ABSTRACT
Defense against diverse biotic and abiotic stresses requires the plant to distinguish between self and non-self signaling molecules. Pathogen/microbe-associated molecular patterns (PAMPs/MAMPs) are pivotal for triggering innate immunity in plants. Unlike in animals and humans, the precise roles of nucleic acids in plant innate immunity are unclear. We therefore investigated the effects of infiltration of total Pseudomonas syringae pv. tomato DC3000 (Pto DC3000) RNAs into Arabidopsis plants. The pathogen population was 10-fold lower in bacterial RNAs pre-treated Arabidopsis plants than in the control. Bacterial RNAs purity was confirmed by physical (sonication) and chemical (RNase A and proteinase K digestion) methods. The perception of bacterial RNAs, especially rRNAs, positively regulated mitogen-activated protein kinase (MAPK) and induced a reactive oxygen species burst, callose deposition, salicylic acid (SA) and jasmonic acid (JA) signaling, and defense-related genes. Therefore, bacterial RNAs function as a new MAMP that activates plant innate immunity, providing a new paradigm for plant–microbe interactions.
Abbreviations
ET | = | ethylene |
ETI | = | effector-triggered immunity |
JA | = | jasmonic acid |
MAPK | = | mitogen-activated protein kinase |
PPR | = | pattern recognition receptor |
PTI | = | PAMP-triggered immunity |
SA | = | salicylic acid |
Unlike in vertebrates, a circulating immune system has not been developed in plants. Plants are continuously subjected to invasion by pathogens and insects. Therefore, to overcome these attacks, plants must produce highly effective and specialized immune responses.Citation1-4 The onset of innate immunity in plants is first activated by the recognition of non-self components.Citation5 Foreign non-self signals or molecular patterns are perceived by pattern recognition receptors (PRRs) in plants.Citation6 Two major innate immune systems, pathogen/microbe-associated molecular patterns (PAMPs/MAMPs)-triggered immunity (PTI) and effector-triggered immunity (ETI), are widely known in plants.Citation7
Flagellin, peptidoglycan, lipopolysaccharide (LPS), translation elongation factor-Tu (EF-Tu), cold-shock protein, and fungal chitin are key molecular patterns that activate plant innate immunity.Citation8-10 In animals, nucleic acids play a role in eliciting innate immunity. However, little is known about the effects of nucleic acids on innate immunity in plants. To shed light on the functions of nucleic acids in this process in plants, we infiltrated total bacterial RNAs from Pseudomonas syringae pv. tomato DC3000 (Pto DC3000) into Arabidopsis thaliana leaves and subsequently inoculated the same plants with the same bacterium.Citation11 Notably, pre-infiltration of bacterial RNAs into plants resulted in reduced pathogen population density and activated downstream signaling pathways, as triggered by the typical PTI, in addition to increasing superoxide anion production and callose deposition and positively regulating mitogen-activated protein kinase (MAPK) and defense-related gene expression.Citation11
Intriguingly, plant innate immunity was elicited by total bacterial RNAs but not by Arabidopsis RNA, suggesting that the host plant can recognize bacterial RNAs as a “non-self” signaling molecule.Citation11 However, this suggestion can be countered by the fact that bacterial RNAs are structurally similar to plant ATP.Citation12 Perhaps when bacterial RNAs are placed into the apoplast of Arabidopsis, they are hydrolyzed and their degradation elicits the plant ATP-type defense response. However, this explanation may be inaccurate because sheared bacterial RNAs did not modulate plant innate immunity.Citation11 This result suggests that certain structural features of bacterial RNAs are the major determinants of plant innate immunity.
The major bottleneck of this study was that any contamination of the bacterial RNAs had to be eliminated. To demonstrate the purity of the bacterial RNAs, we used both physical and chemical approaches. When bacterial RNAs are sheared by sonication, they become fragmented and degraded, and any contaminating peptide(s)/proteins remain in the sheared bacterial RNAs sample. Despite the possible existence of peptide(s)/proteins in sheared bacterial RNAs, no plant innate immunity was elicited by this RNA sample, suggesting that intact bacterial RNAs are needed to activate plant innate immunity. To support the function of bacterial RNAs, purified bacterial RNAs were treated with RNase A before infiltration into Arabidopsis leaves. The pathogen population was 10-fold higher in leaves treated with RNase A + bacterial RNAs than in those treated with bacterial RNAs alone.Citation11 In addition, 5-fold more pathogen colonies were observed in leaves treated with bacterial RNAs + proteinase K than in those treated with bacterial RNAs alone.Citation11 These results clearly demonstrate that bacterial RNAs are indeed implicated in the modulation of innate immunity in Arabidopsis.
Although the pathogen level was higher in leaves treated with RNase A + bacterial RNAs versus RNAs alone, this level failed to reach that of the control plants. Moreover, we observed a difference in pathogen population density between plants treated with bacterial RNAs alone and those treated with bacterial RNAs + proteinase KCitation11. It is possible that the activity of RNase A and proteinase K was not completely diminished during the pre-incubation process and that the residual activity affected the pathogen population density. The levels of the pathogen population in plants treated with RNase A alone vs. the water control were significantly different, whereas the same level was detected in plants treated with proteinase K alone versus the water control.Citation11 This finding, along with the observations that ribonucleases modulate plant defense responsesCitation12 and that protease inhibitors widely participate in improving plant defense responses to pathogens and insects,Citation13 suggests that protease may negatively affect plant innate immunity. Based on our results, RNase A and proteinase K may be involved in innate immunity in Arabidopsis.
To investigate whether plant defense hormones function in plant innate immunity triggered by bacterial RNAs, we used representative Arabidopsis mutant lines for salicylic acid (SA), jasmonic acid (JA), and ethylene (ET) signaling, finding that SA and JA signaling pathways are required for bacterial RNAs-mediated Arabidopsis innate immunity.Citation11 A result of our study concerning plant defense signaling pathways differs from that of a previous study. In contrast to flg22- and elf18-induced resistance, we found that bacterial RNAs-induced resistance to Pto DC3000 was completely abolished in npr1, NahG, and jar1-1 mutant plants. The Pto DC3000 population was only significantly reduced when the quadruple mutant dde2 ein2 pad4 sid2 was used, while no changes were observed in single mutants.Citation14 This difference may be explained as follows: 1) Different PTI-enhancing components were used in the experiments (i.e., flg22 or elf18 vs. bacterial RNAs); 2) The pathogen incubation time after pre-treatment with bacterial RNAs was 4 times longer and the pathogen (Pto DC3000) concentration was approximately 100 times higher in our study than in the previous study; and 3) In our study, the Pto DC3000 population was assessed in systemic leaves rather than local leaves. Collectively, these parameters led to significant differences between our results and those of the previous study, which is not surprising.
In conclusion, our study revealed that pre-infiltrating Arabidopsis with bacterial RNAs positively modulated innate immunity in response to Pto DC3000. Despite the strong ability of bacterial RNAs to function as an elicitor of plant innate immunity, several unanswered questions remain: How are bacterial RNAs perceived by specific plant receptor(s)? How can bacterial RNAs survive in the plant apoplast under acidic condition? Our intriguing findings are listed in . Based on the results, the well-known PRRs of chitin, flagella, and EF-Tu do not appear to be receptors of bacterial RNAs. Inside the plant cell, bacterial RNAs positively regulate the MAP kinase signaling cascade, as well as superoxide anion levels, callose deposition, SA and JA signing pathways, and the associated downstream defense-related gene expression levels. The discovery of the role of bacterial RNAs as an elicitor of plant innate immunity provides new insights into this important process. To better understand the underlying mechanisms by which bacterial RNAs play a major role in the regulation of plant innate immunity, further investigations are needed.
Figure 1. A schematic representation of plant innate immunity elicited by bacterial RNAs as a pathogen-associated molecular pattern (PAMP) in plant. Bacterial RNAs may be perceived by either intercellular or intracellular plant pattern recognition receptor(s) (red or blue bar, respectively). Inside the plant cell, bacterial RNAs affect the downstream pathways. Activation of the MAP kinase signaling cascade, reactive oxygen species (ROS) including superoxide anion burst, callose deposition, and defense-related gene expression levels results in elicitation of PAMP-triggered immunity. PM = plasma membrane.
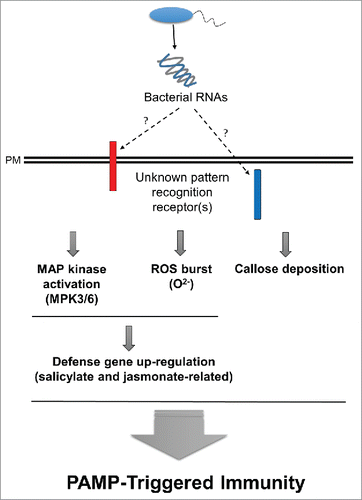
Disclosure of potential conflicts of interest
No potential conflicts of interest are disclosed.
Funding
We are grateful for financial support from the Industrial Source Technology Development Program of the Ministry of Knowledge Economy (10044909) of Korea, the Agenda project of the Rural Development Administration (RDA) (PJ011707), and the KRIBB Initiative Program of South Korea.
References
- Howe GA, Jander G. Plant immunity to insect herbivores. Annu Rev Plant Biol 2008; 59:41-66; PMID:18031220; http://dx.doi.org/10.1146/annurev.arplant.59.032607.092825
- Jones JDG, Dangl JL. The plant immune system. Nature 2006; 444:323-9; PMID:17108957; http://dx.doi.org/10.1038/nature05286
- Pieterse CMJ, van der Does D, Zamioudis C, Leon-Reyes A, van Wees SCM. Hormonal modulation of plant immunity. Annu Rev Cell Dev Biol 2012; 28:489-521; PMID:22559264; http://dx.doi.org/10.1146/annurev-cellbio-092910-154055
- Wu J, Baldwin IT. New insights into plant responses to the attack from insect herbivores. Annu Rev Genet 2010; 44:1021-58; PMID:NOT_FOUND; http://dx.doi.org/10.1146/annurev-genet-102209-163500
- Nurnberger T, Brunner F, Kemmerling B, Piater L. Innate immunity in plants and animals: striking similarities and obvious differences. Immunol Rev 2004; 198:249-66; PMID:15199967; http://dx.doi.org/10.1111/j.0105-2896.2004.0119.x
- Bent AF, Mackey D. Elicitors, effectors, and R genes: the new paradigm and a lifetime supply of question. Annu Rev Phytopathol 2007; 45:399-436; PMID:17506648; http://dx.doi.org/10.1146/annurev.phyto.45.062806.094427
- Tsuda K, Katagiri F. Comparing signaling mechanisms engaged in pattern-triggered and effector-triggered immunity. Curr Opin Plant Biol 2010; 13:459-65; PMID:20471306; http://dx.doi.org/10.1016/j.pbi.2010.04.006
- Nurnberger T, Brunner F. Innate immunity in plants and animals: emerging parallels between the recognition of general elicitors and pathogen-associated molecular patterns. Curr Opin Plant Biol 2002; 5:318-24; PMID:12179965; http://dx.doi.org/10.1016/S1369-5266(02)00265-0
- Zipfel C, Robatzek S, Navarro L, Oakeley EJ, Jones JD, Felix G, Boller T. Bacterial disease resistance in Arabidopsis through flagellin perception. Nature 2004; 428:764-7; PMID:15085136; http://dx.doi.org/10.1038/nature02485
- Ausubel FM. Are innate immune signaling pathways in plants and animals conserved? Nat Immunol 2005; 6:973-9; PMID:16177805; http://dx.doi.org/10.1038/ni1253
- Lee B, Park YS, Lee S, Song GC, Ryu CM. Bacterial RNAs activate innate immunity in Arabidopsis. New Phytol 2016; 209:785-97; PMID:26499893; http://dx.doi.org/10.1111/nph.13717
- Green PJ. The ribonucleases of higher plants. Annu Rev Plant Physiol Plant Mol Biol 1994; 45:421-45; http://dx.doi.org/10.1146/annurev.pp.45.060194.002225
- Ryan CA. Protease inhibitors in plants: genes for improving defenses against insects and pathogens. Annu Rev Phytopathol 1990; 28:425-49; http://dx.doi.org/10.1146/annurev.py.28.090190.002233
- Tsuda K, Sato M, Stoddard T, Glazebrook J, Katagiri F. Network properties of robust immunity in plants. PLoS Genet 2009; 5:e1000772; PMID:20011122; http://dx.doi.org/10.1371/journal.pgen.1000772