ABSTRACT
Light is an important environmental cue, causing a high degree of developmental plasticity in higher plants. The outcome of light-regulated developmental response is determined by not only photo-sensory systems but also endogenous physiological contexts in plants. KARRIKIN-INSENSITIVE2 (KAI2) functions as a receptor of karrikin and endogenous, as yet to be identified, KAI2 ligand (KL). The loss-of-function of KAI2 caused light-hyposensitive photomorphogenesis, affecting the expression light-responsive genes under the light conditions. However, it remains still unclear how KAI2-KL signaling interacts with light-signaling. Here, we show that the ply2 mutation, a severe loss-of-function allele of KAI2 affected the expression of a subset of light-responsive genes, irrespectively of light condition. The results implied that the overlapping set of light- and KAI2-responsive genes may serve as an integrating node between light- and KAI2-KL signaling. Further, the results of double mutant analyses between the ply2 mutant and mutants of CONSTITUTIVELY PHOTOMORPHOGENIC1 (COP1) or LONG HYPOCOTYL IN FAR-RED (HFR1) implicated that KAI2-KL signaling acts at downstream of COP1, largely independently of HFR1. Together, these results suggest that KAI2-KL signaling intersects with a subset of the light-regulatory network, by which plants adjust their photomorphogenic development.
Text
Plants have evolved to have sophisticated photo-sensory systems, including red/far-red light absorbing phytochromes (Phy), blue light absorbing cryptochromes, phototropins, ZTL/FKF proteins and UVR8, a UV-B receptor.Citation1–Citation3 Upon perception of light, the resulting light-responsive development of plants, collectively termed as photomorphogenesis, contributes to the adaptation to fluctuating light environments. However, the extent of photo-responses is determined not only by photo-sensory systems but also by endogenous or environmental stimuli, so plants can coordinate explicitly their photomorphogenesis in accordance with endogenous developmental and physiological contexts.Citation4 Indeed, diverse cues have been identified that impact photomorphogenesis, which include temperature, nutrients, circadian rhythm, and several plant hormones.Citation5–Citation7 The elegant studies for the past decade have uncovered a mode of crosstalk between light-signaling and hormonal/environmental stimuli, which takes places at multi-layered levels. For example, canonical light-signaling components, which include the photoreceptors themselves and photoreceptor-interacting proteins, are also regulated by temperature and circadian rhythm.Citation5 Another well-characterized mode of signaling crosstalk involves the protein–protein interaction between the components of hormonal/environmental signaling and light-signaling.Citation8 The signal integration is also served by the shared regulatory targets of light signaling and hormonal/environmental signaling.Citation7,Citation9 Despite the progress, it is still in need of more pieces to fill the gaps of the light-regulatory network, which would deepen our understanding of the molecular basis of developmental plasticity in response to light.
KARRIKIN INSENSITIVE2 (KAI2), an α/β hydrolase fold protein, serves as a receptor for karrikin, a smoke compound.Citation10,Citation11 Even in the absence of exogenous karrikin, Arabidopsis kai2 mutants exhibited light-hyposensitive phenotypes, including lower germination rate and longer hypocotyl phenotype under light conditions. This suggests its critical role for photomorphogenesis and the presence of the endogenous ligand, which is termed as KAI2-ligand (KL).Citation10,Citation12–Citation15 However, it remains elusive how KAI2-KL signaling interacts with the light-regulatory network. Recently, we revealed that a subset, if not all, of the light-regulated genes was affected by the ply2 mutation (KAI2ply2), a severe loss-of-function allele of KAI2.Citation15 As presented by Lee et al.,Citation15 for example, in line with the reduced germination of the ply2 mutant under the phytochrome B (PhyB)-dependent condition (),Citation16 a subset of light-responsive genes, which included LATE EMBRYOGENESIS ABUNDANT1 (EM1), LATE EMBRYOGENESIS ABUNDANT6 (EM6), DELAY OF GERMINATION1 (DOG1), FUSCA3 (FUS3), MOTHER OF FT AND TFL1 (MFT), GIBBERELLIN 2 OXIDASE2 (GA2ox2), ABA DEFICIENT1 (ABA1), NINE-CIS-EPOXYCAROTENOID DIOXYGENASE 9 (NCED9), and ABA INSENSITIVE 5 (ABI5) was up-regulated in the ply2 mutant, compared to wild type, under the PhyBon condition, which is 12 h darkness after a pulse of far-red light, followed by a pulse of red light. Interestingly, the ply2 mutation also up-regulated the expression of these genes, compared to wild type, even under the PhyBoff condition, which is 12 h darkness after a pulse of FR light (). Thus, the results illustrate that the effect of the ply2 mutation on the gene expression was not light-conditional. It was also notable that the genes affected by the ply2 mutation retained still light-responsiveness in their expression, when we compared the expression level of each gene under PhyBon condition and that under PhyBoff condition in the ply2 mutant. These results suggested that KAI2-signaling and light-signaling regulated an overlapping set of genes, in a largely additive way, which contribute to light-responsive seed germination.
Figure 1. KAI2 signaling regulates light-dependent germination.
(a) Germination assay of wild type (WT) and ply2 mutant under PhyB-dependent condition as depicted in the diagrams. After sterilization, wild type (WT, Col-0) and the mutant seeds were placed in aqueous media. After irradiation with far-red light (1.33 μW cm−2) for 15 min, and then without (PhyBoff) or with subsequent red light (5.72 μW cm−2) for 10 min (PhyBon), the plates were incubated in darkness. After 4 days, the germination rate was determined based on seedling establishment. Average of germination rate was derived from experimental triplicates with at least 100–200 seeds per plate, respectively. Error bars represent SDs. (b) Gene expression analysis. Total RNA was extracted from 12 h imbibed seeds that were treated as in (A) and subjected to quantitative real-time RT-PCR analysis. PP2A was used as an internal control. Values in the graph represent means ± SDs of the relative expression level of the denoted gene, normalized to that of PP2A (n = 3). Different letters indicate significant differences at P< 0.01, analyzed by one-way ANOVA with a post hoc Tukey HSD test. Part of data for the PhyBon experimental set were reproduced from Lee et al. (2018).
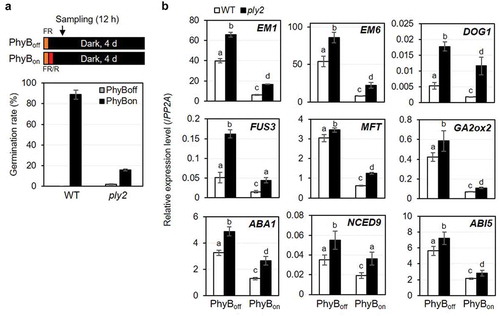
COP1, a RING finger protein, is a key negative regulator of photomorphogenesis.Citation17 In the absence of light, COP1 triggers ubiquitin-mediated protein degradation of several positive regulators of light-signaling such as HY5, LAF1, and HFR1.Citation18 Given with the role of KAI2 as a positive regulator of photomorphogenesis under light conditions,Citation12,Citation15 we examined the genetic hierarchy between COP1 and KAI2 using a double mutant analysis. As shown in , the cop1-6ply2 double mutant displayed more elongated hypocotyl than the cop1-6 single mutant did in darkness, indicating that the ply2 mutation suppressed, if partially, the constitutively photomorphogenic phenotype of cop1-6. The results implied that the photomorphogenic program relieved by the cop1 mutation required functional KAI2 signaling. Intriguingly, exogenously applied karrikin could inhibit the hypocotyl elongation of cop1-6, but not that of wild type in darkness (), which suggested that KAI2 signaling is still functional in the dark-grown seedlings. However, the developmental outcome of KAI2 signaling became evident in the photomorphogenic seedlings, such as light-irradiated wild typeCitation10,Citation15 or the dark-grown cop1 mutant.
Figure 2. Double mutant analysis of ply2 and cop1-6 mutations.
(a) Representative seedling phenotypes. After irradiation with white light for 12 h to stimulate germination, the seedlings were grown on half strength MS media with or without 10 μM of KAR2 (+KAR) for 5 days in darkness. The images show representative 5 d-old dark-grown seedlings. Scale bar, 5 mm. WT, wild type. (b) Quantitative measurement of hypocotyl length. Values represent means ± SDs of hypocotyl length from at least 15 seedlings grown as in (A). WT, wild type. Asterisks indicate significant differences at p < 0.01 (Student t-test).
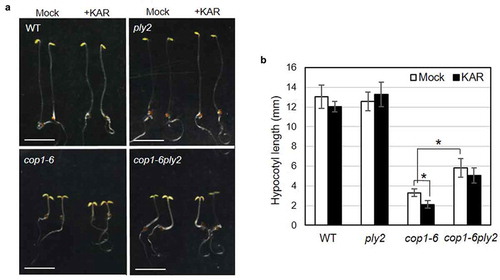
The phenotypes of the cop1-6ply2 double mutant were reminiscent of those of the double mutants between cop1 and the mutant of the light-signaling components such as HY5 and HFR1 which act at downstream of COP1.Citation19 Keeping in mind the hypothesis that KAI2 signaling may converge at downstream of COP1, we tried to address the genetic relationship between KAI2 and HFR1. As shown in , the double mutant hfr1-201ply2 exhibited longer hypocotyl phenotype, compared to its parental lines. The results suggested that HFR1 and KAI2 act largely independently to promote photomorphogenesis.
Figure 3. Double mutant analysis of ply2 and hfr1-201 mutations.
(a) Hypocotyl growth phenotypes under continuous far red light. The seedlings were grown on half strength MS media under far red light (1.33 μW cm−2) for 6 days. Upper, Representative seedling phenotypes. Scale bar, 5 mm. Lower, Values represent means ± SDs of hypocotyl length (n = 15–16). (b) Hypocotyl growth phenotypes under continuous blue light. The seedlings were grown on half strength MS media under blue light (17.4 μW cm−2) for 6 days. Upper, Representative seedling phenotypes. Scale bar, 5 mm. Lower, Values represent means ± SDs of hypocotyl length (n = 12–14). WT, wild type. Different letters indicate significant differences at p< 0.01, analyzed by one-way ANOVA with a post hoc Tukey HSD test.
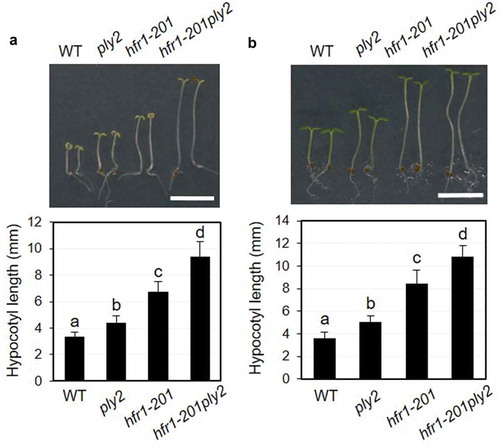
Taking together with our recent findings,Citation15 we proposed that KAI2-KL signaling potentiates photomorphogenesis by regulating a subset of the light-responsive genes. The overlapping set of light-responsive and KAI2-responsive genes may serve as an integrating node, which underpins the crosstalk between light-signaling and KAI2-signaling. Despite the largely additive interaction between KAI2-signaling and light-signaling (),Citation20 the complexity of crosstalk is further added by the findings that HY5 is required for KAI2 expression and KAI2 signaling induces transcriptional expression of HY5.Citation12,Citation20,Citation21 As such, KL, which remains still hypothetical, may represent an important signaling molecule that modulates plant photomorphogenesis. Further, biochemical and genetic identification of KAI2-KL signaling components and KL-biosynthetic pathway would shed light on a new layer of the light-regulatory network, by which plants coordinate their photomorphogenic development.
Acknowledgments
We are thankful for the support of this work by Basic Science Research Program through the National Research Foundation of Korea (NRF) funded by the Ministry of Education (2015R1C1A2A01052950, 2015R1D1A01058039, and 2018R1D1A1B07049914), and by the Rural Development Administration [Next-Generation Biogreen21 program (Agricultural Biotechnology Research Center No. PJ01369001)]. We thank Todd Tate for editorial suggestions for writing manuscript.
Additional information
Funding
References
- Furuya M. Phytochromes: their molecular species, gene families, and functions. Annu Rev Plant Physiol Plant Mol Biol. 1993;44:617–645. doi:10.1146/annurev.pp.44.060193.003153.
- Briggs WR, Huala E. Blue-light photoreceptors in higher plants. Annu Rev Cell Dev Biol. 1999;15:33–62. doi:10.1146/annurev.cellbio.15.1.33.
- Galvão VC, Fankhauser C. Sensing the light environment in plants: photoreceptors and early signaling steps. Curr Opin Neurobiol. 2015;34:46–53. doi:10.1016/j.conb.2015.01.013.
- Gommers CMM, Monte E. Seedling establishment: a dimmer switch-regulated process between dark and light signaling. Plant Physiol. 2018;176:1061. doi:10.1104/pp.17.01460.
- Legris M, Nieto C, Sellaro R, Prat S, Casal JJ. Perception and signalling of light and temperature cues in plants. Plant J. 2017;90:683–697. doi:10.1111/tpj.13467.
- Krahmer J, Ganpudi A, Abbas A, Romanowski A, Halliday KJ. Phytochrome, carbon sensing, metabolism, and plant growth plasticity. Plant Physiol. 2018;176:1039. doi:10.1104/pp.17.01437.
- Nemhauser JL. Dawning of a new era: photomorphogenesis as an integrated molecular network. Curr Opin Plant Biol. 2008;11:4–8. doi:10.1016/j.pbi.2007.10.005.
- Pham VN, Kathare PK, Huq E. Phytochromes and phytochrome interacting factors. Plant Physiol. 2018;176:1025. doi:10.1104/pp.17.01384.
- de Lucas M, Prat S. PIFs get BRright: PHYTOCHROME INTERACTING FACTORs as integrators of light and hormonal signals. New Phytol. 2014;202:1126–1141. doi:10.1111/nph.12725.
- Waters MT, Nelson DC, Scaffidi A, Flematti GR, Sun YK, Dixon KW, Smith SM. Specialisation within the DWARF14 protein family confers distinct responses to karrikins and strigolactones in Arabidopsis. Development. 2012;139:1285–1295. doi:10.1242/dev.074567.
- Guo Y, Zheng Z, La Clair JJ, Chory J, Noel JP. Smoke-derived karrikin perception by the α/β-hydrolase KAI2 from Arabidopsis. Proc Natl Acad Sci. 2013;110:8284–8289. doi:10.1073/pnas.1306265110.
- Sun XD, Ni M. HYPOSENSITIVE TO LIGHT, an alpha/beta fold protein, acts downstream of ELONGATED HYPOCOTYL 5 to regulate seedling de-etiolation. Mol Plant. 2011;4:116–126. doi:10.1093/mp/ssq055.
- Conn CE, Nelson DC. Evidence that KARRIKIN-INSENSITIVE2 (KAI2) receptors may perceive an unknown signal that is not karrikin or strigolactone. Front Plant Sci. 2016;6:7. doi:10.3389/fpls.2015.01219.
- Flematti GR, Waters MT, Scaffidi A, Merritt DJ, Ghisalberti EL, Dixon KW, Smith SM. Karrikin and cyanohydrin smoke signals provide clues to new endogenous plant signaling compounds. Mol Plant. 2013;6:29–37. doi:10.1093/mp/sss132.
- Lee I, Kim K, Lee S, Lee S, Hwang E, Shin K, Kim D, Choi J, Choi H, Cha JS, et al. A missense allele of KARRIKIN-INSENSITIVE2 impairs ligand-binding and downstream signaling in Arabidopsis thaliana. J Exp Bot. 2018;69:3609–3623. doi:10.1093/jxb/ery164.
- Shinomura T, Nagatani A, Chory J, Furuya M. The induction of seed germination in arabidopsis thaliana is regulated principally by phytochrome B and secondarily by phytochrome A. Plant Physiol. 1994;104:363–371.
- Deng XW, Caspar T, Quail PH. COP1 - A REGULATORY LOCUS INVOLVED IN LIGHT-CONTROLLED DEVELOPMENT AND GENE-EXPRESSION IN ARABIDOPSIS. Genes Dev. 1991;5:1172–1182. doi:10.1101/gad.5.7.1172.
- Hoecker U. The activities of the E3 ubiquitin ligase COP1/SPA, a key repressor in light signaling. Curr Opin Plant Biol. 2017;37:63–69. doi:10.1016/j.pbi.2017.03.015.
- Kim YM, Woo JC, Song PS, Soh MS. HFR1, a phytochrome A-signalling component, acts in a separate pathway from HY5, downstream of COP1 in Arabidopsis thaliana. Plant J. 2002;30:711–719.
- Waters MT, Smith SM. KAI2- and MAX2-mediated responses to karrikins and strigolactones are largely independent of HY5 in arabidopsis seedlings. Mol Plant. 2013;6:63–75. doi:10.1093/mp/sss127.
- Nelson DC, Flematti GR, Riseborough JA, Ghisalberti EL, Dixon KW, Smith SM. Karrikins enhance light responses during germination and seedling development in Arabidopsis thaliana. Proc Natl Acad Sci U S A. 2010;107:7095–7100. doi:10.1073/pnas.0911635107.