopsis hypocotyls. Cuticular function was aberrant, and expression of genes of cuticle formation was reduced. Here, the links between cell elongation, cuticular function, and ploidy level in the etiolated hypocotyl were examined. Seedlings defective in cuticle formation exhibited shorter hypocotyls. This was due to inhibition of cell elongation rather than cell proliferation, indicating that the reduced cuticular function was a consequence of tetraploidy-induced cell elongation rather than its cause. Inhibition of hypocotyl elongation by impaired cuticles was lower in tetraploid than diploid, indicating that tetraploid hypocotyls were less sensitive to cuticular damage.
Ploidy level correlates with cell size in many organisms.Citation1 In Arabidopsis, this correlation was particularly apparent for epidermal cell size in leaves and cotyledons.Citation2,3 There are, however, cases in which ploidy level does not positively correlate with cell size.Citation4 Microarray analysis was used to explore ploidy-dependent gene expression in several studies, but only a few genes were identified as differentially expressed in a ploidy-dependent manner.Citation4 Furthermore, no common set of ploidy-related differentially expressed genes was identified from the different studies.Citation5 Given that cell size is determined by a number of factors, at various regulatory levels, that depend on tissue type and developmental stage, it seems unlikely that common regulatory pathways would always be shared between all species and all tissue types.
Recently, we examined post-germination elongation growth of the hypocotyl in Arabidopsis. This developmental process is entirely governed by cell elongation, in both diploid and colchicine-induced tetraploid seedlings.Citation6 Final hypocotyl length was longer by 19% in tetraploid than in diploid as a result of additional stimulation of cell elongation in the tetraploid plants. The difference in hypocotyl length was particularly apparent in dark-grown seedlings. DNA microarray analysis of the actively growing region of dark-grown hypocotyls showed that the gene set downregulated in tetraploid hypocotyls was enriched in genes related to lipid transport. Some of these genes encoded lipid transfer proteins (LTPs), which were proposed as carriers of cuticular lipids or as lipid-derived signaling molecules.Citation7 Cuticle permeability, as determined using toluidine blue (TB), a hydrophilic dye that only penetrates impaired cuticle,Citation8 was higher in tetraploid plants than in diploid plantsCitation6: In the tetraploid, the hypocotyl is stained by TB more broadly than in diploid plants, from the apical down the middle part of the hypocotyl. Cuticular structure was also disorganized and diffused in tetraploid plants, as visualized using transmission electron microscopy (TEM). Similar cuticle phenotypes were observed previously in cuticle-impaired mutants.Citation9-13 Our results therefore suggested that ploidy-dependent cell elongation in Arabidopsis hypocotyls was related to cuticular function.
The cuticle, which covers the outer wall of the shoot epidermis in land plants, acts as a barrier against water loss, supplies mechanical support, and contributes to plant defenses against pathogenic attack.Citation14 Cuticle-defective mutants can exhibit normal or limited growth during stem and leaf development.Citation10,15 To examine the possible link between cell elongation and cuticular function in the hypocotyl, we assessed hypocotyl growth in dark-grown seedlings defective in cuticular lipid export or lipid synthesis. ABCG11/WBC11/DESPERADO and ABCG12/CER5 are ATP binding cassette half-transporters that are thought to mediate cuticular lipid export from the plasma membrane to the apoplast. Their respective loss of function mutants, wbc11 and cer5, exhibited reductions of 40–80% and 55% of the stem wax load, respectively.Citation10,16,17 We used nock-down mutant allele of WBC11, wbc11-2 (SALK_112720C),Citation10 but not a null allele of WBC11, wbc11-3. For, the wbc11-3 allele is reported to show a severe dwarf phenotype, whereas the nock-down mutant allele wbc11–2 shows a fiddlehead-like phenotype, which is typically observed in cuticle-impaired mutants.Citation10 TB staining in 7-day-old dark-grown seedlings was restricted to the apical region in wild-type hypocotyls, whereas staining in wbc11-2 and cer5-2 hypocotyls was observed in the whole region and upper half region of the hypocotyls, respectively (). Hypocotyl length in 13-day-old dark-grown seedlings of wbc11-2 and cer5-2 was shorter by 36% and 10%, respectively, than in the wild-type (). No significant difference in cell number was observed between the wild type and the 2 mutants (). These results indicate that wbc11-2 and cer5-2 are defective in cell elongation in the dark-grown hypocotyl.
Figure 1. Toluidine blue (TB) staining to examine cuticular function in wild-type Arabidopsis and 2 cuticle mutants. Seven-day-old dark-grown wild-type (Col-0), wbc11-2, and cer5-2 seedlings were stained with TB for 2 min and images were recorded using a digital camera (Powershot G12, Canon, Tokyo Japan). Bar = 2 mm.
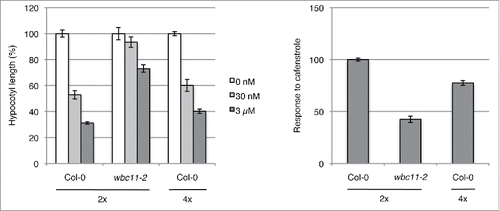
Figure 2. Hypocotyl growth in wild-type Arabidopsis (Col-0) and 2 cuticle mutants, wbc11-2 and cer5-2. (A) Images of 13-day-old seedlings grown on MS gellan gum plates in the dark were recorded using a scanner (GT-9800F, Epson). Image J 1.44 software (www.rsb.info.nih.gov/ij) was used to measure hypocotyl length. Error bars represent SE (n = 20). (B) Total cell numbers for the whole hypocotyl of 5-day-old seedlings grown on MS gellan gum plates in the dark. Cortical cell numbers were counted using a microscope (DMRPX, Leica Microsystems). Error bars represent SE (n = 10). Asterisks indicate significant differences between the wild-type and mutants (**p < 0.01, n.s.: not significant, Student's t-test).
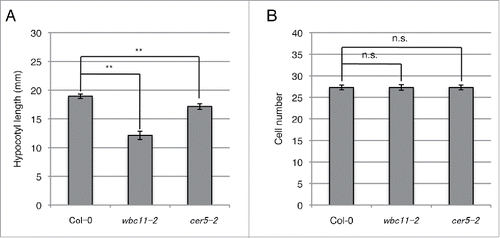
Next, hypocotyl growth was tested in dark-grown seedlings that had been treated with cafenstrole, a potent inhibitor of very-long-chain fatty acid (VLCFA) synthesis.Citation18 VLCFAs are precursors of the majority of cuticular wax. Previous TEM analysis showed that fine cuticle structure was aberrant in seedlings treated with 30 nM cafenstrole, as in cuticle-defective mutants.Citation19 Cafenstrole treatment inhibited hypocotyl growth in wild-type plants, but only partial inhibition was observed in the wbc11-2 mutant (). These results suggest that inhibition of cuticular wax synthesis also causes retardation of cell elongation in the etiolated hypocotyl. We therefore conclude that the reduction in cuticular function does not itself stimulate cell elongation in tetraploid hypocotyls, but rather occurs as a consequence of the increased cell elongation.
Figure 3. Effects of cafenstrole treatment on wild-type (Col-0) diploid and tetraploid Arabidopsis and on one cuticle mutant. Hypocotyl lengths of 11-day-old dark-grown seedlings grown in the absence (0 nM) or presence of cafenstrole (30 nM and 3 μM) were measured. Left panel: Hypocotyl length relative to controls (0 nM). Right panel: Response to cafenstrole treatment, calculated as the inverted ratio of the reduction in hypocotyl length under 3 μM cafenstrole treatment relative to the reduction observed in the wild type (Col-0). Hypocotyl length of dark-grown seedlings grown on MS agar plates was measured as described in . Mean of 3 independent biological replicates of 14–20 plants. Error bars represent SD of the mean. 2x, diploid; 4x, tetraploid.
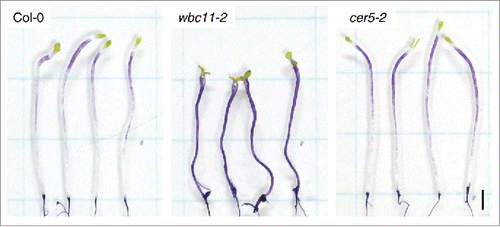
Hypocotyl growth was examined in dark-grown seedlings treated with cafenstrole to test the hypothesis that tetraploid hypocotyls were less sensitive to impaired cuticle than diploid hypocotyls. The inhibitory effect of cafenstrole on hypocotyl growth was slightly but significantly reduced in tetraploid plants compared with diploid plants (). This reduction in sensitivity to cuticular defects may partially explain why hypocotyl growth is stimulated in tetraploid plants in spite of the impaired cuticular function.
Previously, we suggested that cell elongation and cuticular function might be functionally linked during ploidy-dependent cell size elevation in Arabidopsis hypocotyls.Citation6 We proposed 2 possible models to explain the putative pathways. The first model envisages that the cuticle functions as a load-bearing surface that mechanically constrains cell wall expansion and hence controls expansion growth of the whole hypocotyl. According to this model, any reduction in cuticular function would lead to a reduction in mechanical constraint and thereby stimulate growth. However, the present study indicates that Arabidopsis hypocotyls defective in cuticle formation exhibit retardation in cell elongation, suggesting that this model is unlikely ().
The second model envisages that growth resulting from the stimulation of cell elongation stretches and ruptures the cuticular surface in the hypocotyl, producing an impaired cuticle that then inhibits growth. This model is derived from the observation that cuticle formation is ordinarily synchronized with the expansion of surface area in wild-type Arabidopsis stemsCitation20 and assumes that the cell expansion rate in autopolyploid cells exceeds the replenishment rate for the cuticle. Our finding that the tetraploid hypocotyl showed reduced sensitivity to inhibition of growth by impaired cuticles compared with the diploid hypocotyl () is circumstantial support for this model. Polyploidy is thought to enhance stress tolerance, which further supports the second model. For example, autotetraploidy enhanced salt and drought tolerance in light-grown Arabidopsis seedlings, likely through an ABA- and ROS-dependent mechanism.Citation21 In another example, genes encoding LTP proteins, which are involved in the responses to abiotic stressCitation22,23 and disease resistanceCitation13,23-26 as well as in cuticle formation, were significantly downregulated in dark-grown hypocotyls of tetraploid seedlings compared with diploid seedlings.Citation6
Disclosure of potential conflicts of interest
No potential conflicts of interest were disclosed.
Acknowledgments
We thank Dr Shinichiro Komaki and Dr. Keiko Sugimoto RIKEN Center for Sustainable Resource Science for useful discussions, and Ms Kei Saito for excellent technical support.
Funding
This work was supported by a Grant-in-Aid for Scientific Research on Innovative Area, 'Plant cell wall as information-processing system' (grant numbers 24114001 and 24114005) from the Ministry of Education, Culture, Sports, Science and Technology of Japan, to KN.
References
- Müntzing A. The evolutionary significance of autopolyploidy. Hereditas 1936; 21:263-378
- Li X, Yu E, Fan C, Zhang C, Fu T, Zhou Y. Developmental, cytological and transcriptional analysis of autotetraploid Arabidopsis. Planta 2012; 236:579-96; PMID:22476290; http://dx.doi.org/10.1007/s00425-012-1629-7
- Yu Z, Haage K, Streit V, Gierl A, Ruiz RA. Large number of tetraploid Arabidopsis thaliana lines, generated by a rapid strategy, reveal high stability of neo-tetraploids during consecutive generations. Theor Appl Genet 2009; 118:1107-19; PMID:19205656; http://dx.doi.org/10.1007/s00122-009-0966-9
- Sugimoto-Shirasu K, Roberts K. "Big it up:" endoreduplication and cell-size control in plants. Curr Opin Plant Biol 2003; 6:544-53; PMID:14611952; http://dx.doi.org/10.1016/j.pbi.2003.09.009
- Yu Z, Haberer G, Matthes M, Rattei T, Mayer KF, Gierl A, Torres-Ruiz RA. Impact of natural genetic variation on the transcriptome of autotetraploid Arabidopsis thaliana. Proc Nati Acad Sci USA 2010; 107:17809-14; http://dx.doi.org/10.1073/pnas.1000852107
- Narukawa H, Yokoyama R, Komaki S, Sugimoto K, Nishitani K. Stimulation of cell elongation by tetraploidy in hypocotyls of dark-grown Arabidopsis seedlings. PLoS One 2015; 10:e0134547; PMID:26244498; http://dx.doi.org/10.1371/journal.pone.0134547
- Yeats TH, Rose JK. The biochemistry and biology of extracellular plant lipid-transfer proteins (LTPs). Protein Sci 2008; 17:191-8; PMID:18096636; http://dx.doi.org/10.1110/ps.073300108
- Tanaka T, Tanaka H, Machida C, Watanabe M, Machida Y. A new method for rapid visualization of defects in leaf cuticle reveals five intrinsic patterns of surface defects in Arabidopsis. Plant J 2004; 37:139-46; PMID:14675439; http://dx.doi.org/10.1046/j.1365-313X.2003.01946.x
- Chen X, Goodwin SM, Boroff VL, Liu X, Jenks MA. Cloning and characterization of the WAX2 gene of Arabidopsis involved in cuticle membrane and wax production. Plant Cell 2003; 15:1170-85; PMID:12724542; http://dx.doi.org/10.1105/tpc.010926
- Bird D, Beisson F, Brigham A, Shin J, Greer S, Jetter R, Kunst L, Wu X, Yephremov A, Samuels L. Characterization of Arabidopsis ABCG11/WBC11, an ATP binding cassette (ABC) transporter that is required for cuticular lipid secretion. Plant J 2007; 52:485-98; PMID:17727615; http://dx.doi.org/10.1111/j.1365-313X.2007.03252.x
- Go YS, Kim H, Kim HJ, Suh MC. Arabidopsis cuticular wax biosynthesis is negatively regulated by the DEWAX gene encoding an AP2/ERF-Type transcription factor. Plant Cell 2014; 26:1666-80; PMID:24692420; http://dx.doi.org/10.1105/tpc.114.123307
- Kim H, Lee SB, Kim HJ, Min MK, Hwang I, Suh MC. Characterization of glycosylphosphatidylinositol-anchored lipid transfer protein 2 (LTPG2) and overlapping function between LTPG/LTPG1 and LTPG2 in cuticular wax export or accumulation in Arabidopsis thaliana. Plant Cell Physiol 2012; 53:1391-403; PMID:22891199; http://dx.doi.org/10.1093/pcp/pcs083
- Lee SB, Go YS, Bae HJ, Park JH, Cho SH, Cho HJ, Lee DS, Park OK, Hwang I, Suh MC. Disruption of glycosylphosphatidylinositol-anchored lipid transfer protein gene altered cuticular lipid composition, increased plastoglobules, and enhanced susceptibility to infection by the fungal pathogen Alternaria brassicicola. Plant Physiol 2009; 150:42-54; PMID:19321705; http://dx.doi.org/10.1104/pp.109.137745
- Samuels L, Kunst L, Jetter R. Sealing plant surfaces: cuticular wax formation by epidermal cells. Annu Rev Plant Biol 2008; 59:683-707; PMID:18251711; http://dx.doi.org/10.1146/annurev.arplant.59.103006.093219
- Zheng H, Rowland O, Kunst L. Disruptions of the Arabidopsis Enoyl-CoA reductase gene reveal an essential role for very-long-chain fatty acid synthesis in cell expansion during plant morphogenesis. Plant Cell 2005; 17:1467-81; PMID:15829606; http://dx.doi.org/10.1105/tpc.104.030155
- Pighin JA, Zheng H, Balakshin LJ, Goodman IP, Western TL, Jetter R, Kunst L, Samuels AL. Plant cuticular lipid export requires an ABC Transporter. Science 2004; 306:702-4; PMID:15499022; http://dx.doi.org/10.1126/science.1102331
- Panikashvili D, Savaldi-Goldstein S, Mandel T, Yifhar T, Franke RB, Höfer R, Schreiber L, Chory J, Aharoni A. The Arabidopsis DESPERADO/AtWBC11 transporter is required for cutin and wax secretion. Plant Physiol 2007; 145:1345-60; PMID:17951461; http://dx.doi.org/10.1104/pp.107.105676
- Trenkamp S, Martin W, Tietjen K. Specific and differential inhibition of very-long-chain fatty acid elongases from Arabidopsis thaliana by different herbicides. Proc Nati Acad Sci USA 2004; 101:11903-8; http://dx.doi.org/10.1073/pnas.0404600101
- Nobusawa T, Okushima Y, Nagata N, Kojima M, Sakakibara H, Umeda M. Synthesis of very-long-chain fatty acids in the epidermis controls plant organ growth by restricting cell proliferation. PLoS Biol 2013; 11:e1001531; PMID:23585732; http://dx.doi.org/10.1371/journal.pbio.1001531
- Suh MC, Samuels AL, Jetter R, Kunst L, Pollard M, Ohlrogge J, Beisson F. Cuticular lipid composition, surface structure, and gene expression in Arabidopsis stem epidermis. Plant Physiol 2005; 139:1649-65; PMID:16299169; http://dx.doi.org/10.1104/pp.105.070805
- del Pozo JC, Ramirez-Parra E. Deciphering the molecular bases for drought tolerance in Arabidopsis autotetraploids. Plant Cell Environ 2014; 37:2722-37; PMID:24716850; http://dx.doi.org/10.1111/pce.12344
- Guo L, Yang H, Zhang X, Yang S. Lipid transfer protein 3 as a target of MYB96 mediates freezing and drought stress in Arabidopsis. J Exp Bot 2013; 64:1755-67; PMID:23404903; http://dx.doi.org/10.1093/jxb/ert040
- Safi H, Saibi W, Alaoui MM, Hmyene A, Masmoudi K, Hanin M, Brini F. A wheat lipid transfer protein (TdLTP4) promotes tolerance to abiotic and biotic stress in Arabidopsis thaliana. Plant Physiol Biochem 2015; 89:64-75; PMID:25703105; http://dx.doi.org/10.1016/j.plaphy.2015.02.008
- Maldonado AM, Doerner P, Dixon RA, Lamb CJ, Cameron RK. A putative lipid transfer protein involved in systemic resistance signalling in Arabidopsis. Nature 2002; 419:399-403; PMID:12353036; http://dx.doi.org/10.1038/nature00962
- Blein JP, Coutos-Thévenot P, Marion D, Ponchet M. From elicitins to lipid-transfer proteins: a new insight in cell signalling involved in plant defence mechanisms. Trends Plant Sci 2002; 7:293-6; PMID:12119165; http://dx.doi.org/10.1016/S1360-1385(02)02284-7
- McLaughlin JE, Bin-Umer MA, Widiez T, Finn D, McCormick S, Tumer NE. A lipid transfer protein increases the glutathione content and enhances Arabidopsis resistance to a Trichothecene Mycotoxin. PLoS One 2015; 10:e0130204; PMID:26057253; http://dx.doi.org/10.1371/journal.pone.0130204