ABSTRACT
What are the most abundant sphingolipids on earth? The answer is Glycosyl Inositol Phosphoryl Ceramides (GIPCs) present in fungi and the green lineage. In this review, we discuss the putative role of plant GIPCs in the lipid bilayer asymmetry, in the lateral organization of membrane rafts and in the very long chain fatty acid inter-leaflet coupling of lipids in the plant plasma membrane (PM). A special focus on the structural similarities -and putative functions- of GIPCs is discussed by comparison with animal gangliosides, structural homologs of plant GIPCs.
Abbreviations and acronyms
CER | = | Ceramide |
Gal | = | galactose |
GalNAc | = | N-Acetyl galactosamine |
Cer | = | ceramide |
FA | = | Fatty Acid |
Gal | = | galactose |
GIPC | = | Glycosyl Inositol Phosphoryl Ceramides |
GlcA | = | glucuronic acid |
GlcN | = | glucosamine |
GlcR1 | = | glucose with a hydroxyl group, an amine or an N-acetylamine for R1 |
Glc | = | glucose |
gluCER | = | Glucosyl Ceramide |
GPI | = | Glycosylphosphatidylinositol |
Hex | = | hexose |
hVLCFA | = | 2-hydroxylated Very Long Chain Fatty Acid |
Ins | = | Inositol |
IPC | = | Inositol Phosphoryl Ceramides |
IPCS | = | Inositol Phosphoryl Ceramide Synthase |
IPUT1 | = | Inositol Phosphorylceramide glucuronosyltransferase 1 |
LCB | = | Long Chain Base |
PC1P | = | phytoceramide-1-phosphate |
Pen | = | pentose |
PI(4,5)P2 | = | Phosphatidylinositol 4,5-bisphosphate |
PI4P | = | Phosphatidylinositol 4-phosphate |
PM | = | Plasma Membrane |
Sia | = | Sialic acid |
Introduction
In all organisms, the Plasma Membrane (PM) forms a barrier between the cell and the extracellular medium and serves as platform for the orchestration of signal transduction. For example, the PM is directly involved in the perception of pathogens and its translation into a finely tuned and appropriate adaptive response through complex transduction cascades. The understanding of cell membrane organization has evolved since the classic fluid mosaic model.Citation1 It is now recognized that plant PM are highly organized structures, with differences in lipid content between inner and outer leaflets and in lateral structures within the bilayer plane, also called membrane rafts, highly ordered and enriched in sterols and sphingolipids.Citation2,3 These organizing principles are likely crucial for protein localization and function as well as for transducing cellular signaling cascade. Furthermore, the mechanisms and biophysical basis for raft formation, structure, dynamic and function are not clearly understood. The huge lack of knowledge still remaining concerning the molecular organization of plant PMs, and the way this organization governs the set up of signaling processes necessary for cellular adaptation, is a real lock which has to be sprung in plants.
Sphingolipids: crucial elements in membrane organization
PM lipids function in the same time as structural molecules to build a hydrophobic barrier, reservoirs for second messengers (e.g : inositol 3-phosphate deriving from Phosphatidylinositol 4,5-bisphosphate, PtdIns(4,5)P2). Conjointly, they form platforms that organize the assembly of proteins such as regulators, enzymes or ion channels. Three classes of lipids are present in the PM: phosphoglycerolipids, sterols and sphingolipids, these latter ones are supposed to play a major role in raft formation and cross bi-layer lipids interdigitation. Johann Ludwig Wilhelm Thudichum, a German physician and chemist, is considered as the father of sphingolipid research. He isolated several sphingolipids such as cerebroside, sphingosine, and ceramide, and coined the name sphing-o-lipids in 1884 because of their enigmatic properties (“like the Sphinx”). Since their discovery, more than 250 different sphingolipids had been reported and their number is still increasing, but the analysis of their function is still very challenging.Citation4 Sphingolipids can be distinguished in neutral and negatively charged classes. Their biosynthesis starts in the endoplasmic reticulum with the formation of ceramide, CER (N-acyl-Long Chain Base, LCB). Sphingomyelin, with a phosphocholine as polar head, is the most abundant sphingolipid in animals (up to 85% in humans), but animal ceramides can be also glycosylated by a series of membrane-embedded enzymes localized within the secretory pathway. Mammalian glycosphingolipids possess a tremendous diversity of glycan structures that can be grouped into one of the many structural families (e.g. sulfatides, gangliosides, globosides).Citation4,5
GIPCs are major lipids of the plant plasma membrane and are enriched in plasma membrane microdomains
By contrast with animals, sphingomyelin, globosides, sulfatides or gangliosides are absent in plants, but Glycosyl Inositol Phospho Ceramides (GIPCs), glycosylCERamide (gluCER) and ceramide accounted for ca. 64%, 34% and 2%, respectively, of total sphingolipids in Arabidopsis.Citation5 Because of the large biomass of plants and fungi, GIPCs are therefore the most abundant sphingolipids of the biosphere.
During the 60's, pioneering work provided the first structure of the polar head and the ceramide moiety of plant GIPCs.Citation6Citation-8 The core structure of GIPCs generally consists of a trihydroxylated LCB moiety amidified by a 2-hydroxylated Very Long Chain Fatty Acid (hVLCFA) linked to an inositol–glucuronic acid, see left. Many saccharides can be added, forming glycolipids like GIPC series A: GlcR1-GlcA-inositol-1- phosphoceramide or GIPC series B: Gal-GlcR1-GlcA-inositol-1-phosphoceramide (Gal, galactose, GlcR1, glucose with a hydroxyl group, an amine or an N-acetylamine for R1, and GlcA, glucuronic acid).Citation9 Additional saccharides were found to be linked to inositol, e.g., arabinose, galactose, mannose and fucose, forming different series according to the number of sugar moieties,Citation7,10,11,12,9,13 see right. In tobacco, up to 14 sugars (series M) has been observed but not characterized.Citation14 Arabidopsis pollen GIPCs content were found to have a complex array of N-acetyl-glycosylated polar heads, with up to 3 pentose residues, absent in leaf GIPCs, indicating different sphingolipid metabolism in different plant tissues.Citation15 The significance of this remains to be determined. We recently showed that GIPCs are the major lipids of the tobacco (Nicotiana tabacum) highly-purified PM, and can reach up to 40 mol% of total lipids, whereas gangliosides represent only few percent of animal PM, e.g. 0.6% of total brain lipids!Citation16,17
Figure 1. Structure of the different polar heads of plant GIPCs. A, Tobacco GIPC of the series A, hydroxyl groups and net negative charges are indicated Citation14,Citation9; B, Polar head variability of plant GIPCs. GIPC series bearing from one (series 0) to 7 saccharide units (series F). Abbreviations are as follow: Ins: Inositol; Cer: ceramide; FA: Fatty Acid; Gal: galactose; GlcA: glucuronic acid; GlcN: glucosamine; Hex: hexose; (hVLC)FA: (hydroxylated very long chain) fatty acid; LCB: Long Chain Base; Pen: pentose.
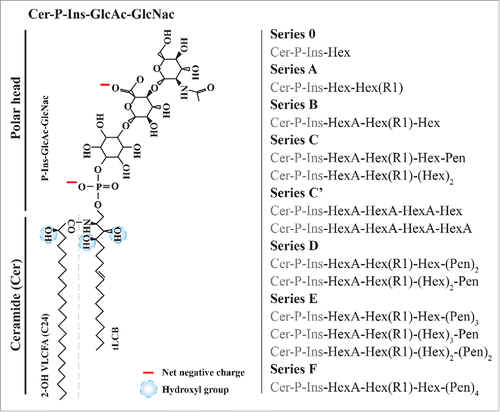
Polyglycosylated GIPCs and polyphosphoinositides cluster within nanodomains in plant PM
The lipid composition of detergent-insoluble membranes (DIMs), the biochemical counterpart of membrane rafts, purified from tobacco plasma membrane (PM) evidenced an enrichment of polyphosphoinositidesCitation18 and polyglycosylated GIPCs.Citation16 Phosphatidylinositol 4-phosphate, PI4P, and Phosphatidylinositol 4,5-bisphosphate PI(4,5)P2, lipids known to be involved in various signal transduction events were enriched in DIMs compared to the whole PM, whereas all structural phospholipids were depleted. Fatty acid analyses showed an enrichment of saturated fatty acids in polyphosphoinositides present in DIMs.Citation18 Similarly GIPCs were also enriched in DIMs and they contain saturated VLCFA.Citation16,19 Using an immunogold-electron microscopy strategy, we were able to visualize domains of PtdIns(4,5)P2 and polyglycosylated GIPCs, clustered in nanodomains at the surface of the PM of 30 to 90 nm in diameter, respectively.Citation18,16 We also showed by multispectral confocal microscopy, allowing spatial analysis of membrane organization of protein-free liposomes, that GIPCs enhanced the sterol-induced ordering effect, emphasizing the formation and increasing the size of sterol-dependent ordered domains. This work supports the involvement of phytosterols in the formation of raft domains, and suggests that the diversity of plant lipids is a major contributor to membrane organization in particular through the formation of GIPC-sterol interacting domains, see .Citation20
Figure 2. Putative model of plant Plasma Membrane lipid organization. The specific interactions between hydroxyl groups of GIPCs with sitosterols are showed in (a), the interdigitation between VLCFA of GIPC in the outer leaflet and FA of Dioleoyl Phosphatidylcholine (Dioleoyl PC) in the inner leaflet are showed in (b).Citation16
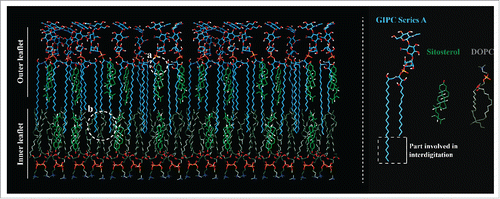
We therefore propose that the diversity of plant lipids organize a set of domains that likely impose a spatial and temporal regulation of protein binding and signaling cascade reactions in the 2 leaflets of the PM.Citation16
Role of interdigitation of GIPC's saturated VLCFA in coupling the inner and the outer leaflets of the PM
GIPCs are lipids with very long saturated acyl chains (up to 26–28). We calculated the depth of insertion of VLCFA of GIPCs into a simplified bilayer (IMPALA method), and compared it with gluCER with 16 carbon atom acyl chains. The results clearly show that the CH3-terminal end of the outer leaflet VLCFAs of GIPC penetrate within the inner leaflet and interdigitate with the inner leaflet lipid acyl chains by at least 6 to 7 carbon atoms, see .Citation16 Although this remains to be fully determined by biophysical studies, these results suggest a strong interdigitation between 40 mol% of the lipids of the plant PM. This function of FA coupling the 2 leaflets is unclear, it could explain the limited diffusion of proteins in the PM, or thermal adaptation.Citation21,22 For example, the role of interdigidation of phosphatidylserine with long saturated acyl chains has been recently shown to be required for actin-based membrane nanoclustering.Citation23 The role of FA interdigitation in plant biology clearly deserves to be fully studied. The dual features of intraleaflet lipid associations and sugar polar head extension in the apoplasm provide the ability of GIPCs to act as cell surface recognition and regulation molecules.
Role of GIPCs in plant biology: Where do we stand?
Several hypotheses have been proposed for the role of GIPCs:
Cell Wall anchoring
GIPCs interact with rhamnogalacturonan II (RG-II) via Boron (B), which suggests a role for GIPCs in the RG-II dimerization process and give a putative first molecular explanation for the cell wall–membrane attachment sites observed in vivo.Citation24
GIPCs as lipid moieties for protein anchoring, cell surface recognition
GPI-anchor proteins play a role in cell-to-cell recognition, signal transduction cascade and nutrient uptake in animals. In yeast, GPI-anchor proteins are linked to the cell wall. Interestingly, GIPC-anchors have been identified as moieties of the lipid anchors of plant proteins targeted to the PM, likely in the outer leaflet.Citation25,26,13 Therefore, GIPC-anchors might enable the clustering of certain proteins in the outer leaflet of the PM, and make potential functional links with plant cell wall.
GIPC precursors of signaling molecules
Sphingolipids have been clearly evidenced as signaling molecules involved in cell death: fungal toxins (like fumonisin B, Alternaria alternata AAL-toxin) can inhibit enzyme of de novo CER biosynthesis and lead to cell death, and a long list of mutants showing a cell death phenotype are involved in sphingolipid metabolism.Citation13,27 These genetic results suggest that sphingolipids may serve as precursors of signaling molecules and influence cell death in plants. In agreement with this, the first enzyme of the GIPC synthesis pathway, IPCS (inositolphosphorylceramide synthase, see ) is involved in the regulation of plant programmed cell death associated with defense in Arabidopsis.Citation28 In addition, Mannopyranosyl Glucuronopyranosyl Inositol accumulated in the culture medium of rose cell-suspensions during the period of cell growth. Authors suggested that GIPCs could be the source of this growth activator compound.Citation29 Finally, a phospholipase D activity that specifically hydrolyzes GIPCs and produces phytoceramide-1-phosphate (PC1P) have been recently identified in Brassicaceae.Citation30 Authors suggested that GIPC-PLD may play an important signaling role in the biological response in plant cells by producing signaling molecules, but the mechanism of regulation of the enzyme activity is largely unknown. GIPC-PLD could also play a role in de-anchoring GPIC-anchored proteins, see above.
Figure 3. Comparison of the biosynthesis of animal ganglioside/sulfatides and plant GIPCs (mostly tobacco). Plant and animal glycosphingolipids are synthesized stepwise by the action of glycosyltransferases. For clarity, the biosynthesis of gangliosides is simplified, e.g., the different forms of sialic acids with 50 naturally occurring structures, are not indicated, as well a number of minor lipids. When known, the genes responsible for the expression of each glycosyltransferase are purple. Note the important number of “?” in the structure and biosynthesis of plant GIPCs.
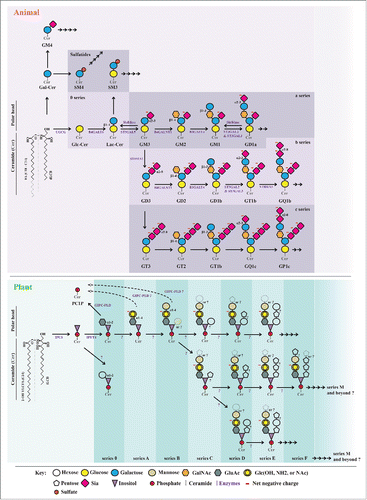
Plant GIPCs are structural homologs of gangliosides in the animal kingdom
GIPCs display a structural similarity with animal gangliosides (see ), a large and complex family of negatively charged lipids mostly residing in the outer leaflet of the PM of animal cells, although some are intracellular.Citation31 Gangliosides are glycosphingolipids composed of a ceramide and an oligosaccharide with one or more sialic acid residues (based on neuraminic acid) linked on a sugar chain. Their polar heads give them a net negative charge, as for GIPCs., Classes of gangliosides are described according to the number of sialic residues (i.e: GM, GD, GT, GQ, GP… stated for Mono-, Di-, Tri-, Quadri- Penta-… up to 7 sialic residues, also called “0- a- b- c- series,” see ). By contrast with GIPCs, gangliosides do not contain phosphate or inositol, and sialic acid is not present in plants.Citation9
Very interestingly, gangliosides are involved in a tremendous number of physiological processes to name a few: host/pathogen relationships, immune defense, surface antigens, blood groups and transplant processes. Gangliosides are thought to provide protection from harsh conditions, such as low pH and degradative enzymes in the extracellular medium, As surface receptor, they play a role in growth and differentiation of tissues as well as in carcinogenesis. For instance, tumor formation can induce the synthesis of new types of gangliosides, that can at very low concentrations induce differentiation of tumor cells in vitro. Subsequently, some gangliosides are useful as cancer markers.Citation17 On the other hand, they have likely an influence on the electrical field across the PM by playing a role in the electrical insulation of myelin cells. Diseases involving ganglioside metabolism -mostly catabolism- are rare but dominated by severe neurological deficiencies. Catabolism disorders result in lysosomal storage diseases marked by progressive neurodegeneration (reviewed inCitation17). Gangliosides have been used as antigens to produce anti-ganglioside antibodies for neurobiologic investigations. Such antibodies have been injected into animals to generate models to study epilepsy and neurological disorders. Thereby, natural and synthetic gangliosides are considered possible therapeutics for neurodegenerative diseases. For example, Guillain-Barré syndrome has been clearly linked to the production of anti-ganglioside antibodies. Finally, The polar heads of gangliosides act as distinguishing surface markers that can interact with extracellular matrices and other cells and serve as surface receptors for bacterial toxins such as cholera letoxin. Consequently, they are also involved in several diseases like in Influenza, in which haemagglutinin of the virus uses gangliosides to enter and infect the cells, in tetanus, botulism and leprosy.Citation31 Futhermore, genetic disruptions of sphingolipid metabolism in animals deregulate immune signaling,Citation32 emphazing their relevance in host-microbes interactions.
The comparison of the structural diversity and functions of gangliosides with what it is known in plant GIPCs () may foreshadow many discoveries in regards to the function of plant GIPCs !
Perspectives
50 years after their discovery, we can conclude that almost nothing is known about the roles of GIPCs in plants! The understanding of the role of these fascinating lipids in biological systems is currently hampered by the fact that no molecular tools are available and efficient purification methods crucially missing. The following questions remain to be challenged: In which leaflet are GIPCs localized? Are they different in the different plant tissues? How are they structured inside the membranes? Do they influence membrane thickness? Do they play a role in temperature adaptation and membrane fluidity? Are they involved as protective layers toward the extracellular harsh conditions? Are transbilayer interactions between outer leaflet long-acyl-chain-containing GIPCs and inner-leaflet lipids pivotal in plant membrane function and signal transduction? Do these interactions provide an underlying mechanism for the generation of functional lipid domains in plant PM? What is their role in plant physiology? Are they involved in host-pathogen relationships? in phytobenefic microorganisms? Is the integrity of membrane domains crucial for elicitor perception and for signal transduction during early events of host-pathogen relationships?
Obtain large amount of purified GIPCs of different series
Efficient methods able to obtain mg amounts of plant GIPCs of different series and different FA content must be developed, as well as obtaining fluorescently-labeled GIPCs and standards for quantification.
Deciphering the structure of plant GIPCs
GIPCs remain poorly characterized in terms of their structures, notably with regard to polar heads. Recently, 23 plant species were analyzed, GIPCs were extracted and their polar heads were characterized by MALDI and ESI mass spectrometry. This study shed light on an unexpected broad diversity of GIPC distributions within plants, and the occurrence of yet-unreported GIPC structures in green and red algae.Citation33 For example, in monocots, GIPCs with 3 saccharides (series B) were apparently found to be preponderant, whereas a series with 2 saccharides (series A) was dominant in dicots with few notable exceptions, . In plant cell cultures, GIPC polar heads appeared to bear a higher number of glycan units than in the tissue from which they originate.Citation33 The shows the comparison between the characterized structure of animal gangliosides / sulfatides and of plant GIPCs. We immediately measure the huge gap in knowledge existing between the animal and the plant kingdom, where the plant GIPC polar head structures are mostly ill-defined: type of sugars, glycosidic bond (i.e: α- and β-, carbon involved), linear or ramified decoration remain to be fully determined.
Computer simulations of plant PM
Resolving the mystery of PM lipid distribution will require advances in both experimentation and modeling. Computational results support the idea that general physical properties of the lipid environment are capable of regulating membrane proteins. Atom-scale computer simulations for 3-component lipid bilayers are very appropriate tools to understand the dynamic organization of membranes.Citation34 Local interactions between the lipid species, the elastic and dynamic properties of the membranes depend strongly on their lipid composition. Based on large-scale molecular dynamic simulations, a high-resolution view of the lipid organization of a plant PM at an unprecedented level of complexity is needed. A special focus is needed on lipid head groups, length of FA, presence of the 2-hydroxylation in FA and asymmetrical distribution of lipids across the 2 leaflets in order to observe an enrichment of phytosterols, non-ideal lateral mixing of different lipid species in the outer or inner leaflets and the coupling of fatty acids across the 2 membrane leaflets… as recently described in animal PMs.Citation34 To do so, experimental biophysical parameters of plant lipids (i.e: area and volume per lipid, membrane thickness, NMR order parameters and X-ray scattering factors) are crucially missing not only for phytosterols, but also for plant sphingolipids, in particular GIPCs.
Biosynthesis of plant GIPCs
By contrast with gangliosides for which the biosynthesis pathways are very well described (, see also http://sphingomap.org), the biosynthesis of plant GIPCs has just begun to be deciphered. In 2015, only 2 steps with their related enzymes have been discovered: the IPC synthase, IPSCitation28 and the IPC glucuronyl transferase, IPUT1.Citation35 Lots of work remain to fully understand the whole biosynthesis pathway and the exact structure of plant GIPCs that may possess up to 14 sugar residues for the series M, present in extremely low amount.Citation10 This leaves open a vast field for the structural and functional study of the most abundant sphingolipids in earth.
Disclosure of potential conflicts of interest
No potential conflicts of interest were disclosed.
Acknowledgements
We are grateful to Dr. Frantisek Baluska for kindly inviting this review and to Françoise Simon-Plas and Patricia Gerbeau for critical reading. This work has been partially funded by the Bordeaux Metabolome Facility-MetaboHUB (grant no. ANR–11–INBS–0010 to S.M.). We acknowledge the French Agence Nationale de la Recherche (ANR), program blanc “PANACEA” NT09_517917, contracts to SM, which supported JLC.
References
- Singer SJ, Nicolson GL. The fluid mosaic model of the structure of cell membranes. Science 1972; 175:720-31; PMID:4333397; http://dx.doi.org/10.1126/science.175.4023.720
- Lingwood D, Kaiser HJ, Levental I, Simons K. Lipid rafts as functional heterogeneity in cell membranes. Biochem Soc Trans 2009; 37:955-60; PMID:19754431; http://dx.doi.org/10.1042/BST0370955
- Mongrand S, Stanislas T, Bayer EM, Lherminier J, Simon-Plas F. Membrane rafts in plant cells. Trends Plant Sci 2010; 15:656-63; PMID:20934367; http://dx.doi.org/10.1016/j.tplants.2010.09.003
- Muthing J, Distler U. Advances on the compositional analysis of glycosphingolipids combining thin-layer chromatography with mass spectrometry. Mass Spectrom Rev 2010; 29:425-79; PMID:19609886; http://dx.doi.org/10.1002/mas.20253
- Markham JE, Li J, Cahoon EB, Jaworski JG. Separation and identification of major plant sphingolipid classes from leaves. J Biol Chem 2006; 281:22684-94; PMID:16772288; http://dx.doi.org/10.1074/jbc.M604050200
- Carter HE, Gigg RH, Law JH, Nakayama T, Weber E. Biochemistry of the sphingolipides. XI. Structure of phytoglycolipide. J Biol Chem 1958; 233:1309-14; PMID:13610833
- Carter HE, Kisic A. Countercurrent distribution of inosol lipids of plant seeds. J Lipid Res 1969; 10:356-62; PMID:4307829
- Carter HE, Koob JL. Sphingolipids in bean leaves (Phaseolus vulgaris). J Lipid Res 1969; 10:363-9; PMID:5797522
- Bure C, Cacas JL, Mongrand S, Schmitter JM. Characterization of glycosyl inositol phosphoryl ceramides from plants and fungi by mass spectrometry. Anal Bioanal Chem 2014; 406:995-1010; PMID:23887274; http://dx.doi.org/10.1007/s00216-013-7130-8
- Hsieh TC, Kaul K, Laine RA, Lester RL. Structure of a major glycophosphoceramide from tobacco leaves, PSL-I: 2-deoxy-2-acetamido-D-glucopyranosyl(alpha1 leads to 4)-D-glucuronopyranosyl(alpha1 leads to 2)myoinositol-1-O-phosphoceramide. Biochemistry 1978; 17:3575-81; PMID:210797; http://dx.doi.org/10.1021/bi00610a024
- Hsieh TC, Lester RL, Laine RA. Glycophosphoceramides from plants. Purification and characterization of a novel tetrasaccharide derived from tobacco leaf glycolipids. J Biol Chem 1981; 256:7747-55; PMID:7263625
- Laine RA, Hsieh TC. Inositol-containing sphingolipids. Methods Enzymol 1987; 138:186-95; PMID:3600321; http://dx.doi.org/10.1016/0076-6879(87)38015-2
- Spassieva S. HJ. Plant Sphingolipids Today - Are They Still Enigmatic? Plant Biology 2008; 5:125-36; http://dx.doi.org/10.1055/s-2003-40726
- Kaul K, Lester RL. Characterization of Inositol-containing Phosphosphingolipids from Tobacco Leaves: Isolation and Identification of Two Novel, Major Lipids: N-Acetylglucosamidoglucuronidoinositol Phosphorylceramide and Glucosamidoglucuronidoinositol Phosphorylceramide. Plant Physiol 1975; 55:120-9; PMID:16659016; http://dx.doi.org/10.1104/pp.55.1.120
- Luttgeharm KD, Kimberlin AN, Cahoon RE, Cerny RL, Napier JA, Markham JE, Cahoon EB. Sphingolipid metabolism is strikingly different between pollen and leaf in Arabidopsis as revealed by compositional and gene expression profiling. Phytochemistry 2015; 115:121-9; PMID:25794895; http://dx.doi.org/10.1016/j.phytochem.2015.02.019
- Cacas JL, Bure C, Grosjean K, Gerbeau-Pissot P, Lherminier J, Rombouts Y, Maes E, Bossard C, Gronnier J, Furt F, et al. Re-Visiting Plant Plasma Membrane Lipids in Tobacco: A Focus on Sphingolipids. Plant Physiol 2015; PMID:26518342; http://dx.doi.org/10.1104/pp.15.00564
- Schnaar RL, Gerardy-Schahn R, Hildebrandt H. Sialic acids in the brain: gangliosides and polysialic acid in nervous system development, stability, disease, and regeneration. Physiol Rev 2014; 94:461-518; PMID:24692354; http://dx.doi.org/10.1152/physrev.00033.2013
- Furt F, Konig S, Bessoule JJ, Sargueil F, Zallot R, Stanislas T, Noirot E, Lherminier J, Simon-Plas F, Heilmann I, et al. Polyphosphoinositides are enriched in plant membrane rafts and form microdomains in the plasma membrane. Plant Physiol 2010; 152:2173-87; PMID:20181756; http://dx.doi.org/10.1104/pp.109.149823
- Bure C, Cacas JL, Wang F, Gaudin K, Domergue F, Mongrand S, Schmitter JM. Fast screening of highly glycosylated plant sphingolipids by tandem mass spectrometry. Rapid Commun Mass spectrom 2011; 25:3131-45; PMID:21953969; http://dx.doi.org/10.1002/rcm.5206
- Grosjean K, Mongrand S, Beney L, Simon-Plas F, Gerbeau-Pissot P. Differential effect of plant lipids on membrane organization: hot features and specificities of phytosphingolipids and phytosterols. J Biol Chem 2015; PMID:25575593; http://dx.doi.org/10.1104/pp.15.00564
- Garner AE, Smith DA, Hooper NM. Sphingomyelin chain length influences the distribution of GPI-anchored proteins in rafts in supported lipid bilayers. Molecular membrane biology 2007; 24:233-42; PMID:17520480; http://dx.doi.org/10.1080/09687860601127770
- Martiniere A, Lavagi I, Nageswaran G, Rolfe DJ, Maneta-Peyret L, Luu DT, Botchway SW, Webb SE, Mongrand S, Maurel C, et al. Cell wall constrains lateral diffusion of plant plasma-membrane proteins. Proc Natl Acad Sci U S A 2012; 109:12805-10; PMID:22689944; http://dx.doi.org/10.1073/pnas.1202040109
- Raghupathy R, Anilkumar AA, Polley A, Singh PP, Yadav M, Johnson C, Suryawanshi S, Saikam V, Sawant SD, Panda A, et al. Transbilayer lipid interactions mediate nanoclustering of lipid-anchored proteins. Cell 2015; 161:581-94; PMID:25910209; http://dx.doi.org/10.1016/j.cell.2015.03.048
- Voxeur A, Fry SC. Glycosylinositol phosphorylceramides from Rosa cell cultures are boron-bridged in the plasma membrane and form complexes with rhamnogalacturonan II. Plant J 2014; 79:139-49; PMID:24804932; http://dx.doi.org/10.1111/tpj.12547
- Morita N, Nakazato H, Okuyama H, Kim Y, Thompson GA, Jr. Evidence for a glycosylinositolphospholipid-anchored alkaline phosphatase in the aquatic plant Spirodela oligorrhiza. Biochimica et biophysica acta 1996; 1290:53-62; PMID:8645707; http://dx.doi.org/10.1016/0304-4165(95)00185-9
- Oxley D, Bacic A. Structure of the glycosylphosphatidylinositol anchor of an arabinogalactan protein from Pyrus communis suspension-cultured cells. Proc Natl Acad Sci U S A 1999; 96:14246-51; PMID:10588691; http://dx.doi.org/10.1073/pnas.96.25.14246
- Berkey R, Bendigeri D, Xiao S. Sphingolipids and plant defense/disease: the “death” connection and beyond. Front Plant Sci 2012; 3:68; PMID:22639658; http://dx.doi.org/10.3389/fpls.2012.00068
- Wang W, Yang X, Tangchaiburana S, Ndeh R, Markham JE, Tsegaye Y, Dunn TM, Wang GL, Bellizzi M, Parsons JF, et al. An inositolphosphorylceramide synthase is involved in regulation of plant programmed cell death associated with defense in Arabidopsis. Plant Cell 2008; 20:3163-79; PMID:19001565; http://dx.doi.org/10.1105/tpc.108.060053
- Smith CK, Fry SC. Biosynthetic origin and longevity in vivo of alpha-d-mannopyranosyl-(1 –> 4)-alpha-d-glucuronopyranosyl-(1 –> 2)-myo-inositol, an unusual extracellular oligosaccharide produced by cultured rose cells. Planta 1999; 210:150-6; PMID:10592043; http://dx.doi.org/10.1007/s004250050664
- Tanaka T, Kida T, Imai H, Morishige J, Yamashita R, Matsuoka H, Uozumi S, Satouchi K, Nagano M, Tokumura A. Identification of a sphingolipid-specific phospholipase D activity associated with the generation of phytoceramide-1-phosphate in cabbage leaves. FEBS J 2013; 280:3797-809; PMID:23738625; http://dx.doi.org/10.1111/febs.12374
- Sonnino S, Prinetti A. Gangliosides as regulators of cell membrane organization and functions. Adv Exp Med Biol 2010; 688:165-84; PMID:20919654; http://dx.doi.org/10.1007/978-1-4419-6741-1_12
- Koberlin MS, Snijder B, Heinz LX, Baumann CL, Fauster A, Vladimer GI, Gavin AC, Superti-Furga G. A Conserved Circular Network of Coregulated Lipids Modulates Innate Immune Responses. Cell 2015; 162:170-83; PMID:26095250; http://dx.doi.org/10.1016/j.cell.2015.05.051
- Cacas JL, Bure C, Furt F, Maalouf JP, Badoc A, Cluzet S, et al. Biochemical survey of the polar head of plant glycosylinositolphosphoceramides unravels broad diversity. Phytochemistry 2013; 96:191-200; PMID:23993446; http://dx.doi.org/10.1016/j.phytochem.2013.08.002
- Ingolfsson HI, Melo MN, van Eerden FJ, Arnarez C, Lopez CA, Wassenaar TA, Periole X, de Vries AH, Tieleman DP, Marrink SJ. Lipid organization of the plasma membrane. J Am Chem Soc 2014; 136:14554-9; PMID:25229711; http://dx.doi.org/10.1021/ja507832e
- Rennie EA, Ebert B, Miles GP, Cahoon RE, Christiansen KM, Stonebloom S, Khatab H, Twell D, Petzold CJ, Adams PD, et al. Identification of a sphingolipid alpha-glucuronosyltransferase that is essential for pollen function in Arabidopsis. The Plant cell 2014; 26:3314-25; PMID:25122154; http://dx.doi.org/10.1105/tpc.114.129171