ABSTRACT
Phytohormones are critical in various aspects of plant biology such as growth regulations and defense strategies against pathogens. Plant–virus interactions retard plant growth through rapid alterations in phytohormones and their signaling pathways. Recent research findings show evidence of how viruses impact upon modulation of various phytohormones affecting plant growth regulations. The opinion is getting stronger that virus-mediated phytohormone disruption and alteration weaken plant defense strategies through enhanced replication and systemic spread of viral particles. These hormones regulate plant–virus interactions in various ways that may involve antagonism and cross talk to modulate small RNA (sRNA) systems. The article aims to highlight the recent research findings elaborating the impact of viruses upon manipulation of phytohormones and virus biology.
1. Introduction
Plant viruses utilize numerous strategies that are more conducive for replication and viral spread inside the plant’s cellular environment.Citation1–Citation5 Phytohormone accumulation and signaling pathways can be directly or indirectly disrupted due to virus infections.Citation6 Several phytohormone pathways exist within plants, contributing to plant growth, reproduction, and development.Citation7,Citation8 Ethylene (Et), jasmonic acid (JA), and salicylic acid (SA) play an important role in mechanisms of defense.Citation9–Citation11 However, abscisic acid (ABA), auxin (Aux), cytokinins (CK), gibberellins (GA),Citation12–Citation16 and brassinosteroids (BR) also show defense relations but play vital roles in plant physiology and development.Citation17–Citation19 Additionally, there is ‘cross talk’ and extensive interactions between different pathways of phytohormones,Citation20,Citation21 providing information to regulate environmental cue responses or attack of pathogens.Citation22–Citation24 Here, we discuss the role of various phytohormones in disease symptom development, host defenses, and enhanced virus replication and movement. We specifically focus upon plant–virus interactions associated with hormonal production, manipulations, and signaling.
2. Mechanisms for symptom development
Plant virus attack exhibits some common symptoms including stunting, curling of leaves, mosaic, mottle, and chlorosis.Citation25,Citation26 Moreover, plant viruses have a great impact on the production of plant hormones leading towards the development of symptoms.Citation22 Despite our understandings about the associations of viral components affecting the phytohormone pathways, our knowledge about their role in the development of symptoms is quite limited.Citation27 Recently, several plant–viral components were found involved in phytohormonal pathways linked to symptom developmentCitation6,Citation28 (). The first mechanistic explanation regarding modulation of virus regulatory systems through phytohormones within their host plants was provided by Wang et al. and Nafisi et al.,Citation46,Citation47 which linked the symptom development to plant–virus interactions. Aux is an important phytohormone that is disrupted directly by viral components.Citation48 Aux signaling disruption largely depends upon the biosynthesis of Aux or mutants showing resembling symptoms to viral disease, i.e., stunting, leaf curling, mosaic, and mottle.Citation49,Citation50 For example, Aux signaling has been shown to disrupt the Tobacco mosaic virus (TMV) 126-kDa replication protein via Aux/indole acetic acid (IAA) family member’s interaction.Citation51,Citation52 Aux/IAA proteins act as negative regulators of Aux-responsive transcription factors (ARFs) to control and modulate genetic ability that is important in different plant functions.Citation53–Citation55 TMV 126 kDa protein interaction disrupts Aux/IAA nuclear-localized proteins and results in the development of diseases such as leaf curling in different plants.Citation29,Citation56 In contrast, TMV-V1087I or a related Tobacco mild green mosaic virus mutant shows no interaction with Aux/IAA proteins and results in symptoms of attenuated disease even though wild-type (WT) plants show replication of these viruses with considerable TMV levels.Citation29,Citation52 Thus, the interaction between the TMV–Aux/IAA is strongly correlated with disease development process.Citation57,Citation58 The viral silencing suppressor’s activity has also been linked to the Aux signaling and disease symptom development.Citation59 Specifically, transgenic Arabidopsis plants constitutively expressing turnip mosaic virus (TuMV) silencing via suppressor HC-Pro exhibit abnormal leaf development resembling the symptoms of viral diseases.Citation30 HC-Pro overexpression was found to increase several miRNA accumulations which include the targeting ARFs. Furthermore, these increased miRNA levels correspond to enhanced target cleavage.Citation30,Citation60–Citation64 Researchers claim that the expression of viral suppressing proteins interfering Aux regulatory pathways accounts for the development of many symptoms induced during viral infections.Citation65 GA biosynthesis pathway disruption is also closely related to symptoms of viral infections. GA takes part in cell division and elongation.Citation66 Stunting and leaf darkening symptoms in GA-deficient rice mutants are characteristically induced by Rice dwarf virus (RDV).Citation67 Specific interaction between the rice ent-kaurene oxidase (EK-O) and the RDV-P2 (an outer capsid protein) has been identified.Citation38 EK-O are also important for the synthesis of GA and can cause rice dwarfing.Citation68,Citation69 Exogenously GA-treated RDV-infected plants exhibited restoration of phenotype of non-dwarf plants, but Aux treatments did not exhibit such results in this regard.Citation38 Virus-directed EK-O-mediated manipulations resulted in symptom development further leading towards the potential interference with the antimicrobial phytoalexin synthesis, thus making the plant more susceptible towards virus infections.Citation48 Viral component/proteins can cause the development of symptoms to cauliflower, e.g., Cauliflower mosaic virus (CaMV) P6 protein.Citation70 Furthermore, this multifunctional P6 viral protein plays a vital role in virus movement, replication, and RNAi suppression.Citation70–Citation72 P6 transgenic expression induces the symptoms like stunting, chlorosis, and banding of veins in leaves.Citation39 Expression of P6 results in interference with the ET pathway’s response; for example, an ET-insensitive phenotype was displayed by P6 transgenic Arabidopsis plants.Citation39 It has been observed that P6 can cause inference with ET-signaling which may produce symptoms.Citation73 However, a direct relationship between P6 and various components of the ET pathway has not yet been identified.
Figure 1. Role of phytohormones in plant–virus interactions. Here, some abbreviations include IAA (indole acetic acid), NRP1 (neuropilin-1), and MeJA (methyl jasmonate). BZR (brassinazole), (p)ppGPP (nucleotides guanosine tetraphosphate and pentaphosphate), ABA (abscisic acid), TuMV (Turnip mosaic virus), CaMV (Cauliflower mosaic virus), RDV (Rice dwarf virus), TMV (Tobacco mosaic virus), CMV (Cucumber mosaic virus), TsWV (Tomato spotted wilt virus), and RBSDV (Rice black-streaked dwarf virus). The articles cited include Refs. Citation29–Citation45.
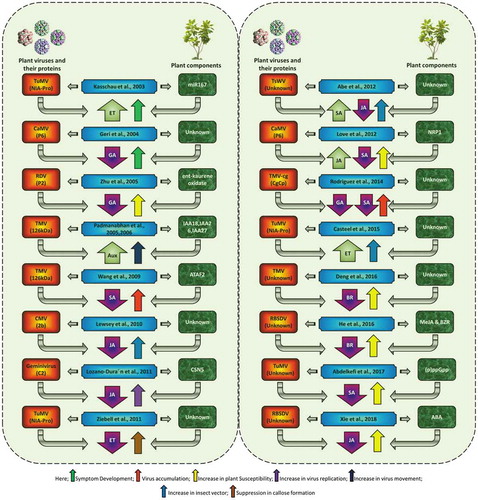
3. Plant defense strategies and phytohormones
Several phytohormones play a crucial role in plant growth, development, and implementation of plant defense strategies against virus infections (). For example, SA regulates basal defense via activating the mechanisms related to systemic acquired resistance (SAR) through R gene.Citation74,Citation75 SA synthesis, activation, and signaling may result in accumulation of reactive oxygen species, callose deposition, activation of pathogenesis-related (PR) proteins, and induction of hypersensitive response.Citation76–Citation78 For activation of SAR, SA is pre-requisite.Citation79 Antagonism has been observed for SA- and JA/ET-mediated defense-related pathways, particularly in case of viruses, insects, and necrotrophs.Citation80,Citation81 However, synergism does exist between these pathways.Citation82 In many studies, it has been explained that SA endogenous depletion or SA signaling disruption leads to defense response impairment and viral infection susceptibility.Citation83–Citation86 For example, SA accumulation reduces salicylate hydrolase via using NahG transgene, further negating the resistance conferred against Potato virus Y (PV-Y) by the potato Ny-1 R-gene.Citation87 Thus, SA synthesis inhibition or SA-dependent defenses are one of the strategies which viruses may use for enhancing their infection. Proteins–virus interactions show its impact upon SA functions. For example, TMV replication protein ATAF2, which targets the degradation of NAC transcription factor domain’s proteasome and regulates plant basal defenses, disrupts SA functions.Citation40,Citation88 Knockout of ATAF2 and SA treatment to repressor lines exhibited a reduction in the level of marker gene, PR1. Moreover, SA treatment does not reveal PR1 production in infected leaves, indicating that plant defense was attenuated because of systemic movement of TMV.Citation88 These results show that TMV-targeted ATAF2 degradation is responsible for the SA-mediated defense suppression. Second, it is proposed that CaMV P6 protein inhibits SA-dependent defense response with the expression, alternation, and localization of SA receptor NPR1.Citation41 P6-expressing plants display a miss localization of an inactive form of NPR1 to the nucleus. This happens via disruption of the SA signaling pathway. It makes P6-expressing plants more resistant to JA but more susceptible to SA-sensitive pathogens, respectively.Citation41 Additionally, current findings with TMV-Cg (a strain of crucifer) have shown that the virus coat protein (CgCP) may suppress signaling of SA by stabilizing of DELLA proteins without altering the SA or JA levels.Citation70 For GA signaling, DELLA proteins negatively regulate and repress the defense SA-mediated defense response, thus displaying antagonism between SA and JA pathways.Citation89,Citation90 Additionally, expression of CgCP also reduces the growth of plants and delays floral transition timing.Citation89 Therefore, JA–SA antagonism is an important factor regarding plant defense against viruses.Citation91 In N gene resistance embedding tobacco lines, exogenously applied methyl jasmonate may cause the reduction of TMV resistance.Citation92 Additionally, silencing of JA receptor COI1 or a JA biosynthetic enzyme allene oxide synthase, resulted in enhanced SA production and reduced TMV accumulations in N gene embedded tobacco lines.Citation92 JA–SA antagonism is also vital during gene expression-mediated defense. For example, RCY1 gene activates the resistance to Cucumber mosaic virus (CMV) via COI1 mutant allele (JA receptor) by minimizing the SA accumulation.Citation77,Citation93 On the contrary, enhanced JA accumulation and its increased levels are not always favorable for plant defense against viral infections. It is reported that increased endogenous JA levels have been incompatible to plant–virus interactions in tobacco and potato.Citation94,Citation95 Additionally, JA exogenous application disrupts infection of geminiviruses.Citation96 Furthermore, the C2 protein of the geminiviruses interacts with COP9 signalosome catalytic subunit, thus compromising the SCF activity of ubiquitin ligase and altering JA regulate ability.Citation97 SCF ubiquitination targets C2 proteins, which modulate plant resistance against geminiviruses through RNA silencing.Citation65
Figure 2. Positive impact of phytohormones on plant defense mechanisms against virus infection. Salicylic acid (SA) signaling exhibits the major defensive pathways through nucleotide-binding leucine-rich repeat (NB-LRR) genes. These defense pathways are triggered by R proteins through SA activation, RNA interference, accumulation of reactive oxygen, and hypersensitive responses. These pathways activate the plant defenses against viral infection where systemically acquired resistance (SAR) and siRNAs are activated by SA at distal sites. Similar to SA, cytokeratins (CKs) and brassinosteroids (BRs) strengthen the plant defense against biotrophs.
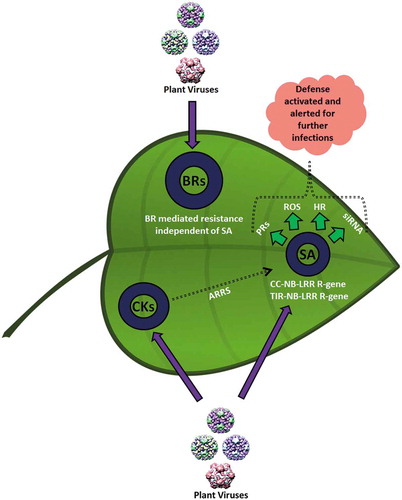
BRs also activate plant defense against viruses.Citation98 Increased resistance against TMV was recorded upon the treatment of WT tobacco plants with brassinolide (BL). No SA accumulation or induction of PR gene expression was observed in BL-treated tobacco plants.Citation99 Furthermore, various geminiviruses exhibit interaction with the BR signaling pathway. For example, BRASSINOSTEROID-INSENSITIVE 2 (BIN2), a negative regulator of BR signaling, interacts with viral C4/AC4.Citation100 But the functional importance of this interaction requires further investigation. Correspondingly, Beat curly type virus (BCTV) C4 protein ectopic expression in Arabidopsis significantly alters plant development via disruption of multiple hormonal pathways.Citation101 Moreover, the LRR-RLK brassinosteroid insensitive-1 (BRI1) that is a BR receptor and PRRs interact with the co-receptor [BRI1-associated kinase 1 (BAK1)] in a ligand-dependent manner. BAK1 is considered essential for plant defense against RNA viruses. For example, bak1-4 and bak1-5 mutants showed higher levels of Turnip crinkle virus (TCV), Oilseed rape mosaic virus (ORMV), and TMV as compared to WT plants.Citation102,Citation103 The mutant plants are hypersensitive to ABA.Citation104 It is thought that enhanced AGO1 (a component of RNA silencing pathway) levels lead to hyposensitivity of ABA.Citation105,Citation106 Additionally, miR168 (AGO1 regulator) contains elements of ABA-response in its promoter region and is upregulated by ABA.Citation107 There is also cross-talk evidence between SA and various pathways of defense through silencing mechanisms.Citation65,Citation108 It was found that NahG expression by plants for reduced SA accumulations lowers the levels of small interfering RNAs (siRNAs) when infected with Plum pox virus (PPV).Citation109 Furthermore, potyvirus silencing suppressor protein HC-Pro overexpression reduced SA-mediated defenses against PPV.Citation110 All these findings suggest that phytohormone signaling and virus defense responses have a strong connection.
4. Virus attacking strategies and phytohormones
Recent research findings demonstrated that phytohormones are directly and indirectly linked to the virus replication and their movement, thus indicating that virus-mediated alterations in hormone levels exhibit negative effects upon plant health (). SA inhibits Tomato bushy stunt virus (TBSV) replication in one system by competitive bindings of cytosolic glyceraldehyde 3-phosphate dehydrogenase (GAPDH).Citation111 Cytosolic GAPDH binds the TBSV-negative RNA strand and has an important role in replication.Citation112 SA accumulations can lead towards its direct binding to GAPDH which actively prevents its interaction to RNA of virus paving the way for TBSV replication suppression.Citation111 Similarly, mutagenesis in ABA2 gene during biosynthesis of ABA resulted in reduction of Bamboo mosaic virus (BMV).Citation113,Citation114 Similarly, failure in replication of CMV was recorded in aba2-1 mutant. This suggests that ABA2 is essential for virus replication or takes part in the translation of complex components.
Figure 3. Negative impact of phytohormones upon plant defenses against viruses. Aux and SA have an antagonistic relationship. Movement of several types of viruses is dependent upon Auxin response factors (ARFs), e.g., TMV. Similarly, Et and SA show antagonism and are actively involved in CaMV symptom development. TMVcg also antagonizes the pathway downstream of SA signaling and is involved in symptom development on Cauliflower mosaic virus (CaMV) infection, systemic movement of TMVcg, and infection establishment. JA and ABA show multiple characteristics regarding plant defenses. For example, at earliest of the virus infection, JA supports plant defense systems, but once the infection is established solely and gets matured, JA production turns towards the favor of virus. ABA antagonizes with the SA pathway leading towards the reduction in resistance at local sites of infection.
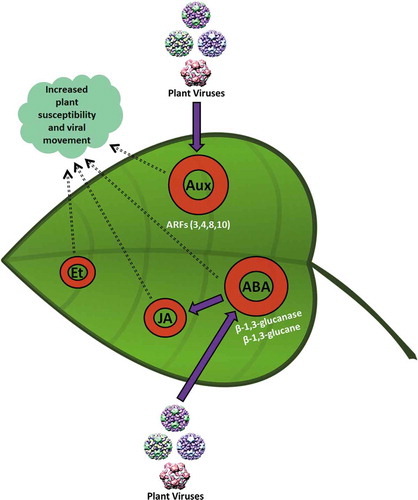
Virus movement from cell-to-cell occurs through the vascular phloem via connections of intercellular plasmodesmata (PD).Citation115 Mounting evidence indicates that in regulating PD connections, several phytohormones play an important role. For example, ABA hinders the movement of viruses through b-1,3-glucanase inhibition which degrades callose.Citation116–Citation118 There is no doubt that callose deposition at PD decreases cell-to-cell viral movement.Citation119,Citation120 Furthermore, ABA exogenous application increases the resistance of plants against viruses such as TMV and Tobacco necrosis virus (TNV) by hindering the virus movement.Citation121–Citation123 Another phytohormone which is closely linked to the closure of PD is SA. Specifically, SA exogenous application results in the deposition of callose within PD.Citation124 This SA-mediated PD closing requires the activity of SA signaling pathways as well as PD gating regulator PDLP5.Citation125,Citation126 SA also inhibits virus movement and replication via another process which requires the involvement of mitochondrial alternative oxidase (AOX) pathway.Citation127,Citation128 Specifically, AOX functions as an inhibitor to counter SA-induced resistance against TMV, PVX, and CMV.
SA has the ability to control extreme resistance (ER), regulated by R genes. ER resembles effector-triggered immunity and completely eliminates the virus infection in the TBSV-resistant tobacco plants.Citation129 Similar examples include the soybean Rsv1-mediated resistance to Soybean mosaic virus (SMV)Citation130 and the Tm-22-mediated resistance against TMV or Tomato mosaic virus (ToMV).Citation131 Sansregret et al. (2013) further observed that an intact SA pathway was required for TBSV resistance in tobacco plants. In tobacco, P19, which is a TBSV viral silencing suppressor (VSR), triggers ER. The consecutive P19 expression resulted in hypersensitive response-like (HR-like) necrosis. From this finding, it was hypothesized that the resistant tobacco plants may have the ability to sense small amounts of P19, which further leads towards the triggering of ER. However, the functionality of P19 due to VSR may result in accumulation of TBSV to the levels, which are sufficient for triggering HR. It suggests that the VSR function of P19 is necessary to induce HR. For example, the P19 mutants who lack in the VSR activity failed to induce HR.Citation129 On a similar note, Arabidopsis SA mutants npr1, sid2 (salicylic acid induction defi-cient2), eds5 (enhanced disease susceptible5), and pad4 (phytoalexin deficient 4) shown higher accumulation of CMV or ORMV as compared to the WT Col-0 plants, thus concluding that SA slowly triggers upon CMV or ORMV infection.Citation132 Correspondingly, Wang et al.Citation133 compared the CP levels of CMV and TCV in eds5 and NahG mutants. The results were similar to previous findings up to 5dpi, but CPs were substantially increased in mutants at 10dpi followed by a gradual decrease after 15 dpi for both viruses. Similar trend of higher Bamboo mosaic virus (BaMV) RNA levels was also observed by Alazem et al.Citation113 in the SA mutants eds1.
SA-mediated viral replication repression is thought to be correlated through siRNA pathway.Citation134,Citation135 For example, P1/HC-Pro tobacco plants carrying NahG accumulated Tomato ringspot virus (ToRSV)-derived sRNAs in lesions.Citation136 More likely, SA treatment increased RNA-dependent RNA polymerase 1 (RDR1) levels in both tobacco and Arabidopsis.Citation122,Citation137,Citation138 However, genes encoding DCLs (dicer-like proteins) seem to be independent of SA-mediated resistance in Arabidopsis, as dcl2, dcl3, and dcl4 mutants exhibited that treating the plants with SA reduced CMV and TMV infection.Citation139 These results suggested that SA can be involved in triggering various DCL redundant mechanisms.Citation139 Interestingly, SA and ToMV infection induced DCL1, DCL2, RDR1, and RDR2 in tomato plants.Citation140 Some reports revealed that SA acts against VSRs. For example, NahG transgenic plants showed higher levels of CMV-2b virus (lacking the CMV2b suppressor) than WT plants.Citation141 Moreover, there are pieces of evidence about strong linkage between siRNA pathways and SA biosynthesis. SA pathways are interfered by viral VSRs. For example, CMV-2b VSR directly affected SA regulations, further reducing the viral symptoms in N. glutinosa.Citation42,Citation142,Citation143
Recently, it is determined that TMV disrupts the selected phloem expressing Aux/IAA gene nuclear localization.Citation52 This vascular-specific IAA regulatory protein disruption was found to be in correlation with the virus ability to load into the phloem and move systemically.Citation144 Therefore, TMV targets the pathway of Aux signaling, thus enhancing its systemic spread and ability to cause disease. Viruses can also move plant-to-plant by many insect vectors which represents another virus biology aspect conferred by phytohormones.Citation145,Citation146 Particularly in plant–insect defenses, JA has been found as a primary hormone responsible for vector transmission. For example, western flower thrips which are vectors for Tomato spotted wilt virus (TSWV) prefer to feed on infected tissues.Citation147,Citation148 SA production is increased, while JA levels are reduced in TSWV-infected plants.Citation149 However, it is still undisclosed whether these pathways are directly targeted by TSWV to enhance insect-mediated virus transmission. Furthermore, CMV-2b silencing suppressor interrupts JA signaling for enhancing vector-(Myzus persicae)-mediated transmission. Moreover, CMV-infected plants or other transgenics expressing 2b exhibited the least levels of JA.Citation43 Additionally, it is interesting to note that aphid survival on CMV-infected tobacco plants (carrying 2b) increases. Oppositely, the survival of aphids decreases when they feed upon the tobacco plants lacking 2b.Citation94 Finally, TuMV NIa-Pro (Nuclear Inclusion–Protease domain) alters the responses of ethylene-suppressing aphid-induced callose defenses. Among potyviruses, NIa-Pro is highly conserved, and researchers propose a conserved mechanism of this interaction for transmission of viruses through aphids.Citation150
5. Concluding remarks
Phytohormones are significant in numerous aspects of plant–virus interactions. Phytohormones have multiple linkages to changes occurred in the accumulation of viruses. It has also been found that cross talk between the pathways of phytohormones is critical for virus defense response regulation. However, despite technological advances in the twenty-first century, we still lack specific information regarding genes that regulate phytohormones and pathways that affect virus biology. Which Aux-regulated genes are responsible for the development of symptoms or which SA-mediated processes impact directly on the accumulation of virus are just one of the few questions that are still unanswered. The answers to these questions will provide a better understanding of the involvement of phytohormones in plant–virus interactions. Furthermore, we should focus on molecular mechanisms that how viruses target and modulate the synthesis of plant hormone and sensing systems to avoid plant defenses, thus enhancing their movement and infection ability.
References
- Islam W, Zhang J, Adnan M, Noman A, Zaynab M, Wu Z. Plant virus ecology: a glimpse of recent accomplishments. Appl Ecol Env Res. 2017;15:691–705. doi:10.15666/aeer/1501_691705.
- Islam W, Zaynab M, Qasim M, Wu Z. Plant-virus interactions: disease resistance in focus. Hosts Virus. 2017;4:5–20. Internet.
- Islam W. Genetic defense approaches against begomoviruses. J Appl Virol Internet. 2017;6:26. doi:10.21092/jav.v6i3.81.
- Islam W, Qasim M, Noman A, Tayyab M, Chen S, Wang L. Management of Tobacco mosaic virus through natural metabolites. Rec Nat Prod Internet. 2018;12:403–415. doi:10.25135/rnp.
- Kuhn M, Neumann S. Differenz und Ungleichheit im Kontext von Mehrsprachigkeit. Differenz - Ungleichheit - Erziehungswissenschaft Internet. 2017;13:275–294. http://link.springer.com/10.1007/978-3-658-10516-7_15.
- Ma KW, Ma W. Phytohormone pathways as targets of pathogens to facilitate infection. Plant Mol Biol. 2016;91:713–725. doi:10.1007/s11103-016-0452-0.
- Michaeli S, Galili G, Genschik P, Fernie AR, Avin-Wittenberg T. Autophagy in plants – what’s new on the menu? Trends Plant Sci. 2016;21:134–144. doi:10.1016/j.tplants.2015.10.008.
- Atamian HS, Harmer SL. Circadian regulation of hormone signaling and plant physiology. Plant Mol Biol. 2016;91:691–702. doi:10.1007/s11103-016-0477-4.
- Bolgova LS, Yaroshuk TM. Histogenesis of lung cancer. Vopr Onkol. 2010;56:469–476.
- Tamaoki D, Seo S, Yamada S, Kano A, Miyamoto A, Shishido H, Miyoshi S, Taniguch S, Akimitsu K, Gomi K. Jasmonic acid and salicylic acid activate a common defense system in rice. Plant Signal Behav. 2013;8:e24260. doi:10.4161/psb.24260.
- Adie B, Chico JM, Rubio-Somoza I, Solano R. Modulation of plant defenses by ethylene. J Plant Growth Regul. 2007;26:160–177. doi:10.1007/s00344-007-0012-6.
- Liu X, Zhang H, Zhao Y, Feng Z, Li Q, Yang H-Q, Luan S, Li J, He Z-H. Auxin controls seed dormancy through stimulation of abscisic acid signaling by inducing ARF-mediated ABI3 activation in Arabidopsis. Proc Natl Acad Sci Internet. 2013;110:15485–15490. doi:10.1073/pnas.1304651110.
- Foo E, Ross JJ, Jones WT, Reid JB. Plant hormones in arbuscular mycorrhizal symbioses: an emerging role for gibberellins. Ann Bot. 2013;111:769–779. doi:10.1093/aob/mct041.
- Ludwig-Müller J. Bacteria and fungi controlling plant growth by manipulating auxin: balance between development and defense. J Plant Physiol. 2015;172:4–12. doi:10.1016/j.jplph.2014.01.002.
- Sauer M, Robert S, Kleine-Vehn J. Auxin: simply complicated. J Exp Bot. 2013;64:2565–2577. doi:10.1093/jxb/ert139.
- Chiang MH, Shen HL, Cheng WH. Genetic analyses of the interaction between abscisic acid and gibberellins in the control of leaf development in Arabidopsis thaliana. Plant Sci. 2015;236:260–271. doi:10.1016/j.plantsci.2015.04.009.
- Saini S, Sharma I, Pati PK. Versatile roles of brassinosteroid in plants in the context of its homoeostasis, signaling and crosstalks. Front Plant Sci Internet. 2015;6. http://journal.frontiersin.org/Article/10.3389/fpls.2015.00950/abstract.
- Rajewska I, Talarek M, Bajguz A. Brassinosteroids and response of plants to heavy metals action. Front Plant Sci Internet. 2016;7. http://journal.frontiersin.org/Article/10.3389/fpls.2016.00629/abstract.
- Cheng X, Gou X, Yin H, Mysore KS, Li J, Wen J. Functional characterisation of brassinosteroid receptor MtBRI1 in medicago truncatula. Sci Rep. 2017;7:9327. doi:10.1038/s41598-017-09297-9.
- Bielach A, Hrtyan M, Tognetti VB. Plants under stress: involvement of auxin and cytokinin. Int J Mol Sci. 2017;18:E1427. doi:10.3390/ijms18071427.
- Pacifici E, Polverari L, Sabatini S. Plant hormone cross-talk: the pivot of root growth. J Exp Bot. 2015;66:1113–1121. doi:10.1093/jxb/eru534.
- Denancé N, Sánchez-Vallet A, Goffner D, Molina A. Disease resistance or growth: the role of plant hormones in balancing immune responses and fitness costs. Front Plant Sci Internet. 2013;4. http://journal.frontiersin.org/article/10.3389/fpls.2013.00155/abstract.
- Aznar A, Chen NWG, Thomine S, Dellagi A. Immunity to plant pathogens and iron homeostasis. Plant Sci. 2015;240:90–97. doi:10.1016/j.plantsci.2015.08.022.
- Nechaeva PA, Natalia VA, Sadriev DS. Transaction costs in the interaction of logistics and marketing in the procurement. Acad Strategic Manage J. 2016;15:59–66.
- Arif M, Lin W, Lin L, Islam W, Jie Z, He Z, Du Z, Wu Z. Cotton leaf curl multan virus infecting Hibiscus sabdariffa in China. Can J Plant Pathol. 2018;40:128–131. doi:10.1080/07060661.2017.1389770.
- Munir M. Management of plant virus diseases; farmer’s knowledge and our suggestions. Hosts Virus. 2017;4:28–33.
- Kumar R, Khurana A, Sharma AK. Role of plant hormones and their interplay in development and ripening of fleshy fruits. J Exp Bot. 2014;65(16):4561–4575.
- Kazan K, Lyons R. Intervention of phytohormone pathways by pathogen effectors. Plant Cell Internet. 2014;26:2285–2309. doi:10.1105/tpc.113.120782.
- Padmanabhan MS, Shiferaw H, Culver JN. The Tobacco mosaic virus replicase protein disrupts the localization and function of interacting Aux/IAA proteins. Mol Plant-Microbe Interact Internet. 2006;19:864–873. doi:10.1094/MPMI-19-0864.
- Kasschau KD, Xie Z, Allen E, Llave C, Chapman EJ, Krizan KA, Carrington JC. P1/HC-Pro, a viral suppressor of RNA silencing, interferes with Arabidopsis development and miRNA function. Dev Cell. 2003;4:205–217. doi:10.1016/S1534-5807(03)00025-X.
- Abe H, Tomitaka Y, Shimoda T, Seo S, Sakurai T, Kugimiya S, Tsuda S, Kobayashi M. Antagonistic plant defense system regulated by phytohormones assists interactions among vector insect, thrips and a tospovirus. Plant Cell Physiol. 2012;53:204–212. doi:10.1093/pcp/pcs042.
- Angel CA, Leisner SM, Nelson RS, Rodriguez A, Schoelz JE, Lutz L. Association of the P6 protein of cauliflower mosaic virus with plasmodesmata and plasmodesmal proteins. Plant Physiol. 2014;166:1345–1358. doi:10.1104/pp.114.249250.
- Casteel CL, De Alwis M, Bak A, Dong H, Whitham SA, Jander G. Disruption of ethylene responses by turnip mosaic virus mediates suppression of plant defense against the green peach aphid vector. Plant Physiol. 2015;169:209–218. doi:10.1104/pp.15.00332.
- Guo H, Yin Y, Lin -H-H, Xi D-H, Zhang D-W, Deng X-G, Zhu T, Peng X-J. Role of brassinosteroid signaling in modulating Tobacco mosaic virus resistance in nicotiana benthamiana. Sci Rep. 2016;6:20579. doi:10.1038/srep20579.
- Chen J, MacFarlane S, Zhu Q, Zhou X, Zhang H, Hong G, Yan F, He Y, Li J, Sun Z. Jasmonic acid-mediated defense suppresses brassinosteroid-mediated susceptibility to rice black streaked dwarf virus infection in rice. New Phytol. 2016;214:388–399.
- Robaglia C, Harchouni S, Ke H, Soubigou-Taconnat L, Sugliani M, Mouille G, Citerne S, Field B, Fakhfakh H, Abdelkefi H. Guanosine tetraphosphate modulates salicylic acid signalling and the resistance of Arabidopsis thaliana to turnip mosaic virus. Mol Plant Pathol. 2017;19:634–646.
- Xie K, Li L, Zhang H, Wang R, Tan X, He Y, Hong G, Li J, Ming F, Yao X, et al. Abscisic acid negatively modulates plant defence against rice black-streaked dwarf virus infection by suppressing the jasmonate pathway and regulating reactive oxygen species levels in rice. Plant Cell Env. 2018;41:2504–2514. doi:10.1111/pce.13372.
- Zhu S, Gao F, Cao X, Chen M, Ye G, Wei C, Li Y. The Rice dwarf virus P2 protein interacts with ent-kaurene oxidases in vivo, leading to reduced biosynthesis of gibberellins and rice dwarf symptoms1. Plant Physiol. 2005;139:1935–1945. doi:10.1104/pp.105.072306.
- Geri C, Love AJ, Cecchini E, Barrett SJ, Laird J, Covey SN, Milner JJ. Arabidopsis mutants that suppress the phenotype induced by transgene-mediated expression of cauliflower mosaic virus (CaMV) gene VI are less susceptible to CaMV-infection and show reduced ethylene sensitivity. Plant Mol Biol. 2004;56:111–124. doi:10.1007/s11103-004-2649-x.
- Wang X, Goregaoker SP, Culver JN. Interaction of the Tobacco mosaic virus replicase protein with a NAC domain transcription factor is associated with the suppression of systemic host defenses. J Virol Internet. 2009;83:9720–9730. doi:10.1128/JVI.02516-08.
- Love AJ, Geri C, Laird J, Carr C, Yun BW, Loake GJ, Tada Y, Sadanandom A, Milner JJ. Cauliflower mosaic virus protein P6 inhibits signaling responses to salicylic acid and regulates innate immunity. PLoS One. 2012;7:e47535. doi:10.1371/journal.pone.0047535.
- Lewsey M, Surette M, Robertson FC, Ziebell H, Choi SH, Ryu KH, Canto T, Palukaitis P, Payne T, Walsh JA, et al. The role of the Cucumber mosaic virus 2b protein in viral movement and symptom induction. Mol Plant-Microbe Interact Internet. 2009;22:642–654. http://apsjournals.apsnet.org/doi/10.1094/MPMI-22-6-0642.
- Groen SC, Moulin M, Powell G, Stevens M, Tungadi T, Ziebell H, Kleczkowski A, Carr JP, Murphy AM, Lewsey MG, et al. Cucumber mosaic virus and its 2b RNA silencing suppressor modify plant-aphid interactions in tobacco. Sci Rep. 2011;1:187. doi:10.1038/srep00187.
- Culver JN, Golem S, Shiferaw H, Padmanabhan MS, Goregaoker SP. Interaction of the Tobacco mosaic virus replicase protein with the Aux/IAA protein PAP1/IAA26 is associated with disease development. J Virol. 2005;79:2549–2558. doi:10.1128/JVI.79.20.12905-12913.2005.
- Lozano-Durán R, Rosas-Díaz T, Luna AP, Bejarano ER. Identification of host genes involved in geminivirus infection using a reverse genetics approach. PLoS One. 2011;6:e22383. doi:10.1371/journal.pone.0022383.
- Nafisi M, Fimognari L, Sakuragi Y. Interplays between the cell wall and phytohormones in interaction between plants and necrotrophic pathogens. Phytochemistry. 2015;112(112):63–71. doi:10.1016/j.phytochem.2014.11.008.
- Wang X, Jiang N, Liu J, Liu W, Wang GL. The role of effectors and host immunity in plant–necrotrophic fungal interactions. Virulence. 2014;5:722–732. doi:10.4161/viru.29798.
- Jin L, Qin Q, Wang Y, Pu Y, Liu L, Wen X, Ji S, Wu J, Wei C, Ding B, et al. Rice dwarf virus P2 protein hijacks auxin signaling by directly targeting the rice OsIAA10 protein, enhancing viral infection and disease development. PLoS Pathog. 2016;12:e1005847. doi:10.1371/journal.ppat.1005847.
- Schaller GE, Bishopp A, Kieber JJ. The Yin-Yang of hormones: cytokinin and auxin interactions in plant development. Plant Cell Online Internet. 2015;27:44–63. doi:10.1105/tpc.114.133595.
- Manojkumar S, Subbaiya R. Isolation and identification of soil derived actinomycetes nocardiopsis alba. Res J Pharm, Biol Chem Sci. 2016;7:485–493.
- Padmanabhan MS, Kramer SR, Wang X, Culver JN. Tobacco mosaic virus replicase-auxin/indole acetic acid protein interactions: reprogramming the auxin response pathway to enhance virus infection. J Virol. 2008;82:2477–2485. doi:10.1128/JVI.01931-07.
- Collum TD, Padmanabhan MS, Hsieh Y-C, Culver JN. Tobacco mosaic virus-directed reprogramming of auxin/indole acetic acid protein transcriptional responses enhances virus phloem loading. Proc Natl Acad Sci Internet. 2016;113:E2740–9. doi:10.1073/pnas.1524390113.
- Yu H, Soler M, Clemente HS, Mila I, Paiva JAP, Myburg AA, Bouzayen M, Grima-Pettenati J, Cassan-Wang H. Comprehensive genome-wide analysis of the Aux/IAA gene family in eucalyptus: evidence for the role of EgrIAA4 in wood formation. Plant Cell Physiol. 2015;56:700–714. doi:10.1093/pcp/pcu215.
- Xie R, Pang S, Ma Y, Deng L, He S, Yi S, Lv Q, Zheng Y. The ARF, AUX/IAA and GH3 gene families in citrus: genome-wide identification and expression analysis during fruitlet drop from abscission zone A. Mol Genet Genomics. 2015;290:2089–2105. doi:10.1007/s00438-015-1063-1.
- Weijers D, Wagner D. Transcriptional responses to the auxin hormone. Annu Rev Plant Biol Internet. 2016;67:539–574. doi:10.1146/annurev-arplant-043015-112122.
- Bargmann BOR, Vanneste S, Krouk G, Nawy T, Efroni I, Shani E, Choe G, Friml J, Bergmann DC, Estelle M, et al. A map of cell type-specific auxin responses. Mol Syst Biol. 2013;9:688. doi:10.1038/msb.2013.40.
- Conti G, Rodriguez MC, Venturuzzi AL, Asurmendi S. Modulation of host plant immunity by Tobamovirus proteins. Ann Bot. 2017;119:737–747.
- Pieterse CMJ, Van der Does D, Zamioudis C, Leon-Reyes A, Van Wees SCM. Hormonal modulation of plant immunity. Annu Rev Cell Dev Biol Internet. 2012;28:489–521. http://www.annualreviews.org/doi/10.1146/annurev-cellbio-092910-154055.
- Wang M-B, Masuta C, Smith NA, Shimura H. RNA silencing and plant viral diseases. Mol Plant-Microbe Interact Internet. 2012;25:1275–1285. http://apsjournals.apsnet.org/doi/10.1094/MPMI-04-12-0093-CR.
- Díaz-Pendón JA, Ding S-W. Direct and indirect roles of viral suppressors of RNA silencing in pathogenesis. Annu Rev Phytopathol Internet. 2008;46:303–326. doi:10.1146/annurev.phyto.46.081407.104746.
- Ghoshal B, Sanfaçon H. Symptom recovery in virus-infected plants: revisiting the role of RNA silencing mechanisms. Virology. 2015;479–480:167–179. doi:10.1016/j.virol.2015.01.008.
- Islam W, Noman A, Qasim M, Wang L. Plant responses to pathogen attack: small RNAs in focus. Int J Mol Sci Internet. 2018;19:515. doi:10.3390/ijms19020515.
- Islam W, Islam SU, Qasim M, Wang L. Host-Pathogen interactions modulated by small RNAs. RNA Biol. 2017;14:891–904. doi:10.1080/15476286.2017.1318009.
- Islam W, Qasim M, Noman A, Adnan M, Tayyab M, Farooq TH, Wei H, Wang L. Plant microRNAs: front line players against invading pathogens. Microb Pathog Internet. 2018;118:9–17. doi:10.1016/j.micpath.2018.03.008.
- Csorba T, Kontra L, Burgyán J. Viral silencing suppressors: tools forged to fine-tune host-pathogen coexistence. Virology. 2015;479–480:85–103. doi:10.1016/j.virol.2015.02.028.
- de Saint Germain A, Ligerot Y, Dun EA, Pillot J-P, Ross JJ, Beveridge CA, Rameau C. Strigolactones stimulate internode elongation independently of gibberellins. Plant Physiol Internet. 2013;163:1012–1025. doi:10.1104/pp.113.220541.
- Satoh K, Shimizu T, Kondoh H, Hiraguri A, Sasaya T, Choi IR, Omura T, Kikuchi S. Relationship between symptoms and gene expression induced by the infection of three strains of Rice dwarf virus. PLoS One. 2011;6:e18094. doi:10.1371/journal.pone.0018094.
- Hayashi KI, Horie K, Hiwatashi Y, Kawaide H, Yamaguchi S, Hanada A, Nakashima T, Nakajima M, Mander LN, Yamane H, et al. Endogenous diterpenes derived from ent-kaurene, a common gibberellin precursor, regulate protonema differentiation of the moss Physcomitrella patens. Plant Physiol Internet. 2010;153:1085–1097. http://www.plantphysiol.org/cgi/doi/10.1104/pp.110.157909.
- Sun TP. The molecular mechanism and evolution of the GA-GID1-DELLA signaling module in plants. Curr Biol. 2011;21:R338–R345. doi:10.1016/j.cub.2011.02.036.
- Rodriguez A, Angel CA, Lutz L, Leisner SM, Nelson RS, Schoelz JE. Association of the P6 protein of cauliflower mosaic virus with plasmodesmata and plasmodesmal proteins. Plant Physiol Internet. 2014;166:1345–1358. doi:10.1104/pp.114.249250.
- Kakumani PK, Ponia SS, Sood V, Chinnappan M, Banerjea AC, Medigeshi GR, Malhotra P, Mukherjee SK, Bhatnagar RK. Role of RNA interference (RNAi) in dengue virus replication and identification of NS4B as an RNAi suppressor. J Virol Internet. 2013;87:8870–8883. http://jvi.asm.org/cgi/doi/10.1128/JVI.02774-12.
- Angel CA, Lutz L, Yang X, Rodriguez A, Adair A, Zhang Y, Leisner SM, Nelson RS, Schoelz JE. The P6 protein of cauliflower mosaic virus interacts with CHUP1, a plant protein which moves chloroplasts on actin microfilaments. Virology. 2013;443:363–374. doi:10.1016/j.virol.2013.04.004.
- Wang W, Esch JJ, Shiu S-H, Agula H, Binder BM, Chang C, Patterson SE, Bleecker AB. Identification of important regions for ethylene binding and signaling in the transmembrane domain of the ETR1 ethylene receptor of arabidopsis. Plant Cell Online Internet. 2006;18:3429–3442. doi:10.1105/tpc.106.044537.
- Gao Q-M, Zhu S, Kachroo P, Kachroo A. Signal regulators of systemic acquired resistance. Front Plant Sci Internet. 2015;06. http://journal.frontiersin.org/article/10.3389/fpls.2015.00228/abstract.
- Fu ZQ, Dong X. Systemic acquired resistance: turning local infection into global defense. Annu Rev Plant Biol. 2013;64:839–863. doi:10.1146/annurev-arplant-042811-105606.
- Voigt CA. Callose-mediated resistance to pathogenic intruders in plant defense-related papillae. Front Plant Sci Internet. 2014;5. http://journal.frontiersin.org/article/10.3389/fpls.2014.00168/abstract.
- Yi SY, Shirasu K, Moon JS, Lee SG, Kwon SY. The activated SA and JA signaling pathways have an influence on flg22-triggered oxidative burst and callose deposition. PLoS One. 2014;9:e88951. doi:10.1371/journal.pone.0088951.
- Luna E, Pastor V, Robert J, Flors V, Mauch-Mani B, Ton J. Callose deposition: a multifaceted plant defense response. Mol Plant-Microbe Interact Internet. 2011;24:183–193. doi:10.1094/MPMI-07-10-0149.
- Ellili A, Rabier J, Prudent P, Salducci MD, Heckenroth A, Lachaâl M, Laffont-Schwob I. Decision-making criteria for plant-species selection for phytostabilization: issues of biodiversity and functionality. J Environ Manage. 2017;201:215–226. doi:10.1016/j.jenvman.2017.06.041.
- Guerreiro A, Figueiredo J, Sousa Silva M, Figueiredo A. Linking jasmonic acid to grapevine resistance against the biotrophic oomycete plasmopara viticola. Front Plant Sci Internet. 2016;7. http://journal.frontiersin.org/Article/10.3389/fpls.2016.00565/abstract.
- Yuan HM, Liu WC, Lu YT. CATALASE2 coordinates SA-mediated repression of both auxin accumulation and JA biosynthesis in plant defenses. Cell Host Microb. 2017;21:143–155. doi:10.1016/j.chom.2017.01.007.
- Yang Y-X, Ahammed G, Wu C, Fan S, Zhou Y-H. Crosstalk among jasmonate, salicylate and ethylene signaling pathways in plant disease and immune responses. Curr Protein Pept Sci Internet. 2015;16:450–461. doi:10.2174/1389203716666150330141638.
- Xu E, Brosché M. Salicylic acid signaling inhibits apoplastic reactive oxygen species signaling. BMC Plant Biol. 2014;14:155. doi:10.1186/1471-2229-14-155.
- Kawagoe Y, Shiraishi S, Kondo H, Yamamoto S, Aoki Y, Suzuki S. Cyclic lipopeptide iturin A structure-dependently induces defense response in Arabidopsis plants by activating SA and JA signaling pathways. Biochem Biophys Res Commun. 2015;460:1015–1020. doi:10.1016/j.bbrc.2015.03.143.
- Lewsey MG, Murphy AM, MacLean D, Dalchau N, Westwood JH, Macaulay K, Bennett MH, Moulin M, Hanke DE, Powell G, et al. Disruption of two defensive signaling pathways by a viral RNA silencing suppressor. Mol Plant-Microbe Interact Internet. 2010;23:835–845. http://apsjournals.apsnet.org/doi/10.1094/MPMI-23-7-0835.
- Carella P, Wilson DC, Cameron RK. Some things get better with age: differences in salicylic acid accumulation and defense signaling in young and mature Arabidopsis. Front Plant Sci Internet. 2015;5. http://journal.frontiersin.org/article/10.3389/fpls.2014.00775/abstract.
- Baebler Š, Witek K, Petek M, Stare K, Tušek-Žnidarič M, Pompe-Novak M, Renaut J, Szajko K, Strzelczyk-Zyta D, Marczewski W, et al. Salicylic acid is an indispensable component of the Ny-1 resistance-gene-mediated response against potato virus y infection in potato. J Exp Bot. 2014;65:1095–1109. doi:10.1093/jxb/eru348.
- Wang X, Culver JN. DNA binding specificity of ATAF2, a NAC domain transcription factor targeted for degradation by Tobacco mosaic virus. BMC Plant Biol. 2012;12:157. doi:10.1186/1471-2229-12-157.
- Rodriguez MC, Conti G, Zavallo D, Manacorda CA, Asurmendi S. TMV-Cg coat protein stabilizes DELLA proteins and in turn negatively modulates salicylic acid-mediated defense pathway during Arabidopsis thaliana viral infection. BMC Plant Biol. 2014;14. doi:10.1186/s12870-014-0210-x.
- Naseem M, Kaltdorf M, Dandekar T. The nexus between growth and defence signalling: auxin and cytokinin modulate plant immune response pathways. J Exp Bot. 2015;66:4885–4896. doi:10.1093/jxb/erv297.
- Robert-Seilaniantz A, Grant M, Jones JDG. Hormone crosstalk in plant disease and defense: more than just jasmonate-salicylate antagonism. Annu Rev Phytopathol Internet. 2011;49:317–343. http://www.annualreviews.org/doi/10.1146/annurev-phyto-073009-114447.
- Oka K, Kobayashi M, Mitsuhara I, Seo S. Jasmonic acid negatively regulates resistance to Tobacco mosaic virus in tobacco. Plant Cell Physiol. 2013;54:1999–2010. doi:10.1093/pcp/pct065.
- Takahashi H, Miller J, Nozaki Y, Sukamto TM, Shah J, Hase S, Ikegami M, Ehara Y, Sp D-K. RCY1, an Arabidopsis thaliana RPP8/HRT family resistance gene, conferring resistance to Cucumber mosaic virus requires salicylic acid, ethylene and a novel signal transduction mechanism. Plant J. 2002;32:655–667.
- Westwood JH, Lewsey MG, Murphy AM, Tungadi T, Bates A, Gilligan CA, Carr JP. Interference with jasmonic acid-regulated gene expression is a general property of viral suppressors of RNA silencing but only partly explains virus-induced changes in plant-aphid interactions. J Gen Virol. 2014;95:733–739. doi:10.1099/vir.0.054783-0.
- Lulai E, Huckle L, Neubauer J, Suttle J. Coordinate expression of AOS genes and JA accumulation: JA is not required for initiation of closing layer in wound healing tubers. J Plant Physiol. 2011;168:976–982. doi:10.1016/j.jplph.2010.12.001.
- Sahu PP, Prasad M. Application of molecular antiviral compounds: novel approach for durable resistance against geminiviruses. Mol Biol Rep. 2015;42:1157–1162. doi:10.1007/s11033-015-3852-3.
- Cavadini S, Fischer ES, Bunker RD, Potenza A, Lingaraju GM, Goldie KN, Mohamed WI, Faty M, Petzold G, Beckwith REJ, et al. Cullin-RING ubiquitin E3 ligase regulation by the COP9 signalosome. Nature. 2016;531:598–603. doi:10.1038/nature17416.
- De Bruyne L, Höfte M, De Vleesschauwer D. Connecting growth and defense: the emerging roles of brassinosteroids and gibberellins in plant innate immunity. Mol Plant. 2014;7:943–959. doi:10.1093/mp/ssu050.
- Nakashita H, Yasuda M, Nitta T, Asami T, Fujioka S, Arai Y, Sekimata K, Takatsuto S, Yamaguchi I, Yoshida S. Brassinosteroid functions in a broad range of disease resistance in tobacco and rice. Plant J. 2003;33:887–898. doi:10.1046/j.1365-313X.2003.01675.x.
- Lai Y. A statistical method for estimating the proportion of differentially expressed genes. Comput Biol Chem. 2006;30:193–202. doi:10.1016/j.compbiolchem.2005.10.002.
- Mills-Lujan K, Deom CM. Geminivirus C4 protein alters Arabidopsis development. Protoplasma. 2010;239:95–110. doi:10.1007/s00709-009-0086-z.
- Kørner CJ, Klauser D, Niehl A, Domínguez-Ferreras A, Chinchilla D, Boller T, Heinlein M, Hann DR. The immunity regulator BAK1 contributes to resistance against diverse RNA viruses. Mol Plant-Microbe Interact Internet. 2013;26:1271–1280. doi:10.1094/MPMI-06-13-0179-R.
- Postma J, Liebrand TWH, Bi G, Evrard A, Bye RR, Mbengue M, Kuhn H, Joosten MHAJ, Robatzek S. Avr4 promotes Cf-4 receptor-like protein association with the BAK1/SERK3 receptor-like kinase to initiate receptor endocytosis and plant immunity. New Phytol. 2016;210:627–642. doi:10.1111/nph.13802.
- Zhang X, Zhang X, Singh J, Li D, Qu F. Temperature-dependent survival of turnip crinkle virus-infected Arabidopsis plants relies on an RNA silencing-based defense that requires DCL2, AGO2, and HEN1. J Virol Internet. 2012;86:6847–6854. doi:10.1128/JVI.00497-12.
- Csorba T, Lózsa R, Hutvágner G, Burgyán J. Polerovirus protein P0 prevents the assembly of small RNA-containing RISC complexes and leads to degradation of ARGONAUTE1. Plant J. 2010;62:463–472. doi:10.1111/j.1365-313X.2010.04186.x.
- Vaucheret H, Mallory AC, Bartel DP. AGO1 homeostasis entails coexpression of MIR168 and AGO1 and preferential stabilization of miR168 by AGO1. Mol Cell. 2006;22:129–136. doi:10.1016/j.molcel.2006.03.011.
- Li W, Cui X, Meng Z, Huang X, Xie Q, Wu H, Jin H, Zhang D, Liang W. Transcriptional regulation of Arabidopsis MIR168a and ARGONAUTE1 homeostasis in abscisic acid and abiotic stress responses. Plant Physiol Internet. 2012;158:1279–1292. doi:10.1104/pp.111.189621.
- Pumplin N, Voinnet O. RNA silencing suppression by plant pathogens: defence, counter-defence and counter-counter-defence. Nat Rev Microbiol. 2013;11(11):745–760. doi:10.1038/nrmicro3120.
- Clemente-Moreno MJ, Hernández JA, Diaz-Vivancos P. Sharka: how do plants respond to Plum pox virus infection? J Exp Bot. 2015;66:25–35. doi:10.1093/jxb/eru428.
- Di Nicola-Negri E, Brunetti A, Tavazza M, Ilardi V. Hairpin RNA-mediated silencing of plum pox virus P1 and HC-Pro genes for efficient and predictable resistance to the virus. Transgenic Res. 2005;14:989–994. doi:10.1007/s11248-005-8925-y.
- Tian M, Sasvari Z, Gonzalez PA, Friso G, Rowland E, Liu X-M, van Wijk KJ, Nagy PD, Klessig DF. Salicylic acid inhibits the replication of tomato bushy stunt virus by directly targeting a host component in the replication complex. Mol Plant Microbe Interact Internet. 2015;28:379–386. doi:10.1094/MPMI-09-14-0259-R.
- Huang T-S, Nagy PD. Direct inhibition of tombusvirus plus-strand RNA synthesis by a dominant negative mutant of a host metabolic enzyme, glyceraldehyde-3-phosphate dehydrogenase, in yeast and plants. J Virol Internet. 2011;85:9090–9102. doi:10.1128/JVI.00666-11.
- Alazem M, Lin K-Y, Lin N-S. The abscisic acid pathway has multifaceted effects on the accumulation of Bamboo mosaic virus. Mol Plant-Microbe Interact Internet. 2014;27:177–189. doi:10.1094/MPMI-08-13-0216-R.
- Toh S, Imamura A, Watanabe A, Nakabayashi K, Okamoto M, Jikumaru Y, Hanada A, Aso Y, Ishiyama K, Tamura N, et al. High temperature-induced abscisic acid biosynthesis and its role in the inhibition of gibberellin action in Arabidopsis seeds. Plant Physiol. 2008;146:1368–1385. doi:10.1104/pp.107.110361.
- Schoelz JE, Harries PA, Nelson RS. Intracellular transport of plant viruses: finding the door out of the cell. Mol Plant. 2011;4:813–831. doi:10.1093/mp/ssr070.
- Oide S, Bejai S, Staal J, Guan N, Kaliff M, Dixelius C. A novel role of PR2 in abscisic acid (ABA) mediated, pathogen-induced callose deposition in Arabidopsis thaliana. New Phytol. 2013;200:1187–1199. doi:10.1111/nph.12436.
- Zavaliev R, Ueki S, Epel BL, Citovsky V. Biology of callose (β-1,3-glucan) turnover at plasmodesmata. Protoplasma. 2011;248:117–130. doi:10.1007/s00709-010-0247-0.
- Zhang H, Shi WL, You JF, M DB, Qin XM, Yu H, Liu Q, Ryan PR, Yang ZM. Transgenic Arabidopsis thaliana plants expressing a β-1,3-glucanase from sweet sorghum (Sorghum bicolorL.) show reduced callose deposition and increased tolerance to aluminium toxicity. Plant, Cell Environ. 2015;38:1178–1188. doi:10.1111/pce.12472.
- Li W, Zhao Y, Liu C, Yao G, Wu S, Hou C, Zhang M, Wang D. Callose deposition at plasmodesmata is a critical factor in restricting the cell-to-cell movement of soybean mosaic virus. Plant Cell Rep. 2012;31:905–916. doi:10.1007/s00299-012-1250-z.
- De Storme N, Geelen D. Callose homeostasis at plasmodesmata: molecular regulators and developmental relevance. Front Plant Sci Internet. 2014;5. http://journal.frontiersin.org/article/10.3389/fpls.2014.00138/abstract.
- Chen L, Zhang L, Li D, Wang F, Yu D. WRKY8 transcription factor functions in the TMV-cg defense response by mediating both abscisic acid and ethylene signaling in Arabidopsis. Proc Natl Acad Sci Internet. 2013;110:E1963–71. doi:10.1073/pnas.1221347110.
- Lee WS, Fu SF, Li Z, Murphy AM, Dobson EA, Garland L, Chaluvadi SR, Lewsey MG, Nelson RS, Carr JP. Salicylic acid treatment and expression of an RNA-dependent RNA polymerase 1 transgene inhibit lethal symptoms and meristem invasion during Tobacco mosaic virusinfection in Nicotiana benthamiana. BMC Plant Biol. 2016;16. doi:10.1186/s12870-016-0796-2.
- Qin L, Mo N, Zhang Y, Muhammad T, Zhao G, Zhang Y, Liang Y. CaRDR1, an RNA-dependent RNA polymerase plays a positive role in pepper resistance against TMV. Front Plant Sci Internet. 2017;8. http://journal.frontiersin.org/article/10.3389/fpls.2017.01068/full.
- Yadav SR, Yan D, Sevilem I, Helariutta Y. Plasmodesmata-mediated intercellular signaling during plant growth and development. Front Plant Sci. 2014;5. doi:10.3389/fpls.2014.00044.
- Wang X, Sager R, Cui W, Zhang C, Lu H, Lee J-Y. Salicylic acid regulates plasmodesmata closure during innate immune responses in Arabidopsis. Plant Cell Internet. 2013;25:2315–2329. doi:10.1105/tpc.113.114959.
- Lim GH, Shine MB, De Lorenzo L, Yu K, Cui W, Navarre D, Hunt AG, Lee JY, Kachroo A, Kachroo P. Plasmodesmata localizing proteins regulate transport and signaling during systemic acquired immunity in plants. Cell Host Microb. 2016;19:541–549. doi:10.1016/j.chom.2016.03.006.
- Chivasa S. Salicylic acid interferes with Tobacco mosaic virus replication via a novel salicylhydroxamic acid-sensitive mechanism [Internet]. Plant Cell Online. 1997;9:547–557. http://www.plantcell.org/cgi/doi/10.1105/tpc.9.4.547.
- Faoro F, Gozzo F. Is modulating virus virulence by induced systemic resistance realistic? Plant Sci. 2015;234:1–13. doi:10.1016/j.plantsci.2015.01.011.
- Sansregret R, Dufour V, Langlois M, Daayf F, Dunoyer P, Voinnet O, Bouarab K. Extreme resistance as a host counter-counter defense against viral suppression of RNA silencing. PLoS Pathog. 2013;9:e1003435. doi:10.1371/journal.ppat.1003435.
- Zhang C, Grosic S, Whitham SA, Hill JH. The requirement of multiple defense genes in soybean Rsv1 –mediated extreme resistance to soybean mosaic virus. Mol Plant-Microbe Interact Internet. 2012;25:1307–1313. doi:10.1094/MPMI-02-12-0046-R.
- Zhang H, Zhao J, Liu S, Zhang DP, Liu Y. Tm-22 confers different resistance responses against Tobacco mosaic virus dependent on its expression level. Mol Plant. 2013;6:971–974. doi:10.1093/mp/sst069.
- Huang Z. Salicylic acid-dependent expression of host genes in compatible Arabidopsis-virus interactions. Plant Physiol Internet. 2005;137:1147–1159. doi:10.1104/pp.104.056028.
- Wang SD, Zhu F, Yuan S, Yang H, Xu F, Shang J, Xu MY, Jia SD, Zhang ZW, Wang JH, et al. The roles of ascorbic acid and glutathione in symptom alleviation to SA-deficient plants infected with RNA viruses. Planta. 2011;234:171–181. doi:10.1007/s00425-011-1391-2
- Nakano K, Ando T, Yamagishi M, Yokoyama K, Ishida T, Ohsugi T, Tanaka Y, Brighty DW, Watanabe T. Viral interference with host mRNA surveillance, the nonsense-mediated mRNA decay (NMD) pathway, through a new function of HTLV-1 Rex: implications for retroviral replication. Microbes Infect. 2013;15:491–505. doi:10.1016/j.micinf.2013.03.006.
- Bouttier M, Saumet A, Peter M, Courgnaud V, Schmidt U, Cazevieille C, Bertrand E, Lecellier CH. Retroviral GAG proteins recruit AGO2 on viral RNAs without affecting RNA accumulation and translation. Nucleic Acids Res. 2012;40:775–786. doi:10.1093/nar/gkr762.
- Jovel J, Walker M, Sanfacon H. Salicylic acid-dependent restriction of tomato ringspot virus spread in tobacco is accompanied with a hypersensitive response, local RNA silencing and moderate systemic resistance. Mol Plant-Microbe Interact Internet. 2011;24:110220083022068. http://apsjournals.apsnet.org/doi/abs/10.1094/MPMI-09-10-0224?url_ver=Z39.88-2003&rfr_id=ori:rid:crossref.org&rfr_dat=cr_pub=pubmed.
- Hunter LJR, Brockington SF, Murphy AM, Pate AE, Gruden K, Macfarlane SA, Palukaitis P, Carr JP. RNA-dependent RNA polymerase 1 in potato (Solanum tuberosum) and its relationship to other plant RNA-dependent RNA polymerases. Sci Rep. 2016;6:23082. doi:10.1038/srep23082.
- Rakhshandehroo F, Rezaee S, Palukaitis P. Silencing the tobacco gene for RNA-dependent RNA polymerase 1 and infection by potato virus Y cause remodeling of cellular organelles. Virology. 2017;510:127–136. doi:10.1016/j.virol.2017.07.013.
- Lewsey MG, Carr JP. Effects of DICER-like proteins 2, 3 and 4 on Cucumber mosaic virus and Tobacco mosaic virus infections in salicylic acid-treated plants. J Gen Virol. 2009;90:3010–3014. doi:10.1099/vir.0.009381-0.
- Campos L, Granell P, Tárraga S, López-Gresa P, Conejero V, Bellés JM, Rodrigo I, Lisón P. Salicylic acid and gentisic acid induce RNA silencing-related genes and plant resistance to RNA pathogens. Plant Physiol Biochem. 2014;77:35–43. doi:10.1016/j.plaphy.2014.01.016.
- Ji L-H, Ding S-W. The suppressor of transgene RNA silencing encoded by Cucumber mosaic virus interferes with salicylic acid-mediated virus resistance. Mol Plant-Microbe Interact Internet. 2007;14:715–724. http://apsjournals.apsnet.org/doi/10.1094/MPMI.2001.14.6.715.
- Zhang X, Yuan YR, Pei Y, Lin SS, Tuschl T, Patel DJ, Chua NH. Cucumber mosaic virus-encoded 2b suppressor inhibits Arabidopsis argonaute1 cleavage activity to counter plant defense. Genes Dev. 2006;20:3255–3268. doi:10.1101/gad.1495506.
- Der WK, Empleo R, Nguyen TTV, Moffett P, Sacco MA. Elicitation of hypersensitive responses in Nicotiana glutinosa by the suppressor of RNA silencing protein P0 from poleroviruses. Mol Plant Pathol. 2015;16:435–448. doi:10.1111/mpp.12201.
- Wan J, Cabanillas DG, Zheng H, Laliberté J-F. Turnip mosaic virus moves systemically through both phloem and xylem as membrane-associated complexes. Plant Physiol Internet. 2015;167:1374–1388. doi:10.1104/pp.15.00097.
- Islam W, Akutse KS, Qasim M, Khan KA, Ghramh HA, Idrees A, Latif S. Bemisia tabaci-mediated facilitation in diversity of begomoviruses: evidence from recent molecular studies. Microb Pathog. 2018;123:162–168. doi:10.1016/j.micpath.2018.07.008.
- Islam W, Lin W, Islam SU, Arif M, Li X, Yang Y, Ding X, Du Z, Wu Z. Genetic diversity of begomoviruses in Pakistan captured through a vector based survey. Microb Pathog Internet. 2018;118:91–97. doi:10.1016/j.micpath.2018.03.019.
- Tomitaka Y, Abe H, Sakurai T, Tsuda S. Preference of the vector thrips Frankliniella occidentalis for plants infected with thrips-non-transmissible tomato spotted wilt virus. J Appl Entomol. 2015;139:250–259. doi:10.1111/jen.2015.139.issue-4.
- Macharia I, Backhouse D, Skilton R, Ateka E, Wu SB, Njahira M, Maina S, Harvey J. Diversity of thrips species and vectors of tomato spotted wilt virus in tomato production systems in Kenya. J Econ Entomol. 2015;108:20–28. doi:10.1093/jee/tou010.
- López-Gresa MP, Lisón P, Yenush L, Conejero V, Rodrigo I, Bellés JM. Salicylic acid is involved in the basal resistance of tomato plants to citrus exocortis viroid and tomato spotted wilt virus. PLoS One. 2016;11:e0166938. doi:10.1371/journal.pone.0166938.
- Revers F, García JA. Molecular biology of potyviruses. Adv Virus Res. 2015;92:101–199.