ABSTRACT
To overcome high temperature stress, plants have developed transcriptional cascades which express a large amount of chaperone proteins called heat shock proteins (HSPs). In our recent publication, we reported that STABILIZED1, as an U5-snRNP-interacting protein, is involved in the splicing of heat shock factor (HSF) and HSP transcripts during high temperature stress. This indicates that not only transcriptional regulation, but also post-transcriptional regulation by STA1, is essential for the full activation of HSF-HSP cascades and for thermotolerance. Here, we observed that the splicing of HSP transcripts was induced independent of STA1 at room temperature after heat acclimation, indicating that STA1 acts as a high temperature-specific splicing factor for the splicing of HSP transcripts. Our findings suggest the molecular mechanism for how HSF and HSP transcripts are spliced well under high temperature stress that blocks the splicing of overall transcripts.
High temperature is an environmental stress that can induce fatal damage to all organisms, encompassing plants and animals. Heat stress affects every aspect of plant growth, development and physiology.Citation1 At the molecular level, the most extensive damage caused by high temperatures is the denaturation or aggregation of intracellular proteins. Denatured proteins not only lose their biological activity, they also act as toxic molecules inducing programmed cell death.
To overcome the cellular disasters caused by heat stress, plants induce the expression of chaperone proteins that assist proteins to fold properly and maintain their biological activity.Citation2 Molecular chaperone proteins, also called heat-shock proteins (HSPs), are explosively expressed against high temperature stress; such expressions are regulated by upstream transcription factors, heat-shock transcription factors (HSFs).Citation3,Citation4 In Arabidopsis, 21 genes encoding HSF have been identified, and their expression is induced under various abiotic conditions, including cold, drought, osmotic, oxidative and heat stresses.Citation5,Citation6 Several studies have shown that HSFA3 is directly upregulated by the dehydration-responsive element binding protein 2A (DREB2A) during high temperatures, indicating that DREB2A→ HSFA3→HSP transcriptional cascades contribute to resistance to high temperature stress.Citation7,Citation8
Overall cellular activity is suppressed during heat stress, which includes post-transcriptional regulation or pre-mRNA splicing.Citation9-11 Many alternatively spliced transcripts are produced, especially intron-retained forms, and they are retained in the nucleus as a mechanism of mRNA quality control.Citation12,Citation13 Although intron-containing pre-mRNAs leak out of the nucleus, they are not translated into normal proteins due to the presence of a premature stop codon on the intron. However, in the case of HSFs and HSPs, their transcripts are properly spliced and translated into proteins, resulting in resistance to high temperatures.Citation14 This suggests the presence of a specific splicing factor that selectively acts on HSF and HSP transcripts under heat stress conditions.
Our recent study has shown that the U5-snRNP-interacting protein called STABILIZED1 (STA1) has an important role during high temperature stress responses.Citation15 STA1 was originally found to regulate the splicing of cold responsive genes such as COR15A under low temperature stress.Citation16 As a heat inducible gene, STA1 is also responsible for activating DREB2A-HSFA3 transcriptional cascades by being involved in the pre-mRNA splicing of HSFA3.Citation15,Citation17 Furthermore, global target analysis has shown that STA1 regulates the pre-mRNA splicing of a subset of HSPs as well as HSFA3, indicating that STA1 acts as a splicing factor specifically for heat stress responses. To verify the contribution of STA1 at the organism level, an acquired heat tolerance assay was conducted using sta1-1 mutant seedlings with complementation transgenic lines. Instead of the conventionally used method, we used a slightly modified heat treatment condition that had no recovery time between 38°C and 45°C to observe the heat specific splicing activity of STA1.Citation15,Citation18 With this modified method, we found that STA1 is indispensable for the splicing of HSFs and HSPs under high temperatures.
To support our results, a heat tolerance assay was conducted with five-day-old seedlings of wild type (Col) and sta1-1 mutants at each condition, with or without recovery time (). For the conventional heat treatment, seedlings were first exposed to an acclimation temperature of 38°C for 2 h, recovered for 2 h and then treated at 45°C for 2 h. Seeding viability was monitored for seven days after the heat treatment. Surprisingly, sta1-1 mutant seedlings maintained green shoots and survived under conventional heat treatment conditions (), but failed to survive under the modified heat treatment method without recovery time, which is consistent with our previous work (). To monitor the molecular responses of sta1-1 mutant under different heat treatment conditions, the expressions of HSP23.6 and HSA32, genes that are targets of STA1 and are induced by high temperatures, were measured using quantitative RT-PCR. To measure spliced transcripts, primers were designed on the exon-exon junctions of HSPs. The expression levels of mature transcripts were highly induced one day after both heat treatment conditions for Col (), but those for sta1-1 mutant differed greatly. Consistent with the phenotype shown in sta1-1 mutant, the expression of these genes was induced in more than half of Col under conventional heat treatment conditions ( & ). On the other hand, their expression showed no increase under heat treatment without recovery time ( & ). In sta1-1, the presence of recovery time after heat acclimation enhanced the expression of HSPs after heat shock at 45°C and ultimately raised stress tolerance to high temperatures. However, unlike that in sta1-1, the expression of HSP23.6 in Col was significantly higher in heat treatment without recovery time than conventional heat treatment conditions ( & ).
Figure 1. Different responses of sta1-1 to different types of heat treatment conditions. Seedling survival assays were carried out with Col and sta1-1 for acquired heat treatments with (A) and without (B) recovery time between 38°C and 45°C. Schematic models describe the detailed heat treatment conditions. The gene expressions of HSP23.6 (C and D) and mature HSA32 (E and F) were quantitatively monitored before and one day after acquired heat treatment conditions, with (C and E) and without (D and F) recovery time, using RT-qPCR. Quantitative values were normalized with the internal control EIF4a. The means of triplicates are shown with standard error bars. Different letters indicate a significance difference found by the Tukey-Kramer test (p<0.05).
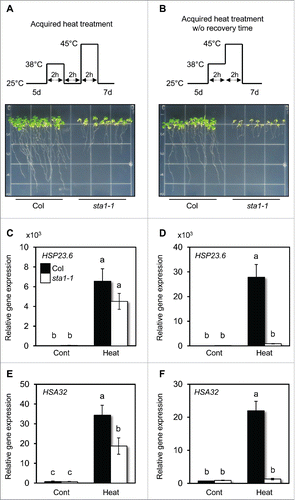
To precisely monitor the splicing pattern of HSP23.6 by STA1 under heat stress, Col and sta1-1 seedlings were incubated at 38°C for 2 h, followed by recovery at 25°C or continued incubation at 38°C (). Total RNA was extracted every hour and used for cDNA synthesis. In Col, the level of the mature transcripts of HSP23.6 steadily increased during incubation at 38°C. Upon recovery to room temperature, the expression of HSP23.6 decreased slowly but remained at a high level. In sta1-1 mutant, the heat treatment at 38°C did not increase mature HSP23.6 transcripts. However, when sta1-1 was recovered at 25°C, mature HSP23.6 transcripts began to gradually accumulate. Although this level of HSP23.6 was lower than that of Col, it was sufficient to have resistance to high temperature (, ), indicating that other splicing factors are responsible for the splicing of HSP23.6 at 25°C on behalf of STA1. These results imply that STA1 plays a role as a high temperature-specific splicing factor that acts on the splicing of HSF and HSP transcripts during high temperature stress that suppresses the splicing of most transcripts.
Figure 2. Restoration of splicing activity for HSP23.6 transcript under room temperature in sta1-1. The expression of mature HSP23.6 was monitored with (solid line) and without (dashed line) recovery after two hours of heat treatment at 38°C in Col and sta1-1 seedlings. The circle and triangle indicate Col and sta1-1 samples respectively. The time points for sample harvests are marked with the letters a to e. Detailed conditions are described with the schematic model. Quantitative values were normalized with the internal control EIF4a. The means of triplicates are shown with standard error bars.
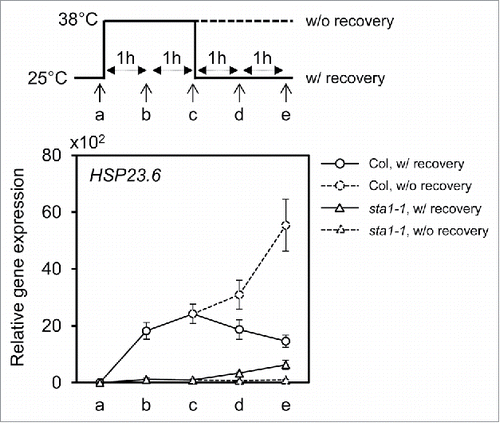
Our findings suggest the presence of a stress-specific splicing factor or spliceosome. The next research area will be the elucidation of molecular mechanisms on how STA1 maintains its activity at high temperatures and how STA1 targets specific transcripts such as HSFs or HSPs.
Disclosure of potential conflicts of interest
No potential conflicts of interest were disclosed.
Additional information
Funding
References
- Hasanuzzaman M, Nahar K, Alam MM, Roychowdhury R, Fujita M. Physiological, biochemical, and molecular mechanisms of heat stress tolerance in plants. Int J Mol Sci. 2013;14:9643–84. doi:10.3390/ijms14059643.
- Hartl FU, Bracher A, Hayer-Hartl M. Molecular chaperones in protein folding and proteostasis. Nature. 2011;475:324–32. doi:10.1038/nature10317.
- Lindquist S, Craig EA. The Heat-Shock Proteins. Annu Rev Genet. 1988;22:631–77. doi:10.1146/annurev.ge.22.120188.003215.
- Wang W, Vinocur B, Shoseyov O, Altman A. Role of plant heat-shock proteins and molecular chaperones in the abiotic stress response. Trends Plant Sci. 2004;9:244–52. doi:10.1016/j.tplants.2004.03.006.
- von Koskull-Doring P, Scharf KD, Nover L. The diversity of plant heat stress transcription factors. Trends Plant Sci. 2007;12:452–7. doi:10.1016/j.tplants.2007.08.014.
- Guo M, Liu JH, Ma X, Luo DX, Gong ZH, Lu MH. The Plant Heat Stress Transcription Factors (HSFs): Structure, Regulation, and Function in Response to Abiotic Stresses. Front Plant Sci. 2016;7:114. doi:10.3389/fpls.2016.00114.
- Schramm F, Larkindale J, Kiehlmann E, Ganguli A, Englich G, Vierling E, Von Koskull-Döring P. A cascade of transcription factor DREB2A and heat stress transcription factor HsfA3 regulates the heat stress response of Arabidopsis. Plant J. 2008;53:264–74. doi:10.1111/j.1365-313X.2007.03334.x.
- Yoshida T, Sakuma Y, Todaka D, Maruyama K, Qin F, Mizoi J, Kidokoro S, Fujita Y, Shinozaki K, Yamaguchi-Shinozaki K. Functional analysis of an Arabidopsis heat-shock transcription factor HsfA3 in the transcriptional cascade downstream of the DREB2A stress-regulatory system. Biochem Biophys Res Commun. 2008;368:515–21. doi:10.1016/j.bbrc.2008.01.134.
- Bond U. Heat-Shock but Not Other Stress Inducers Leads to the Disruption of a Subset of Snrnps and Inhibition of Invitro Splicing in Hela-Cells. Embo J. 1988;7:3509–18.
- Utans U, Behrens SE, Luhrmann R, Kole R, Kramer A. A Splicing Factor That Is Inactivated during Invivo Heat-Shock Is Functionally Equivalent to the [U4/U6.U5] Triple Snrnp-Specific Proteins. Gene Dev. 1992;6:631–41. doi:10.1101/gad.6.4.631.
- Shalgi R, Hurt JA, Lindquist S, Burge CB. Widespread inhibition of posttranscriptional splicing shapes the cellular transcriptome following heat shock. Cell Rep. 2014;7:1362–70. doi:10.1016/j.celrep.2014.04.044.
- Filichkin SA, Priest HD, Givan SA, Shen R, Bryant DW, Fox SE, Wong WK, Mockler TC. Genome-wide mapping of alternative splicing in Arabidopsis thaliana. Genome Res. 2010;20:45–58. doi:10.1101/gr.093302.109.
- Casolari JM, Silver PA. Guardian at the gate: Preventing unspliced pre-mRNA export. Trends Cell Biol. 2004;14:222–5. doi:10.1016/j.tcb.2004.03.007.
- Biamonti G, Caceres JF. Cellular stress and RNA splicing. Trends Biochem Sci. 2009;34:146–53. doi:10.1016/j.tibs.2008.11.004.
- Kim GD, Cho YH, Lee BH, Yoo SD. STABILIZED1 Modulates Pre-mRNA Splicing for Thermotolerance. Plant Physiol. 2017;173:2370–82. doi:10.1104/pp.16.01928.
- Lee BH, Kapoor A, Zhu J, Zhu JK. STABILIZED1, a stress-upregulated nuclear protein, is required for pre-mRNA splicing, mRNA turnover, and stress tolerance in Arabidopsis. Plant Cell. 2006;18:1736–49. doi:10.1105/tpc.106.042184.
- Yu SI, Han JH, Chhoeun C, Lee BH. Genetic Screening for Arabidopsis Mutants Defective in STA1 Regulation under Thermal Stress Implicates the Existence of Regulators of Its Specific Expression, and the Genetic Interactions in the Stress Signaling Pathways. Front Plant Sci. 2016;7:618. doi:10.3389/fpls.2016.00618.
- Silva-Correia J, Freitas S, Tavares RM, Lino-Neto T, Azevedo H. Phenotypic analysis of the Arabidopsis heat stress response during germination and early seedling development. Plant Methods. 2014;10:7. doi:10.1186/1746-4811-10-7.