ABSTRACT
Priming for better defense performance is an important strategy in acclimation to the ever-changing environment. In the present study, defense priming induced by sodium chloride at the seedling stage significantly increased the expression of defense gene VSP2, the content of total glucosinolates and the level of the reactive oxygen species in mature Arabidopsis thaliana plants after transferred into the stress-free environment. The previously primed plants could effectively resist the feeding of Spodoptera litura (Fabricius) larvae. Salt-priming enhanced defense of Arabidopsis plants in the absence of either MYC2 or AOS, which encodes a critical transcription factor in JA-signaling and an important enzyme in JA biosynthesis, respectively. Our results supported the JA-independent defense primed by sodium chloride, as well as the elevated ROS and glucosinolate level in primed plants. In addition, the feasibility of using mild salt-priming to improve crop performance in field was proposed.
Introduction
Herbivory by insect larvae causes tremendous loss of crop yield and is the main threat to food supply around the world. Different strategies, such as application of pesticide, conventional breeding, and genetic engineering have been employed aiming at reducing the loss caused by insect pest in crops.Citation1 In pursuit of safer pest control techniques, many studies propose that priming treatments to enhance plant defense against stresses is highly promising. Compared to non-primed plants, primed plants are provided better leverage with acquired ability to resist future abiotic or biotic stresses.Citation2,Citation3 The protective responses of plants in a priming state will be faster or stronger when a stress is encountered.Citation4–Citation7
A growing body of literature indicates that plants primed by chemical inducers are effectively resistant to insects. In response to herbivores damaging a single leaf, the whole plant releases a bouquet of volatiles, which induce the neighboring plants into a priming state and enable plants to respond more effectively when challenged with secondary attack.Citation8 The non-protein amino acid β-aminobutyric acid (BABA) is known as a potent agent to prime plants against herbivores, such as tobacco hornworms (Manduca sexta), green peach aphids (Myzus persicae), pea aphids (Acyrthosiphon pisum), and spider mites (Tetranychus urticae).Citation9–Citation12 Soil or foliar application of silicon enhances resistance of plants to insect herbivores and other arthropods.Citation13
Sodium chloride (NaCl), a low-cost inorganic compound, is also used as a priming agent. Priming chickpea seeds with NaCl increased the activities of amylase, invertases, sucrose synthase and sucrose phosphate synthase in shoots of primed seedlings.Citation14 Alfalfa seeds primed with salt solution showed increased seed germinative vigor under salinity stress.Citation15 Cakile maritima seeds imbibed in high salinity (200 mM NaCl) showed higher germination percentage and faster germination than the mock-treated seeds.Citation16 Cakile maritima plants pre-exposed to salinity preserved a lower oxidative stress when subsequently exposed to salinity and maintain constant tolerance to salt stress.Citation17 Arabidopsis plants exposed to repetitive salt stress were more resistant to virulent bacteria than those grown in a more stable environment.Citation18
Upon chewing herbivory, the performance of attacked plants is orchestrated by plant hormones, which largely depends on the biosynthesis and signaling of jasmonates (JA).Citation19–Citation21 Allene oxide synthase (AOS) is a key enzyme that catalyzes the formation of allene oxides from fatty acid hydroperoxides generated by lipoxygenases (LOXs), which is essential for JA synthesis.Citation22,Citation23 MYC2 is a basic helix-loop-helix (bHLH) transcription factor that directly regulates expression of JA-responsive genes including VSP2.Citation24 A functional MYC2 is required for intact JA signaling and JA-mediated defense against herbivory.Citation25,Citation26
Though many reports focused on NaCl-induced priming, few of them reported that priming induced by NaCl at seedling stage could impart and maintain defense “memory” against insect herbivores in grown-up plants. Moreover, the relationship between NaCl-induced defense priming and the JA-signaling pathway should be reconsidered as full-scale activation of the JA-induced defense often disrupts the balance between defense and growth. As shown in Figure S1 in Supplmentary Material, VSP2 expression was activated after primed by a set of NaCl at different concentrations (0, 50, 100, 150 mM). In this study, the defensive performance including resistance against herbivory and VSP2 expression in wild-type (Col-0), aos and myc2 backgrounds was investigated. To study the physiological effects of priming, the total glucosinolates (GSL) level and expression of biosynthesis genes SUR1 and FMO-OX3 were profiled. In addition, ROS accumulation and gene expression involved in ROS biogenesis and scavenging were investigated. Collectively, the results showed that salt-priming during seedling stage increased ROS level and GSL content even in adult plants. Salt-priming also mounted long-lasting defense against Spodoptera litura (Fabricius) larvae in adult plants, which was probably independent on MYC2- and AOS-controlled JA signaling pathway.
Materials and methods
Plant materials and priming treatment
Arabidopsis thaliana wild-type Col-0 and two knock-out mutants of critical genes in jasmonate pathway (aos, TAIR stock number: CS6149; myc2, TAIR stock number: SALK_083483) were used in the experiments. The block of JA biosynthesis in aos was reportedCitation22 and the knock-out of MYC2 was demonstrated in the supplementary materials (Figure S2 in Supplmentary Material; Table S1 in Supplmentary Material). The seeds were soaked in 75% alcohol for 10 min and rinsed three times with sterilized water. Then the surface-sterilized seeds were laid onto the MS medium supplemented with 100 mM NaCl as priming treatment (primed) or without NaCl as the control (non-primed). After stratified for 72 h at 4°C, the petri dishes with seeds were transferred to a walk-in growth chamber. The 10-day-old primed and non-primed seedlings were transplanted into autoclaved soil (PindstrupTM, Denmark). Unless stated otherwise, the grown conditions were 23 ± 1°C, 65 ± 5% relative humidity, light intensity of 180 μM photons m−2s−1 and a 16/8 h photoperiod (light/dark). Three weeks after transplanted, eight-rosette-leave plants from primed and non-primed seedlings were subjected to subsequent assays.
Bioassay of resistance to herbivory
The eggs of S. litura (Fabricius), a herbivore causing severe defoliation of host plants,Citation27 were purchased (Baiyun Industrial Co., Ltd, Jiyuan, Henan, China) and reared on artificial diet under a 12 h light/12 h dark regime at 25 ± 1°C with 65 ± 5% relative humidity. For the larval feeding assay, two second-instar larvae were transferred onto the leaves of primed or non-primed plants three weeks after transplantation. Four days after infestation, each larva was weighed and the degree of foliar damage was analyzed by the software ImagJ.Citation28 The ion leakage of leaves after damage was measured by an electrolytic conductivity meter (AR8011, SmartSensorTM, Dongguan, China) following the procedures with minor modifications.Citation29 Briefly, 0.5 g rosette leaves were collected and immersed in 10 ml of de-ionized water for 5 min. The water was subjected to electrical conductivity measurement. The values of the conductivity obtained from the sensor of the electrical conductivity meter were recorded and directly used to indicate the electrolyte leakage in damaged leaves.
Gene expression analyses by RT-qPCR
Approximately 0.1 g leaf tissue was subjected to total RNA isolation using the Trizol reagent (Invitrogen) according to the manufacturer’s instructions. After treated with RNase-Free DNase (Promega) to remove any DNA contamination, 2 μg total RNA was converted into cDNA using a RevertAid First Strand cDNA Synthesis kit (Thermo Fisher Scientific). Three technical replicates of qPCR were performed on a CFX96 Real-Time PCR System C1000 Touch Thermal Cycler (Bio-RAD) using the PowerUpTM SYBRTM Green Master mix (Applied Biosystems) according to the manufacturer’s instructions (http://thermofisher.com/support). Two-step PCR was carried out with 40 cycles of denaturation at 95°C for 15 s and annealing/extension at 60°C for 60 s following an initial denaturation of 2 min at 95°C. This program was followed by a melting curve analysis. The relative expression level of each gene was calculated by the ΔΔCt method using ACTIN2 (At3g18780) as internal reference genes.Citation30,Citation31 The relative gene expression level was averaged over three biological replicates. Data was analyzed using the Student’s t test. The qPCR primer sequences of all analyzed genes are available as supporting information in Table S2 in Supplmentary Material.
Histochemical analysis of reactive oxygen species
Leaves from primed and non-primed Arabidopsis were collected and subjected to 3,3′-Diaminobenzidine (DAB) staining to visualize the accumulation of hydrogen peroxide (H2O2). After incubation, the stained samples were boiled for 10 min and bleached in fresh bleaching solution (ethanol/acetic acid/glycerol = 3:1:1, v/v/v). The stained samples were observed under stereoscopic microscope (Nikon stereoscope SMZ1000) or recorded by photographing. H2O2 content in leaves of primed or nonprime plants were quantified as describedCitation32 with a spectrometer (NanoDrop 2000, Thermo ScientificTM).
Measurement of total glucosinolates by high-performance liquid chromatography (HPLC)
The measurement of total glucosinolates was modified based on the published procedures.Citation33 Briefly, 200 mg leaves from eight-rosette-leave plants were ground into fine powder then extracted with 2 ml of boiling 70% methanol for about 5 min. The mixture was then centrifuged at 12,000× g at 4°C for 15 min. The supernatant was collected and passed through 0.22 μM syringe filters and then loaded onto an anion-exchange column (DEAE-Sephadex A-25) treated overnight with aryl-sulfatase to convert the glucosinolates to their desulfated derivatives. After the conversion procedure, the desulfated glucosinolates were then eluted from the column with 70% (v/v) methanol. The eluent was evaporated to dryness under nitrogen at 45°C and resuspended in water. The desulfated glucosinolates were analyzed by high-performance liquid chromatography (HPLC) with the Agilent Infinity 1260 platform. The HPLC parameters were as follows: column, DiKMA C18 (Diomosil C18, 5 μm, 250 mm, 4.5 mm); mobile phase flow rate, 1 mL/min. Mobile phase parameters were programmed as follows: a linear gradient from 1.25% to 5% acetonitrile (v/v) over 5 min, a linear gradient from 7% to 8% acetonitrile (v/v) over the 2 min, a linear gradient from 8% to 30% acetonitrile (v/v) for 10 min, a linear gradient from 30% to 92% acetonitrile (v/v) over 3 min, constant at 92% acetonitrile (v/v) for 5 min followed by a linear gradient from 92% to 1.25% acetonitrile (v/v) over 1.5 min, then constant at 1.25% acetonitrile (v/v) for 5 min. Desulfated GSL was detected at A229 nm in a HPLC-DAD system. The GSL was quantified by comparison with 2-propenyl glucosinolate (sinigrin) as an internal standard. All tests were replicated three times.
Statistical analysis
Data were averaged from at least three independent biological replicates, and the data on various parameters were subjected to statistical analysis using GraphPad Prism 7 (https://www.graphpad.com). A one-way analysis of variance (ANOVA) was performed, and differences in means were separated using Dunnett’s multiple comparison test at a 1% level of significance. All data are presented as mean ± standard error.
Results
Salt-priming enhanced defense against larval feeding in adult plants
The leaf damage degree caused by larval feeding of primed plants was significantly lower compared to that of non-primed plants (P < .01) (,). The dry weight of larvae feeding on primed plants was decreased by 32% compared to that of larvae feeding on non-primed plants (), suggesting that the growth of larvae feeding on the plants primed by NaCl was inhibited. In the foliar ion leakage assay after feeding, the leakage in primed plants was 27.1% lower compared to that of non-primed plants (Figure S3 in Supplementary Material). The results showed that NaCl-induced priming could effectively protect Arabidopsis plants against S. litura. Previous exposure to NaCl imparted long-lasting defense ability even after Arabidopsis plants were transplanted into a stress-free environment.
Figure 1. Sodium chloride primes Arabidopsis plants against Spodoptera litura larval feeding.
Arabidopsis eight-rosette-leave plants were fed by second instar larvae of Spodoptera litura (Fabricius). (a) Primed plants and non-primed plants on the fourth day after infestation. (b) Rosette leaf damage caused by larval feeding in primed plants and non-primed plants. (c) Larval growth assessment after feeding on primed plants and non-primed plants. The scale bar represents 5 mm. Ten larvae were used to calculate mean values and standard deviations. Feeding assay was replicated above 3 times. Error bars indicate the standard deviation. ** differences are highly significant (P < .01) by Dunnett’s multiple comparison test. dai: days after infection by larvae. NP: non-primed plants; P: primed plants.
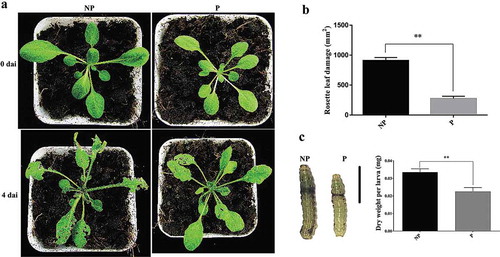
The relationship between NaCl-elicited defense priming and JA pathway
It is known that JA biosynthesis and JA signaling pathway play important roles in plant resistance to insect feeding.Citation19–Citation21 To test whether an intact JA pathway is required for NaCl-primed defense against S. litura, the defensive performance against herbivory in aos and myc2 mutants was investigated. Compared to the non-primed plants, leaf damage caused by feeding was significantly decreased in plants that underwent salt-priming at their seedling stage (, ; Figure S3 in Supplmentary Material). Even in the aos and myc2 mutant plants, the defoliation degree was also significantly decreased by salt-priming. Bioassay results showed the growth of larvae was also effectively inhibited after feeding on primed plants of Col-0, aos or myc2 plants despite the different genetic backgrounds (, ). In addition, the expression levels of the VSP2 marker gene in primed plants increased by above 700% compared with that in plants from non-primed seedlings in both wild-type and mutant back grounds (). The results suggested that NaCl-induced defense priming against larval feeding is probably independent of MYC2- and AOS-controlled branches in the JA pathway.
Figure 2. Primed defense responses of Arabidopsis aos and myc2 mutants.
Arabidopsis eight-rosette-leave plants were fed by second instar larvae of Spodoptera litura (Fabricius). (a) Primed plants and non-primed plants on the fourth day after infestation. (b) Rosette leaf damage measurement by ImageJ. (c) Larvae on the fourth day after infestation; the scale bar represents 5 mm. (d) Larval growth assessment after feeding on primed plants and non-primed plants. Ten larvae were used to calculate mean values and standard deviations. Feeding assay was replicated above 3 times. (e) Relative expression levels of VSP2 in primed plants and non-primed plants in wild type Col-0 and two mutants, aos and myc2. Error bars indicate the standard deviation. ** differences are highly significant (P< .01) by Dunnett’s multiple comparison test. dai: days after infection by larvae. NP: non-primed plants; P: primed plants.
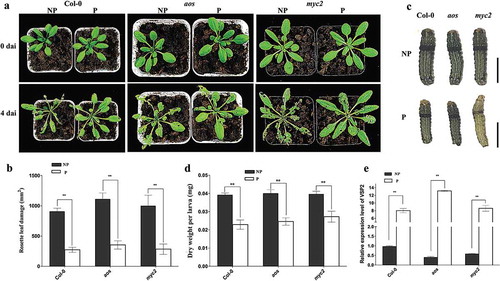
ROS and GSL level was increased by salt-priming
ROS accumulation is a common feature of many plant defense responses,Citation34 and glucosinolates (GSL) are a class of defensive secondary metabolites.Citation35,Citation36 In this respect, salt-priming induced ROS and total GSL levels in Col-0 as well as expression of the genes involved in the relevant metabolic processes were investigated. As visualized by DAB staining and quantified with KI, respectively, primed plants accumulated more hydrogen peroxide (H2O2) compared to non-primed plants at seedling stage. Interestingly, after transplanted into a stress-free environment, H2O2 level persisted in eight-rosette-leave stage plants, which might be indicative of defensive priming state (, ). RT-qPCR results showed that the expression of RBOHA, RBOHB and RBOHC related to ROS production was increased by more than 30% and the expression levels of genes SOD2 and CAT1 related to ROS scavenging also increased by more than 100% (), which suggested ROS synthesis and degradation might be dynamically regulated and the maintaining the intracellular ROS homeostasis to support its defensive role and avoid cellular toxicity was also necessary. The amount of GSL was increased by 31% by salt-priming. The expression level of key genes SUR1 and FMOGS-OX3 related to GSL synthesis in plants undergoing salt-priming increased significantly as compared to that in non-primed plants (, ), suggesting that salt-priming modulated defensiveness in primed plants by maintaining higher GSL level.
Figure 3. ROS and GSL in non-primed and primed plants.
(a) DAB staining of leaves from Arabidopsis seedlings and mature plants with (P) or without (NP) 100 mM NaCl for 10 days; DAB staining of non-primed or primed plants at eight-rosette-leave stage. The scale bar represents 1 mm. (b) H2O2 content in non-primed plants. (c) Relative expression levels of RBOHA, RBOHB, RBOHC, SOD2 and CAT1 in non-primed and primed Arbidopsis at eight-rosette-leave stage. (d) Total glucosinolate content in Arabidopsis at eight-rosette-leave satge. (e) The expression of key genes SUR1 and FMO (FMOGS-OX3) related to glucosinolate synthesis in leaves of non-primed and primed plants at eight-rosette-leave stage. All tests were replicated three times. Error bars indicate the standard deviation. **differences are significant (P < .01) by Dunnett’s multiple comparison test.
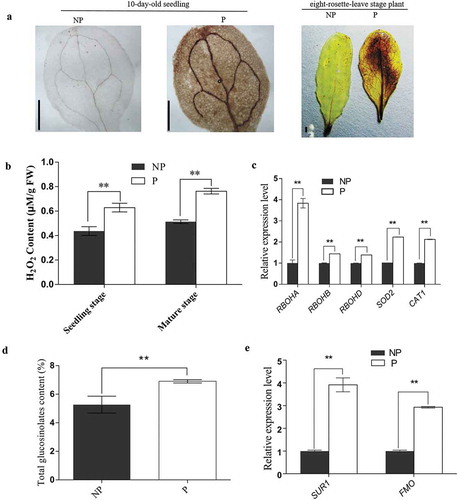
Discussion
Priming has been well documented and proposed as an important strategy to increase the vitality of plants under adverse conditions.Citation2–Citation16 Several studies suggested that NaCl as a priming agent could effectively enhance plant resistance to abiotic stresses.Citation25–Citation29 Owing to its ionic properties, NaCl is commonly regarded as a hazardous factorCitation37 causing salinity stress and less used as a priming agent for protecting plants against impending biotic/abiotic stresses.
Here in this study, priming induced by NaCl effectively enhanced defense against larval feeding (), activated ROS accumulation and increased the glucosinolates content (). ROS is a universal cellular signal in many organisms while glucosinolates are known to be exclusively produced by Brassicaceae plants. Genes as ROS producers including RBOHA (AT5G07390), RBOHB (AT1G09090) and RBOHC (AT5G47910)Citation38,Citation39 and scavengers including SOD2 (AT2G28190) and CAT1 (AT1G20630)Citation40,Citation41 were both upregulated by salt-priming, suggesting even though the ROS level was elevated, the burst of ROS was monitored to avoid excessive cytotoxicity. Expression of SUR1 (AT2G20610) and FMOGS-OX3 (AT1G62560) was upregulated in primed plants, which might be causative of the increased GSL content regarding their important roles in GSL biosynthesisCitation35. Both ROS burst and glucosinolates accumulation are believed to be representative of defensive behaviors induced by biotic stresses.Citation36,Citation41 Accumulation of ROS induced expression of genes involved in glucosinolate biogenesisCitation42. In return, breakdown products of glucosinolates reduced the level of ROS and programmed cell death in ArabidopsisCitation43. In another report, breakdown products of glucosinolates increased peroxidase-mediated reactive oxygen species productionCitation44. According to our results, ROS and GSL accumulation were simultaneously triggered by salt-priming. Due to the antioxidant and anti-insect roles of breakdown products of glucossinolate, glucosinolates might act as an intermediator to balance the defense and cytotoxicity. Thus, it is likely that ROS and glucosinolates are not absolutely independent but instead, their might act synergistically or antagonistically in certain situations.
Previous studies have shown that the JA signaling pathway plays a key role in plant resistance to insect feeding, and the mutation of key genes in the pathway will lead to impairment of JA-induced defense responses.Citation19–Citation26,Citation45–Citation47 However, neither the ability to resist insect feeding nor VSP2 gene expression levels were impaired in primed aos or myc2 mutant plants compared to the primed wild-type plants (), suggesting priming-induced resistance to herbivory and elevated expression of VSP2 might be JA-independent. VSP2 has been reported to be regulated by MYC2-branch and directly regulated by MYC2 in the JA signaling pathway. However, gene expression in eukaryotes is often regulated by multiple factors which increases the plasticity of organisms in responses to changing environments. In JA-signaling pathway, MYC2 binds to G-box of target genes including VSP2.Citation25 In silico analysis reveals that the promoter of VSP2 is enriched with W-box, ABRE, and as-1 motifsCitation48 in addition to G-box, which are extensively involved in responses to both biotic and abiotic stresses. W-box is specific recognized by WRKY transcription factors and ABRE is responsive to ABA signaling. TGA members binds to as-1 motif to activate gene transcription. These cis-elements probably equip VSP2 with anchoring sites for other priming-induced transcription factors. Those transcription factors, which are JA-independent, might be involved in activation of VSP2 during the process of priming.
Our results indicated that salt-priming treatment at seedling stage enhanced adult plants’ defense responses against herbivory. The previously primed plants showed higher expression of VSP2 in response to scissor-clipping as a mimic of chewing herbivores (Figure S4 in Supplmentary Material). In response to wounding, the VSP2 expression level of primed plants was 79.7% higher than that of non-primed plants. The augmented VSP2 expression might improve plant performance in the battle with herbivores. Based on our results, NaCl-priming barely hindered Arabidopsis growth after germination. Instead, the priming treatment enhanced Arabidopsis growth after transplanted into stress-free environment, which was indicated by the increased biomass and rosette leaf size (Figure S5 in Supplmentary Material). Considering the fact that hyperactive defense often results in inhibited growthCitation49 and JA is a potent inhibitor of plant growth,Citation50,Citation51 the primed defense by NaCl seems to be a leverage to improve plant performance under attack without the penalty of JA-mediated growth inhibition, which might be an additional bonus for increasing the yield of crop vegetative organs. Presumably the salt-priming could be regarded as a mild biotic stress, it’s surprising that Arabidopsis seedlings memorized such a “lesson” and activated defense responses even the environmental factors have been reset after the transplantation. In this respect, we proposed that the seedling priming induced by NaCl should have a promising prospect in agronomy, especially where seedlings were transplanted after germination and incubation artificially.
Author’s contributions
Mu Xiao conducted the experiments of seedling priming, bioassay, RT-qPCR, secondary metabolite measurements and data processing. Rong Liu was responsible for rearing the insects and verifying knock-out of MYC2. Ying Ruan and Chunlin Lin designed the experiments and drafted the manuscript. All authors participated in the manuscript revision.
Supplemental Material
Download Zip (4 MB)Disclosure statement
No potential conflicts of interest were disclosed.
Supplementary material
Supplemental data for this article can be accessed on the publisher’s website.
Additional information
Funding
References
- Yang Z, Chen H, Tang W, Hua H, Lin Y. Development and characterisation of transgenic rice expressing two Bacillus thuringiensis genes. Pest Manag Sci. 2011;67(4):414–422. doi:10.1002/ps.2079.
- Buswell W, Schwarzenbacher RE, Luna E, Sellwood M, Chen B, Flors V, Pétriacq P, Ton J. Chemical priming of immunity without costs to plant growth. New Phytol. 2018;218(3):1205–1216. doi:10.1111/nph.15062.
- Savvides A, Ali S, Tester M, Fotopoulos V. Chemical priming of plants against multiple abiotic stresses: mission possible? Trends Plant Sci. 2016;21(4):329–340. doi:10.1016/j.tplants.2015.11.003.
- Conrath U, Beckers GJ, Flors V, García-Agustín P, Jakab G, Mauch F, Newman MA, Pieterse CM, Poinssot B, Pozo MJ, et al. Priming: getting ready for battle. Mol Plant Microbe Interact. 2006;19(10):1062–1071. doi:10.1094/MPMI-19-1062.
- Chen K, Arora R. Priming memory invokes seed stress-tolerance. Environ Exp Bot. 2013;94(6):33–45. doi:10.1016/j.envexpbot.2012.03.005.
- Pastor V, Luna E, Mauch-Mani B, Ton J, Flors V. Primed plants do not forget. Environ Exp Bot. 2013;94(5):46–56. doi:10.1016/j.envexpbot.2012.02.013.
- Bruce TJA, Matthes MC, Napier JA, Pickett JA. Stressful memories of plants: evidence and possible mechanisms. Plant Sci. 2007;173:603–608. doi:10.1016/j.plantsci.2007.09.002.
- Engelberth J, Alborn HT, Schmelz EA, Tumlinson JH. Airborne signals prime plants against insect herbivore attack. Proc Natl Acad Sci U S A. 2004;101:1781–1785. doi:10.1073/pnas.0308037100.
- Hodge S, Thompson GA, Powell G. Application of DL-beta-aminobutyric acid (BABA) as a root drench to legumes inhibits the growth and reproduction of the pea aphid Acyrthosiphon pisum (Hemiptera: aphididae). Bull Entomol Res. 2005;95:449–455. doi:10.1079/BER2005375.
- Huang T, Jander G, Vos MD. Non-protein amino acids in plant defense against insect herbivores: representative cases and opportunities for further functional analysis. Phytochemistry. 2011;72:1531–1537. doi:10.1016/j.phytochem.2011.03.019.
- Worrall D, Holroyd GH, Moore JP, Glowacz M, Croft P, Taylor JE, Paul ND, Roberts MR. Treating seeds with activators of plant defence generates long-lasting priming of resistance to pests and pathogens. New Phytol. 2012;193:770–778. doi:10.1111/j.1469-8137.2011.03987.x.
- Zhong Y, Wang B, Yan J, Cheng L, Yao L, Xiao L, Wu T. DL-β-aminobutyric acid-induced resistance in soybean against Aphis glycines Matsumura (Hemiptera: aphididae). PLoS One. 2014;15(9):e85142. doi:10.1371/journal.pone.0085142.
- Reynolds OL, Padula MP, Zeng R, Gurr GM. Silicon: potential to promote direct and indirect effects on plant defense against arthropod pests in agriculture. Front Plant Sci. 2016;7:744. doi:10.3389/fpls.2016.00744.
- Kaur S, Gupta AK, Kaur N. Effect of osmo-and hydro-priming of chickpea seeds on seedling growth and carbohydrate metabolism under water deficit stress. Plant Growth Regul. 2002;37:17–22. doi:10.1023/A:1020310008830.
- Yacoubi R, Job C, Belghazi M, Chaibi W, Job D. Proteomic analysis of the enhancement of seed vigour in osmoprimed alfalfa seeds germinated under salinity stress. Seed Sci Res. 2013;23:99–110. doi:10.1017/S0960258513000093.
- Debez A, Belghith I, Pich A, Taamalli W, Abdelly C, Braun HP. High salinity impacts germination of the halophyte Cakile maritima but primes seeds for rapid germination upon stress release. Physiol Plant. 2017;164(2):134–144. doi:10.1111/ppl.12679.
- Ellouzi H, Ben HK, Asensi-Fabado MA. Drought and cadmium may be as effective as salinity in conferring subsequent salt stress tolerance in Cakile maritima. Planta. 2013;237(5):1311–1323. doi:10.1007/s00425-013-1847-7.
- Singh P, Yekondi S, Chen PW, Tsai CH, Yu CW, Wu K, Zimmerli L. Environmental history modulates arabidopsis pattern-triggered immunity in a HISTONE ACETYLTRANSFERASE1-dependent manner. Plant Cell. 2014;26:2676–2688. doi:10.1105/tpc.114.123356.
- Ballaré CL. Jasmonate-induced defenses: a tale of intelligence, collaborators and rascals. Trends Plant Sci. 2011;16(5):249–257. doi:10.1016/j.tplants.2010.12.001.
- Acosta IF, Farmer EE. The arabidopsis book. Jasmonates. 2010;8:e0129. doi:10.1199/tab.0129.
- Wasternack C. Jasmonates: an update on biosynthesis, signal transduction and action in plant stress response, growth and development. Ann Bot. 2007;100(4):681–697. doi:10.1093/aob/mcm079.
- Park JH, Halitschke R, Kim HB, Baldwin IT, Feldmann KA, Feyereisen R. A knock-out mutation in allene oxide synthase results in male sterility and defective wound signal transduction in Arabidopsis due to a block in jasmonic acid biosynthesis. Plant J. 2002;31:1–12. doi:10.1046/j.1365-313X.2002.01328.x.
- Von Malek B, Van der Graaff E, Schneitz K, Keller B. The Arabidopsis male-sterile mutant dde2-2 is defective in the ALLENE OXIDE SYNTHASE gene encoding one of the key enzymes of the jasmonic acid biosynthesis pathway. Planta. 2002;216(1):187–192. doi:10.1007/s00425-002-0906-2.
- Berger S, Mitchellolds T, Stotz H. Local and differential control of vegetative storage protein expression in response to herbivore damage in Arabidopsis thaliana. Physiol Plant. 2010;114(1):85–91. doi:10.1046/j.0031-9317.2001.1140112.x.
- Dombrecht B, Xue G, Sprague SJ, Kirkegaard JA, Ross JJ, Reid JB, Fitt GP, Sewelam N, Schenk PM, Manners JM, et al. MYC2 differentially modulates diverse jasmonate-dependent functions in arabidopsis. Plant Cell. 2007;19(7):2225–2245. doi:10.1105/tpc.106.048017.
- Lorenzo O, Solano R. Molecular players regulating the jasmonate signalling network. Curr Opin Plant Biol. 2005;8(5):532–540. doi:10.1016/j.pbi.2005.07.003.
- Howe GA, Jander G. Plant immunity to insect herbivores. Annu Rev Plant Biol. 2008;59:41–66. doi:10.1146/annurev.arplant.59.032607.092825.
- Collins TJ. ImageJ for microscopy. Biotechniques. 2007;43(1):25–30. doi:10.2144/000112517.
- Hatsugai N, Katagiri F. Quantification of plant cell death by electrolyte leakage assay. Bio-Protocol. 2018;8(5):e2758. doi:10.21769/BioProtoc.2758.
- Livak KJ, Schmittgen TD. Analysis of relative gene expression data using real-time quantitative PCR and the 2(-Delta Delta C(T)) method. Methods. 2001;25(4):402–408. doi:10.1006/meth.2001.1262.
- Czechowski T, Stitt M, Altmann T, Udvardi MK, Scheible WR. Genome-wide identification and testing of superior reference genes for transcript normalization in Arabidopsis. Plant Physiol. 2005;139(1):5–17. doi:10.1104/pp.105.063743.
- Junglee S, Urban L, Sallanon H, Lopez-Lauri F. Optimized assay for hydrogen peroxide determination in plant tissue using potassium iodide. Am J Anal Chem. 2014;5:730e736. doi:10.4236/ajac.2014.511081.
- Brown PD, Tokuhisa JG, Reichelt M, Gershenzon J. Variation of glucosinolate accumulation among different organs and developmental stages of Arabidopsis thaliana. Phytochemistry. 2003;62:471–481.
- Das K, Roychoudhury A. Reactive oxygen species (ROS) and response of antioxidants as ROS-scavengers during environmental stress in plants. Front Environ Sci. 2014;2:53. doi:10.3389/fenvs.2014.00053.
- Sønderby IE, Geuflores F, Halkier BA. Biosynthesis of glucosinolates-gene discovery and beyond. Trends Plant Sci. 2010;15(5):283–290. doi:10.1016/j.tplants.2010.02.005.
- Burow M, Halkier BA. How does a plant orchestrate defense in time and space? Using glucosinolates in Arabidopsis as case study. Curr Opin Plant Biol. 2017;38:142–147. doi:10.1016/j.pbi.2017.04.009.
- Munns R, Tester M. Mechanisms of salinity tolerance. Annu Rev Plant Biol. 2008;59:651–684. doi:10.1146/annurev.arplant.59.032607.092911.
- Suzuki N, Miller G, Morales J, Shulaev V, Torres MA, Mittler R. Respiratory burst oxidases: theengines of ROS signaling. Curr Opin Plant Biol. 2011;14:691–699. doi:10.1016/j.pbi.2011.07.014.
- Marino D, Dunand C, Puppo A, Pauly N. A burst of plant NADPH oxidases. Trends Plant Sci. 2012;17:9–15. doi:10.1016/j.tplants.2011.10.001.
- Saed-Moucheshi A, Shekoofa A, Pessarakli M. Reactive oxygen species (ROS) generation and detoxifying in plants. J Plant Nutr. 2014;37(10):1573–1585. doi:10.1080/01904167.2013.868483.
- Czarnocka W, Karpiński S. Friend or foe? Reactive oxygen species production, scavenging and signaling in plant response to environmental stresses. Free Radic Biol Med. 2018;122:4–20. doi:10.1016/j.freeradbiomed.2018.01.011.
- Balazadeh S 1, Jaspert N, Arif M, Mueller-Roeber B, Maurino VG. Expression of ROS-responsive genes and transcription factors after metabolic formation of H(2)O(2) in chloroplasts. Front Plant Sci. 2012 Nov 1;3:234. eCollection 2012. doi:10.3389/fpls.2012.00234.
- Zhao Y 1, Wang J, Liu Y, Miao H, Cai C, Shao Z, Guo R, Sun B, Jia C, Zhang L, et al. Classic myrosinase-dependent degradation of indole glucosinolate attenuates fumonisin B1-induced programmed cell death in Arabidopsis. Plant J. 2015 Mar;81(6):920–933. doi:10.1111/tpj.12778.
- Glucosinolate degradation products, isothiocyanates, nitriles, and thiocyanates, induce stomatal closure accompanied by peroxidase-mediated reactive oxygen species production in Arabidopsis thaliana
- Benedetti CE, Costa CL, Turcinelli SR, Arruda P. Differential expression of a novel gene in response to coronatine, methyl jasmonate, and wounding in the Coi1 mutant of Arabidopsis. Plant Physiol. 1998;116(3):1037–1042. doi:10.1104/pp.116.3.1037.
- McConn M, Creelman RA, Bell E, Mullet JE, Browse J. Jasmonate is essential for insect defense in Arabidopsis. Proc Natl Acad Sci U S A. 1997;94:5473–5477.
- Kazan K, Manners JM. MYC2: the master in action. Mol Plant. 2013;6(3):686–703. doi:10.1093/mp/sss128.
- Lescot M, Déhais P, Thijs G, Marchal K, Moreau Y, Van de Peer Y, Rouzé P, Rombauts S. PlantCARE, a database of plant cis-acting regulatory elements and a portal to tools for in silico analysis of promoter sequences. Nucleic Acids Res. 2002 Jan 1;30(1):325–327.
- Huot B, Yao J, Montgomery BL, He SY. Growth-defense tradeoffs in plants: a balancing act to optimize fitness. Mol Plant. 2014;7:1267–1287. doi:10.1093/mp/ssu049.
- Yan Y, Stolz S, Chetelat A, Reymond P, Pagni M, Dubugnon L, Farmer EE. A downstream mediator in the growth repression limb of the jasmonate pathway. Plant Cell. 2007;19:2470–2483. doi:10.1105/tpc.107.050708.
- Noir S, Bomer M, Takahashi N, Ishida T, Tsui TL, Balbi V, Shanahan H, Sugimoto K, Devoto A. Jasmonate controls leaf growth by repressing cell proliferation and the onset of endoreduplication while maintaining a potential stand-by mode. Plant Physiol. 2013;161:1930–1951. doi:10.1104/pp.113.214908.