ABSTRACT
Sulfur (S) is a macronutrient for the plant, which has an immense role in basic plant processes and regulation of several metabolic pathways. It has also a major role in providing protection against adverse conditions. Sulfur-containing amino acids and metabolites maintain plant cell mechanisms to improve stress tolerance. It interacts with several biomolecules such as phytohormones, polyamines, nitric oxide (NO), and even with other plant nutrients, which can produce some derivatives those are essential for abiotic stress tolerance. Different S derivatives stimulate signaling cascades, for the upregulation of different cellular messengers such as abscisic acid, Ca2+, and NO. Sulfur is also known to interact with some essential plant nutrients by influencing their uptake and transport, hence, confers nutrient homeostasis efficiencies. This review focuses on how S is interacted with several signaling molecules like NO, glutathiones, phytohormones, hydrogen sulfide, polyamines, etc. This is a concise summary aimed at guiding the researchers to study S-related plant processes in the light of abiotic stress tolerance.
As a sessile organism, the plant faces various obstacles in acclimation and adaptation to the surrounding environment.Citation1 The role of plant nutrients in plant abiotic stress is well known. Although sulfur (S) is the fourth major nutrients, it plays a vital role in regulating many foremost plant processes. There are some sporadic research findings confirmed about signaling function of S and its derivatives such as glutathione (GSH), hydrogen sulfide (H2S), methionine (Met), cysteine (Cys), phytochelatin (PC), ATP sulfurylase (ATPS), protein thiols, etc.Citation2 Sulfur and its derivatives have been reported to improve the antioxidant defense to scavenge excessive reactive oxygen species (ROS) under different abiotic stresses. In presence of S, the GSH content, ratio of GSH and glutathione disulfide, GSSG (GSH/GSSG), non-protein thiols (NPTs) and PCs, ascorbate (AsA) content, ratio of AsA and dehydroascorbate, DHA (AsA/DHA), activities of AsA peroxidase (APX), GSH reductase (GR), catalase (CAT) were improved in Cd affected Brassica juncea. Moreover, superoxide dismutase (SOD) expression was upregulated that decreased superoxide radicals (O2•−).Citation3 Sulfur addition decreased the As uptake and content in the shoot, modulated thiol metabolism, glycolysis and amino acid in Oryza sativa.Citation4 Apart from the direct effect, the interaction of S with other molecules is very common to counteract abiotic stress. Sulfur can interact with different phytohormones including auxins (AUX), gibberellins (GA), cytokinins (CK), abscisic acid (ABA), ethylene (ET), jasmonic acid (JA) and salicylic acid (SA) under abiotic stress situation, and positively regulates plant defenseCitation5 (). Sulfur contributes to improving stress tolerance by interacting with some other plant nutrients. Some S derivatives like H2S involved in a complex signaling network consisting of many secondary messengers and phytohormones such as Ca2+, ABA, hydrogen peroxide (H2O2), nitric oxide (NO), and polyamines (PAs), which protect the plants from stress-induced damagesCitation6 (). Recently, reactive S species (RSS), a group of S-related free radicals have been designated, which modulate the redox status of biological thiols and disulfides, hence positively act to confer stress tolerance.Citation7 Therefore, it is necessary to extend research with S to exploit its function thoroughly. In this review, we present a brief overview of the interaction between S and other cellular molecules in enhancing abiotic stress tolerance based on the recent available literature.
Figure 1. Interaction of S with phytohormones and other signaling molecules in the signaling cascade to combat abiotic stresses. Here, GSH plays a central role which interacts with NO and produce GSNO to provide signal. GSH is also actively involved in metal chelation and also regulates antioxidant defense system. NO is directly interacted with H2S which further modulates several molecules and their functions. Cysteine is one of the major S-containing amino acids which is interacted with several phytohormones and also regulates PAs and other molecules via several pathways. Dotted lines represent activation or enhancement. Additional details are in text.
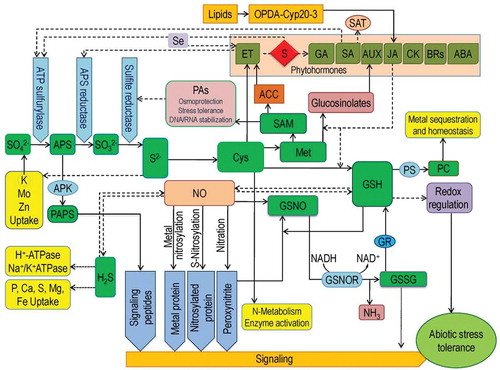
Sulfur is associated with phytohormones regulation
Although phytohormones influence metabolism of S, some phytohormone require S or its derivative compounds for their biosynthesis. The greatest instance is the ET, and the biosynthesis of ET is evidently reliant on S bioavailability and firmly connected with Met metabolism.Citation8 At the beginning Met activated to form S-adenosylmethionine (SAM), which transformed to 1-aminocyclopropane carboxylate (ACC). This ACC is used as the substrate for ethylene biosynthesis. On the other hand, the co-product from ACC biosynthesis —5-methylthioadenosine, is further recycled back to Met and serve as the source of S to plants.Citation9 Sulfate (SO42−) deficiency to plants not only reduces the SAM levels but also disrupts the ethylene and ABA formation; leads to lower Cys synthesis rate, in turns lower levels of ABA, and poor abiotic stress tolerance.Citation10 Therefore, efficient S metabolism is urgent for biosynthesis of at least some of the phytohormones such as AUX and indole 3-acetic acid (IAA).Citation11,Citation12 For instance, decrease in GSH levels in the cell results in a sharp decline of AUX gradient in root tips and showed alteration of lateral root growth and density.Citation12 Although, CK levels declined in SO42− deficit plant leaves this hormone is not linked directly to the response of S starvation, rather regulates SO42− uptake and metabolism. Moreover, CK receptor – CRE1 and CK act similarly in SO42− uptake and regulation.Citation13
In plants, JA or its derivatives induce sulfide formation, GSH biosynthesis, and glucosinolate (GS) metabolism without altering the steady state of Cys or GSH, but accumulate GSs.Citation14 Some transcription factors also responsible for controlling JA responses, namely MYC2, MYC3, and MYC4, also controls GS synthesis.Citation15 Sulfur yet again had some role in JA signaling via 12-oxo-phytodienoic acid (OPDA) and cyclophilin CYP20-3 JA precursors. For producing O-acetyl serine (OAS), the precursor of Cyt, OPDA-CYP20-3 complex interacts with serine acetyltransferase (SAT), and to accomplish this mechanism S metabolites are necessary.Citation16 On the other hand, 3ʹ-Phosphoadenosine-5ʹ-phosphosulfate (PAP) is also responsible for signaling S metabolism during drought stress.Citation17
Both ET generation and S metabolism path shares several metabolites. Assimilation of SO4Citation2− during salt stress revealed that APS reductase activity is upregulated by exogenous application of ET precursor ACC, which increased transcription of APR1 and APR3 genes.Citation18 Methionine is a precursor of ET, and that cease ET biosynthesis. Methionine pathway also contributes to the metabolism of ET and SCitation19 by SAM, which is also an ET precursor. Mao et al.Citation20 reported that FERONIA, a plasma membrane receptor-like kinase, is involved in regulation of SAM. Moreover, reports suggest that ET increases ATPS activity and S uptake in Brassica.Citation21
Among the classical plant hormones connected to abiotic stress especially salinity and drought, ABA is the most important. In plants, oxidative stress induces SO42− assimilation, as well as sulfite reductase, ATPS, APS reductase (APR), SAT, and SO42− transporters.Citation22 Furthermore, SO42− affects ABA signaling to root-to-shoot and influence stomatal closure at the time of drought.Citation23 Another S containing gaseous molecule H2S worked at the downstream pathway of ABA and upstream pathway of NO, which aid in ABA modulated stomatal closure to cope up with drought.Citation24 Thus, ABA is greatly interconnected with S assimilation rather than simple regulation.
There is a great interaction between SA mediated S assimilation and stress defense response. Plants receiving exogenous SA were found with elevated GSH content, along with higher GR activity,Citation25which confirms the interacting role among SA and S. Despite increasing GSH content, SA increases Cys and S content, ATPS and SAT activity,Citation26 while SA homeostasis is regulated by a sulfo-transferase (SOT12) via sulfation.Citation27 Glucosinolate accumulation in plant tissue is adversely affected by SA.Citation28 On the other hand, SA signaling is greatly controlled through S-nitrosylation, hence, require GSH for the activity. Therefore, further research is required for establishing the relation between SA and S metabolism.
The roles of GAs in regulating S assimilation enzymes are crucial for acquired abiotic stress tolerance in plants. Koprivova et al.Citation18 reported that APR transcript levels induction by salinity could be prevented in gai mutant by disrupting GA signaling, but enzyme activity remains unchanged. A recent report suggested that GA and S together decrease oxidative stress by ethylene formation and promoting S use efficiency, photosynthesis and GSH production of Cd stressed plants.Citation29 For the regulation of S assimilation BRs can be modified by sulfation and sulfotransferases, thus exert their role on S metabolism.Citation30
The interplay of S with nitric oxide
Nitric oxide is reported to be involved in decreasing oxidative damage through GSH generation under various abiotic stresses.Citation1 In contrary, S supplementation increase GSH content in plant tissue by increased S assimilation.Citation31 Thus, maybe there is an obvious interaction between S and NO. Nitric oxide also stimulates assimilation of S and react with targeted molecules thus decrease the ROS damage through the enhancement of antioxidant defense. Furthermore, NO interacts in the expression of some stress-responsive genes.Citation32 For establishing the abiotic stress tolerance, NO avowedly or obliquely upregulates different genes connected with redox state of cells.Citation33 The relationship between the antioxidants and NO is mainly lighted upon GSH, which is enormously significant for controlling of ROS.Citation34 As a signaling molecule, NO activity controls S-utilization for GSH production under abiotic stress condition.Citation32 The efficiency of AsA-GSH cycle and antioxidant defense mechanism mainly depends on the GSH content and redox homeostasis.Citation1 Thus NO is vital for stress tolerance in plants.
In the NO metabolic pathway, the reaction between NO or NO derived ONOO− and GSH is vital to form an intermediate S-nitrosoglutathione (GSNO), which can further donate NOCitation35 (). Under abiotic stress condition GSNOR take part in either limiting or alleviating NO levels in cells to tolerate stress.Citation36 The enzyme GSNOR, break down GSNO to release NO and form NH3 and GSSG. Further GSSG is reduced to GSH by the activity of GR and balance redox homeostasis. Hence homeostasis of the enzyme GSNOR is imperative for stress tolerance in plantsCitation37 and vital for regulating downstream ROS and RNS effects.Citation34
A further important interacting role of S exists with NO via H2S – another important signaling molecule in plant physiology. Applying NaHS as an H2S donor to Pb-stressed Sesamum indicum plants increased the NO content in root tissue, which hinted the H2S induced NO signaling in Pb-stress tolerance.Citation38 Li et al.Citation39 also found that the H2S–induced NO generation is essential for Cd tolerance in Medicago sativa. Similar results were also reported by Chen et al.Citation40 in barley seedling roots under salt stress and Li et al.Citation41 under heat stress in Zea mays. Furthermore, exogenous SNP application enhanced the H2S content, suggesting NO as an upstream signaling molecule for H2S production in cells.Citation39 Shi et al.Citation38 observed that the NO-induced Cd tolerance in bermuda grass could be inhibited by both NO and H2S scavengers, and stated that the NO responses were mediated via the H2S signal. Therefore, it can be concluded that both NO and H2S interaction offer plants tolerance to abiotic stress.
Polyamines and S interaction
Putrescine (Put), spermidine (Spd), and spermine (Spm) are the three major PAs originate in plants and are synthesized successively in the same biosynthetic pathway. S-Adenosyl methionine is one of the routs for PA biosynthesis. In this process SAM is decarboxylated with the action of SAM decarboxylase and the intermediate of this reaction is further act as the donor of aminopropyl sub-units for Spd and Spm biosynthesisCitation8 (). Here, Yang cycle functions to restore the S moiety in SAM and regulated by the S accessibility of the cell.Citation42 Therefore, when plants suffer from S deficiency, PA biosynthesis is hampered, and as a result, the concentration of PA decreases.Citation43 Polyamines also take part in S assimilation as well as osmoprotection.Citation17
Interaction with other plant nutrients
Sulfur interacts with all other essential plant nutrients, for instance, nitrogen, potassium (K), phosphorus, magnesium, molybdenum (Mo) and selenium. But among them, SO42− supply alters K, Mo, and zinc (Zn), thus are strongly linked with the S availability. Researchers found a positive correlation between K and S content in shoots highlighted the interacting role of K+ as the counter cation of SO42– during vacuolar storage and xylem loading in leaf tissue.Citation44 It is observed that Se treatment mimics S deficiency to activate specific SO42− transporter expression to stimulate S uptake, resulting in the selenate-induced S accumulation in plants.Citation45
One of the important S-derivative – H2S, has a profound effect on nutrient homeostasis thus conferring abiotic stress tolerance.Citation46 Although H2S is produced in plants in a minor quantity, researchers have identified its nutrient balancing effects using NaHS as H2S source exogenously. In a S-sufficient soil exogenous H2S reduce S uptake by roots, but at S deficit condition, exogenous H2S serves as S source for the normal metabolic process of plants.Citation44 Sulfur deficiency in plants triggers Mo and Zn uptake, but when S is supplied through H2S fumigation, it prevents Mo and Zn uptake.Citation44 Exogenous NaHS enhanced absorption of P, S, Ca, Mg, and iron (Fe) in plants together with reduced the toxic Al accumulation. Moreover, exogenous NaHS increased the activity of H+-ATPase and Na+/K+-ATPase in Hordeum valgure.Citation47
In conclusion, S and their derivatives alone or interactively regulated many defense-related plant processes such as stomatal movement, redox balance, ion homeostasis and antioxidant defense. Glutathione, one of the key components of AsA-GSH cycle, is most important for antioxidant defense mechanism and controlling of ROS under abiotic stress condition. Nitric oxide signaling is largely dependent on S and GSH. On the other hand, S via H2S can induce NO signaling in plants to increase abiotic stress tolerance. The interaction of S with PAs and some plant nutrients have been studied sporadically and therefore, warrants further investigation. This review briefly highlighted the recent progress on the interaction of S with other biomolecules to confer abiotic stress tolerance. However, further researches are necessary to elucidate the interacting role of S with ROS and other plant signals.
Conflicts of interest
There is no potential conflict of interest to disclose.
References
- Hasanuzzaman M, Oku H, Nahar K, Bhuyan MHMB, Mahmud JA, Baluska F, Fujita M. Nitric oxide-induced salt stress tolerance in plants: ROS metabolism, signaling, and molecular interactions. Plant Biotechnol Rep. 2018; doi: 10.1007/s11816-018-0480-0.
- Capaldi FR, Gratão PL, Reis AR, Lima LW, Azevedo RA. Sulfur metabolism and stress defense responses in plants. Trop Plant Biol. 2015; 8:60−73.
- Bashir H, Ibrahim MM, Bagheri R, Ahmad J, Arif IA, Baig MA, Qureshi MI. Influence of sulfur and cadmium on antioxidants, phytochelatins and growth in Indian mustard. AoB PLANTS. 2015; 7: plv001. doi: 10.1093/aobpla/plv001.
- Dixit G, Singh AP, Kumar A, Dwivedi S, Deeba F, Kumar S, Pandey V. Sulfur alleviates arsenic toxicity by reducing its accumulation and modulating proteome, amino acids and thiol metabolism in rice leaves. Sci Rep. 2015; 5: 16205. doi: 10.1038/srep16205.
- Fatma M, Khan MIR, Masood A, Khan NA. Coordinate changes in assimilatory sulfate reduction are correlated to salt tolerance: involvement of phytohormones. Annu Rev Mater Res. 2013; 33: 267–295.
- Li ZG, Min X, Zhou ZH. Hydrogen sulfide: A signal molecule in plant cross-adaptation. Front Plant Sci. 2016; 7: 1621. doi: 10.3389/fpls.2016.01621.
- Corpas FJ, Barroso JB. Reactive sulfur species (RSS): possible new players in the oxidative metabolism of plant peroxisomes. Front Plant Sci. 2015; 6: 116. doi: 10.3389/fpls.2015.00116.
- Sauter M, Moffatt B, Saechao MC, Hell R, Wirtz M. Methionine salvage and S-adenosylmethionine: essential links between sulfur, ethylene and polyamine biosynthesis. Biochem J. 2013; 451: 145–154. doi: 10.1042/BJ20121744.
- Sauter M, Cornell KA, Beszteri S, Rzewuski G. Functional analysis of methylthioribose kinase genes in plants. Plant Physiol. 2004; 136: 4061–4071. doi: 10.1104/pp.104.053587.
- Cao MJ, Wang Z, Zhao Q, Mao JL, Speiser A, Wirtz M, Xiang CB. Sulfate availability affects ABA levels and germination response to ABA and salt stress in Arabidopsis thaliana. Plant J. 2014; 77: 604–615. doi: 10.1111/tpj.12403.
- Kopriva S, Talukdar D, Takahashi H, Hell R, Sirko A, Sf D, Talukdar T. Frontiers of sulfur metabolism in plant growth, development, and stress response. Front Plant Sci. 2016; 6: 1220. doi: 10.3389/fpls.2015.01220.
- Passaia G, Queval G, Bai J, Margis-Pinheiro M, Foyer CH. The effects of redox controls mediated by glutathione peroxidases on root architecture in Arabidopsis thaliana. J Exp Bot. 2014; 65: 1403–1413. doi: 10.1093/jxb/eru348.
- Franco-Zorrilla JM, Martin AC, Leyva A, Paz-Ares J. Interaction between phosphate-starvation, sugar, and cytokinin signaling in Arabidopsis and the roles of cytokinin receptors CRE1/AHK4 and AHK3. Plant Physiol. 2005; 138: 847–857. doi: 10.1104/pp.105.060517.
- Frerigmann H, Gigolashvili T. MYB34, MYB51, and MYB122 distinctly regulate indolic glucosinolate biosynthesis in Arabidopsis thaliana. Mol Plant. 2014; 7: 814–828. doi: 10.1093/mp/ssu004.
- Frerigmann H, Berger B, Gigolashvili T. bHLH05 is an interaction partner of MYB51 and a novel regulator of glucosinolate biosynthesis in Arabidopsis. Plant Physiol. 2014; 166: 349–369. doi: 10.1104/pp.114.240887.
- Park SW, Li W, Viehhauser A, He B, Kim S, Nilsson AK, Andersson MX, Kittle JD, Ambavaram MMR, Luan S, et al. Cyclophilin 20-3 relays a 12-oxo-phytodienoic acid signal during stress responsive regulation of cellular redox homeostasis. Proc Natl Acad Sci USA. 2013; 110: 9559–9564. doi: 10.1073/pnas.1218872110.
- Chan KX, Wirtz M, Phua SY, Estavillo GM, Pogson BJ. Balancing metabolites in drought: the sulfur assimilation conundrum. Trends Plant Sci. 2013; 18: 18–29. doi: 10.1016/j.tplants.2012.07.005.
- Koprivova A, North KA, Kopriva S. Complex signaling network in regulation of adenosine 5′-phosphosulfate reductase by salt stress in Arabidopsis roots. Plant Physiol. 2008; 146: 1408–1420. doi: 10.1104/pp.107.110361.
- Wawrzynska A, Moniuszko G, Sirko A. Links between ethylene and sulfur nutrition—a regulatory interplay or just metabolite association? Front Plant Sci. 2015; 6: 1053. doi: 10.3389/fpls.2015.01053.
- Mao D, Yu F, Li J, Tan D, Li J, Liu Y, Li X, Dong M, Chen L, Li D, et al. FERONIA receptor kinase interacts with S-adenosylmethionine synthetase and suppresses S-adenosylmethionine production and ethylene biosynthesis in Arabidopsis. Plant Cell Environ. 2015; 38: 2566–2574. doi: 10.1111/pce.12570.
- Iqbal N, Masood A, Khan MIR, Asgher M, Fatma M, Khan NA. Cross-talk between sulfur assimilation and ethylene signaling in plants. Plant Signal Behav. 2013; 8: e22478. doi: 10.4161/psb.22478.
- Koprivova A, Kopriva S. Hormonal control of sulfate uptake and assimilation. Plant Mol Biol. 2016; 91: 617–627. doi: 10.1007/s11103-016-0438-y.
- Ernst L, Goodger JQ, Alvarez S, Marsh EL, Berla B, Lockhart E, Jung J, Li P, Bohnert HJ, Schachtman DP. Sulphate as a xylem-borne chemical signal precedes the expression of ABA biosynthetic genes in maize roots. J Exp Bot. 2010; 61: 3395–3405. doi: 10.1093/jxb/erp353.
- Scuffi D, Álvarez C, Laspina N, Gotor C, Lamattina L, García-Mata C. Hydrogen sulfide generated by L-cysteine desulfhydrase acts upstream of nitric oxide to modulate abscisic acid-dependent stomatal closure. Plant Physiol. 2014; 166: 2065–2076. doi: 10.1104/pp.114.245373.
- Pál M, Kovács V, Szalai G, Soós V, Ma X, Liu H, Mei H, Janda T. Salicylic acid and abiotic stress responses in rice. J Agron Crop Sci. 2014; 200: 1–11. doi: 10.1111/jac.12037.
- Nazar R, Umar S, Khan NA. Exogenous salicylic acid improves photosynthesis and growth through increase in ascorbate-glutathione metabolism and S assimilation in mustard under salt stress. Plant Signal Behav. 2015; 10: e1003751. doi: 10.1080/15592324.2014.1003751.
- Baek D, Pathange P, Chung JS, Jiang J, Gao L, Oikawa A, Hirai MY, Saito K, Pare PW, Shi H. A stress−inducible sulphotransferase sulphonates salicylic acid and confers pathogen resistance in Arabidopsis. Plant Cell Environ. 2010; 33: 1383–1392.
- Mikkelsen MD, Petersen BL, Glawischnig E, Jensen AB, Andreasson E, Halkier BA. Modulation of CYP79 genes and glucosinolate profiles in Arabidopsis by defense signaling pathways. Plant Physiol. 2003; 131: 298–308. doi: 10.1104/pp.011015.
- Masood A, Khan MIR, Fatma M, Asgher M, Per TS, Khan NA. Involvement of ethylene in gibberellic acid-induced sulfur assimilation, photosynthetic responses, and alleviation of cadmium stress in mustard. Plant Physiol Biochem. 2016; 104: 1–10. doi: 10.1016/j.plaphy.2016.03.031.
- Marsolais F, Boyd J, Paredes Y, Schinas AM, Garcia M, Elzein S, Varin L. Molecular and biochemical characterization of two brassinosteroid sulfotransferases from Arabidopsis, AtST4a (At2g14920) and AtST1 (At2g03760). Planta. 2007; 225: 1233–1244. doi: 10.1007/s00425-006-0413-y.
- Fatma M, Asgher M, Masood A, Khan NA. Excess sulfur supplementation improves photosynthesis and growth in mustard under salt stress through increased production of glutathione. Environ Exp Bot. 2014; 107: 55–63. doi: 10.1016/j.envexpbot.2014.05.008.
- Fatma M, Masood A, Per TS, Rasheed F, Khan NA. Interplay between nitric oxide and sulfur assimilation in salt tolerance in plants. Crop J. 2016; 4: 153–161. doi: 10.1016/j.cj.2016.01.009.
- Sung CH, Hong JK. Sodium nitroprusside mediates seedling development and attenuation of oxidative stresses in Chinese cabbage. Plant Biotechnol Rep. 2010; 4: 243–251. doi: 10.1007/s11816-010-0138-z.
- Baudouin E, Hancock J. Nitric oxide signaling in plants. Front Plant Sci. 2014; 4: 553. doi: 10.3389/fpls.2013.00553.
- Barroso JB, Corpas FJ, Carreras A, Rodríguez-Serrano M, Esteban FJ, Fernández-Ocana A, Chaki M, Romero-Puertas MC, Valderrama R, Sandalio LM, et al. Localization of S-nitrosoglutathione and expression of S-nitrosoglutathione reductase in pea plants under cadmium stress. J Exp Bot. 2006; 57: 1785–1793. doi: 10.1093/jxb/erj175.
- Corpas FJ, Jdd A, Barroso JB. Current overview of S-nitrosoglutathione (GSNO) in higher plants. Front Plant Sci. 2013; 4: 126. doi: 10.3389/fpls.2013.00126.
- Lee U, Wie C, Fernandez BO, Feelisch M, Vierling E. Modulation of nitrosative stress by S-nitrosoglutathione reductase is critical for thermotolerance and plant growth in Arabidopsis. Plant Cell. 2008; 20: 786–802. doi: 10.1105/tpc.107.052647.
- Shi H, Ye T, Chan Z. Comparative proteomic and physiological analyses reveal the protective effect of exogenous polyamines in the bermudagrass (Cynodon dactylon) response to salt and drought stresses. J Proteom Res. 2013; 12: 4951–4964. doi: 10.1021/pr400479k.
- Li ZG, Gong M, Xie H, Yang L, Li J. Hydrogen sulfide donor sodium hydrosulfide-induced heat tolerance in tobacco (Nicotiana tabacum L.). Suspension Cultured Cells and Involvement of Ca2+ and Calmodulin. Plant Sci.. 2012; 185: 185–189.
- Chen J, Wang WH, Wu FH, He EM, Liu X, Shangguan ZP, Zheng HL. Hydrogen sulfide enhances salt tolerance through nitric oxide-mediated maintenance of ion homeostasis in barley seedling roots. Sci Rep. 2015; 5: 12516. doi: 10.1038/srep12516.
- Zg L, Xj D, Pf D. Hydrogen sulfide donor sodium hydrosulfide-improved heat tolerance in maize and involvement of proline. J Plant Physiol. 2013; 170: 741–747. doi: 10.1016/j.jplph.2012.12.018.
- Bürstenbinder K, Rzewuski G, Wirtz M, Hell R, Sauter M. The role of methionine recycling for ethylene synthesis in Arabidopsis. Plant J. 2007; 49: 238–249. doi: 10.1111/j.1365-313X.2006.02944.x.
- Nikiforova VJ, Kopka J, Tolstikov V, Fiehn O, Hopkins L, Hawkesford MJ, Hesse H, Hoefgen R. Systems rebalancing of metabolism in response to sulfur deprivation, as revealed by metabolome analysis of Arabidopsis plants. Plant Physiol. 2005; 138: 304–318. doi: 10.1104/pp.104.053793.
- Reich M, Shahbaz M, Prajapati DH, Parmar S, Hawkesford MJ, De Kok LJ. Interactions of sulfate with other nutrients as revealed by H2S fumigation of Chinese cabbage. Front Plant Sci. 2016; 7: 541. doi: 10.3389/fpls.2016.00541.
- Boldrin PF, De Figueiredo MA, Yang Y, Luo H, Giri S, Hart JJ, Faquin V, Guilherme LR, Thannhauser TW, Li L. Selenium promotes sulfur accumulation and plant growth in wheat (Triticum aestivum). Physiol Plant. 2016; 158: 80–91. doi: 10.1111/ppl.12465.
- Fotopoulos V, Christou A, Manganaris GA. Hydrogen sulfide as a potent regulator of plant responses to abiotic stress factors. Gaur RK, Sharma P. eds. Molecular Approaches in Plant Abiotic Stress.Boca Raton: CRC Press; 2013. 353–373.
- Dawood M, Cao F, Jahangir MM, Zhang G, Wu F. Alleviation of aluminum toxicity by hydrogen sulfide is related to elevated ATPase, and suppressed aluminum uptake and oxidative stress in barley. J Hazard Mater. 2012; 209: 121–128. doi: 10.1016/j.jhazmat.2011.12.076.