ABSTRACT
Phytoliths are silica bodies of various shapes including in the shape of sharp needles formed by many land plants. Defense from herbivory is one of the several known functions of phytoliths, especially the mechanical defense by abrasion of the mouthparts of arthropods and the teeth of mammalian herbivores. Another, although somewhat lesser-known, anti-herbivory defensive mechanism of phytoliths is wounding by sharp silica needles. We discuss and illuminate an even much less known defensive mechanism by phytoliths, i.e., the ability of needle-like phytoliths to insert microscopic pathogens (bacteria, fungi, viruses) into herbivores‘ tissues. We do it by comparison and by showing analogy with the better-known insertion of microbial pathogens into the body of herbivores by thorns, spines, and prickles. This largely overlooked and understudied defensive mechanism is a special case of a double extended phenotype; plants‘ defense, and the multiplication and dispersal of microorganisms, and is thus a case of mutualism.
Introduction
Many plants, especially the grasses, produce a large number of silica bodies, known as phytoliths.Citation1–Citation3 Phytoliths are formed by an ordered biological deposition of silicon that enters the plant from the soil solution via the roots.Citation4,Citation5 Phytoliths have several known functions, including structural, optical, serving as co-factors in the detoxification of heavy metals, and even as defense from herbivory.Citation4,Citation6–Citation8
It was suggested a long time ago, that phytoliths physically defend plants from herbivory, especially by wearing down the mouthparts of arthropods and mammals‘ teeth.Citation7,Citation9–Citation13 Lev-Yadun and HalpernCitation14 proposed and shortly discussed an additional and a different silica-body-based potential defensive (anti-herbivory) mechanism. Accordingly, many plant species that do not have thorns, spines, or prickles, use their sharp microscopic defensive silica needles (or calcium oxalate needles, known as raphides) as an alternative. Lev-Yadun and HalpernCitation14 suggested that sharp phytoliths not only mechanically wound various herbivores‘ tissues, but that the many small wounds inflicted by them, enable the penetration of various harmful microorganisms into the tissues of the attacking herbivores. Because of their small and even microscopic size, silica needles have an inherited advantage over typical spines, thorns, or prickles, which are very much larger than phytoliths. Small and sharp phytoliths can internally wound the mouth and digestive system not only of large vertebrates, but also of insects and other small herbivores such as snails, that usually manage to avoid thorns, spines, and prickles by passing between them. As with thorns, spines and prickles,Citation15–Citation17 the silica needles may actually inject the pathogenic microorganisms found on the plant surfaces into the herbivores‘ tissues. Moreover, these silica needles may wound the mouth and the digestive tracts of the herbivores, and thus may also cause infections by the herbivores‘ endogenous microbiota.Citation14 These, for example, can cause bacteremia in the herbivores‘ blood system.
In this short review, we illuminate an almost unknown indirect defensive (anti herbivory) mechanism by phytoliths, i.e., their ability to insert microscopic pathogens (bacteria, fungi, viruses) into herbivores‘ tissues.
Pathogenic bacteria and fungi on thorns, spines, and prickles
Thorns, spines, and prickles are commonly visually aposematicCitation18–Citation20 and thus have the potential to deter herbivores. Halpern et al.Citation15 found that bacterial pathogens commonly inhabit these sharp-pointed structures and that they may have a special potential role in defending plants from herbivores. The severity and frequency of aggressive and very hard to cure even with modern medicins of bacterial infections among date-palm orchard workers in Israel, following spine wounding, have necessitated the costly practice of the removal of all the millions of spines from many of the orchards by mechanical saws. This is a very good indication that an important aspect of defensive (anti-herbivory) plant ecology was hidden there.
Spines from leaflets of the lower leaves of date palm (Phoenix dactylifera) trees, thorns from common hawthorn (Crataegus aronia) trees, and from two thorny shrub species, i.e., thorny burnet (Sarcopoterium spinosum) and manna tree (Alhagi graecorum), all growing in northern Israel, were sampled for aerobic and anaerobic bacteria. Every typical mature individual of these trees and shrubs carry hundreds or even many thousands of spines or thorns.Citation21 Halpern et al.,Citation15–Citation17 and Lev-Yadun and HalpernCitation14 showed that thorns, spines, and prickles indeed regularly harbor an array of pathogenic bacteria and fungi, and discussed their potential significance in the evolution and functionality of microorganism-based plant defense.
Indeed, spine and thorn studies resulted in the identification of several very pathogenic aerobic and anaerobic bacterial species, including Clostridium perfringens, Bacillus anthracis, and Pantoea agglomerans.Citation15–Citation17 Clostridium perfringens is known to be a flesh-eater in that it can produce a necrotizing infection of the skeletal muscle called gas gangrene.Citation22 Bacillus anthracis is the etiological agent of anthrax, a notoriously acute fatal disease in both domesticated and wild animals, particularly herbivorous ones, and also in humans.Citation23 The cutaneous form of the disease is usually acquired through injured skin or mucous membranes, a typical thorn, spine or prickle injury. Clostridium tetani is the etiological agent of tetanus, a serious disease in humans and animals, which can be fatal when left untreated. Thorn, spine and prickle injuries have been known to cause tetanus in many countries, for instance in the USA, in Ethiopia, and in Turkey.Citation24–Citation26
In their review of the medical literature, Halpern et al.Citation16 found that septic inflammation caused by plant thorn, spine, or prickle injury, can result not only from bacteria, but also from pathogenic fungi. Dermatophytes that cause subcutaneous mycoses are unable to penetrate the skin and must be introduced into the subcutaneous tissue by a puncture or a cut wound.Citation27 Thus, the physical defense against herbivores provided to the plants by thorns, spines, and prickles, might be only the tip of the defensive iceberg, indicating that on the sharp defensive appendages there is a much more complicated story, hidden from the naked eye. These sharp plant structures may inject various types of microorganisms into herbivores by wounding, enabling the microorganisms to pass the animal’s first line of defense (the skin) and in so doing may cause severe infections that are much more dangerous and also have the potential to inflict more pain than the mechanical wounding itself.Citation14–Citation17
An obvious question concerning the potential defensive (anti-herbivory) role of pathogenic microorganisms on plant surfaces is about those found on surfaces other than on thorns, spines or prickles. The positive answer to this question is theoretically simple in many cases. Thousands of spineless plant species have internal and/or external sharp microscopic alternatives to thorns, spines and prickles, i.e., pointed and sharp phytoliths, which can, like thorns, spines or prickles, insert bacterial, viral, and fungal pathogens, and probably also dangerous viruses into the tissues of the herbivores that attack the plants. Microorganisms are ubiquitous, so there is no reason to assume that only specific plants or specific plant organs are inhabited by high microorganism diversity.
Despite this ubiquitous occurrence, however, certain plants or plant organs may have specific chemical components or microscopic structures on their surfaces that either reduce or increase the possibility of survival for various microorganism taxa. For example, microorganisms can grow on plant surfaces in biofilms, which are assemblages of bacterial cells attached to a surface and enclosed in adhesive polysaccharides excreted by the bacteria. Within the biofilm matrix, several different microenvironments may exist, including pockets of anoxic conditions that facilitate the existence of anaerobic bacteria. Considering the findings of Halpern et al.Citation15–Citation17 in regard to spines and thorns, it is clear that not only aerobic, but also pathogenic anaerobic bacteria survive on these defensive structures. Although limited in its scope, the study by Halpern et al.Citation17 gave good indications that in the palm Washingtonia filifera there are significant differences in the composition of the bacterial flora of the colorful spines, versus green, flat, and soft photosynthetic tissues of the same leaf.
Silica needles wound and support the insertion of pathogenic microorganisms into animal tissues
There is clear evidence of the considerable ability of silica needles to wound animal and human tissues.Citation28–Citation31 Since silica needles, especially in various members of the Poaceae, are formed in huge numbers,Citation3 possibly even millions per plant, they cause huge numbers of small wounds in the mouth and digestive systems of herbivores, and also in the skins of humans and animals that consume them or just come into close contact with the plants. Eventually, such wounds can commonly cause cancer. Carcinogenesis following exposure to silica needles is known concerning several members of the genera Phalaris,Citation29,Citation32,Citation33 Setaria,Citation28,Citation34 Saccharum (sugar cane),Citation35 and Oryza (rice).Citation36
Plant-related extended phenotype functions
The concept of the extended phenotype was introduced by DawkinsCitation37 concerning animals. According to this concept, an animal‘s behavior tends to maximize the survival of its genes whether or not those genes happen to be in the body of the particular animal performing it, or if the phenotype is expressed outside its body.Citation37,Citation38 Aposematism, i.e., warning signaling, defends plants from herbivores.Citation18-Citation20 RothschildCitation39,Citation40 described how various toxic aposematic insects aggregate on poisonous plants, thus adding to the plant‘s aposematic odor and possibly to its aposematic coloration. In the cases discussed by Rothschild,Citation39,Citation40 the aposematic apparatus was not based on and not made of plant parts, but rather made of poisonous insects (e.g., extended phenotype sensu DawkinsCitation37), but not much research has been done on this.
Concerning the defense of plants against herbivory via an extended phenotype, colorful, and chemically defended plant galls (abnormal plant growths induced by various organisms) were recently proposed to be the extended aposematic phenotypes of their inducing aphids.Citation41 The aposematic signaling about the gall‘s defense has two components: (1) colors and shape, and (2) chemical defense. Many galls are colorful (red, yellow, etc.), and their colors are produced by the host‘s tissues, and the galls can be clearly distinguished from other parts of their plant host. Some of the defensive secondary metabolites, which are volatile, are also produced by the host‘s tissues, and may thus serve as olfactory signals about the chemical defense.Citation42,Citation43 These visual and olfactory components serve in visual aposematism of the galls. These plant-related extended phenotype functions defend these induced abnormal plant parts, i.e., the galls, from potential enemies of the gall-inducing organisms.Citation41
When plants are defended from herbivory by microorganisms, it is a good case of an extended phenotype and even a double one as we will discuss below.
Concerning microorganisms in plants that have sharp phytoliths, the plants can enjoy defensive profits following the infections inflicted on herbivores by the various pathogenic microorganisms that by this, serve as their extended phenotype. At the same time, the microorganisms that need susceptible hosts for their multiplication and dispersal, i.e., for their own fitness, by practically using the plants as lures and traps for the herbivores victims, which they can attack only after the herbivores consume the plants. Altogether, this is actually a mutualistic system where plants enjoy defense by microorganisms, and the microorganisms enjoy a supply of susceptible herbivorous animal targets ().
To add an additional layer to that already complicated system and of relationships, the pathogens already found in the mouths and digestive tracts of the herbivores can also exploit the wounds inflicted by the phytoliths, and thus, cause various infections to the herbivores.
Olfactory plant aposematism versus olfactory luring of herbivores
The question of whether simultaneous olfactory aposematism is involved in bacteria-infected plants has never been addressed. Moreover, the even more complicated possibility that the pathogenic bacterial biofilms emit attractive molecules that cause herbivores to approach the plants and thus be wounded and infected to the benefit of the bacteria, which by this will contaminate new victims, and in the long run also benefit the plants because of reduction of herbivore populations, has also never been addressed. There are very good experimental indications that microorganisms my lure animals to their host plants. Eberl et al.Citation44 found that the rust fungus Melampsora larici-populina reduced herbivore-induced volatile emission and terpene synthase transcripts in Populus nigra, influencing herbivore preferences. Mwando et al.Citation45 found that maize plants attacked by the maize chlorotic mottle virus (MCMV) emitted volatiles that attracted the trips species Thrips tabaci that is a vector of the virus. Rering et al.Citation46 found that nectar-inhabiting microorganisms influence nectar volatile composition and attraction of pollinating bees in various wild Californian plant species. These cases indicate that pathogenic microorganisms that occupy plant surfaces have a potential of attracting herbivores to thorny, spiny, and prickly plants.
Future directions
Many more studies are needed in order to evaluate the defensive microorganism flora on plant surfaces, including studies of plants that have sharp silica needles. There is a great need to better understand the physical and chemical components of the potential defense of plants from herbivory by injecting pathogenic microorganisms via sharp plant organs and structures. The following subjects should be explored: (1) The nature and composition of the microflora found on plant surfaces, as well as on the skin and in the mouth and digestive tracts of herbivores. (2) The nature of biofilms, their chemical composition, and the microorganism taxa involved in forming them, as well as their population dynamics. (3) The changes of microorganism biodiversity with plant age, nutrition, light level, and spectra, patterns of air and plant surface humidity, plant surface chemistry including pH, and micro-topography, seasonality, influences of rain, snow, fires, and in relation to the diversity of animals in the environments. (4) The possibility that the various microorganisms emit attracting molecules that can attract herbivores or that the plants emit repelling molecules because they sense the microorganisms, i.e., olfactory aposematism, should be carefully examined (). (5) The possibility of visual aposematism associated with defensive silica bodies, especially sharp ones. Since many insects see UV light, the possibility that plants defended by silica needles that can harm caterpillars advertise it visually to insect herbivores in the UV should also be examined.
Altogether, actual cases and not only well-planned lab experiments of defense via wounding and pathogenesis of the herbivores should be studied. This new, complicated, little studied and little discussed area of phytolith-related plant defense from herbivores, is a terra icognita that probably holds many potential surprizing discoveries.
Conclusion
Here we illuminate the overlooked potential plant defense from herbivory, by sharp phytoliths via microscopic pathogens (bacteria, fungi, viruses) into herbivores‘ tissues. summarizes a comparison between this plant defensive mechanism and the better-known insertion of microbial pathogens into the herbivores by thorns, spines, and prickles. We hope that this review will stimulate studies regarding this defensive strategy.
Figure 1. Mutualistic relationship between plants and bacteria: Pathogenic bacterial biofilms may release attractive or repellent molecules to attract or repel herbivores (yellow green and red squares, left side of the diagram) while thorns, spines, prickles or microscopic needles may insert these pathogenic bacteria into herbivores (right side of the diagram)
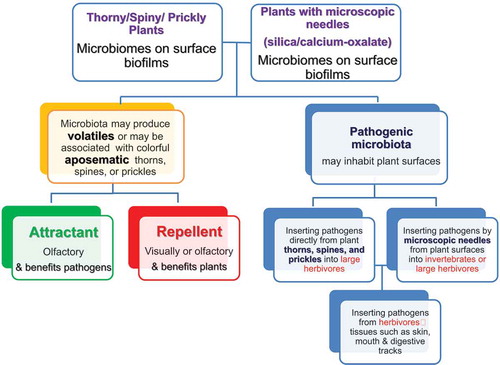
Disclosure of Potential Conflicts of Interest
No potential conflicts of interest were disclosed.
Acknowledgment
This research was supported by the Joint Program between the Israel Science Foundation (ISF) and the University Grants Commission of India (UGC); (UGC-ISF grant: 2728/17).
References
- Rapp G Jr, Mulholland SC, Eds. Phytolith systematics. emerging issues. New York (NY): Plenum Press; 1992.
- Meunier JD, Colin F, Eds. Phytoliths: application in earth sciences and human history. Lisse (Belgium): A.A. Balkema Publishers; 2001.
- Prychild CJ, Rudall PJ, Gregory M. Systematics and biology of silica bodies in Monocotyledons. Bot Rev. 2003;69:377–440. doi:10.1663/0006-8101(2004)069[0377:SABOSB]2.0.CO;2.
- Richmond KE, Sussman M. Got silicon? The non-essential beneficial plant nutrient. Curr Opin Plant Biol. 2003;6:268–272.
- Kumar S, Soukup M, Elbaum R. Silicification in grasses: variation between different cell types. Front Plant Sci. 2017;8:438. doi:10.3389/fpls.2017.00438.
- Wang Y, Stass A, Horst WJ. Apoplastic binding of aluminum is involved in silicon-induced amelioration of aluminum toxicity in maize. Plant Physiol. 2004;136:3762–3770. doi:10.1104/pp.104.045005.
- Katz O. Silica phytoliths in Angiosperms: phylogeny and early evolutionary history. New Phytol. 2015;208:642–646. doi:10.1111/nph.13559.
- Meharg C, Meharg AA. Silicon, the silver bullet for mitigating biotic and abiotic stress, and improving grain quality, in rice? Environ Exp Bot. 2015;120:8–17. doi:10.1016/j.envexpbot.2015.07.001.
- Baker G, Jones LHP, Wardrop ID. Cause of wear in sheeps‘ teeth. Nature. 1959;184:1583–1584.
- Walker A, Hoeck HN, Perez L. Microwear of mammalian teeth as an indicator of diet. Science. 1978;201:908–910.
- McNaughton SJ, Tarrants JL. Grass leaf silicification: natural selection for an inducible defense against herbivores. Proc Natl Acad Sci USA. 1983;80:790–791.
- McNaughton SJ, Tarrants JL, McNaughton MM, Davis RH. Silica as a defense against herbivory and a growth promotor in African grasses. Ecology. 1985;66:528–535. doi:10.2307/1940401.
- Massey FP, Hartley SE. Experimental demonstration of the antiherbivore effects of silica in grasses: impacts on foliage digestibility and vole growth rates. Proc R Soc Lond B. 2006;273:2299–2304. doi:10.1098/rspb.2006.3586.
- Lev-Yadun S, Halpern M. External and internal spines in plants insert pathogenic microorganisms into herbivore‘s tissues for defense. In: Van Dijk T, editor. Microbial ecology research trends. New York (NY): Nova Scientific Publishers; 2008. p. 155–168.
- Halpern M, Raats D, Lev-Yadun S. Plant biological warfare: thorns inject pathogenic bacteria into herbivores. Environ Microbiol. 2007;9:584–592. doi:10.1111/j.1462-2920.2006.01174.x.
- Halpern M, Raats D, Lev-Yadun S. The potential anti-herbivory role of microorganisms on plant thorns. Plant Signaling & Behav. 2007;2:503–504. doi:10.4161/psb.2.6.4608.
- Halpern M, Waissler A, Dror A, Lev-Yadun S. Biological warfare of the spiny plant: introducing pathogenic microorganisms into herbivore‘s tissues. Adv Appl Microbiol. 2011;74:97–116. doi:10.1016/B978-0-12-387022-3.00008-2.
- Lev-Yadun S. Aposematic (warning) coloration associated with thorns in higher plants. J Theor Biol. 2001;210:385–388. doi:10.1006/jtbi.2001.2315.
- Lev-Yadun S. Defensive (anti-herbivory) coloration in land plants. Anti-herbivory plant coloration and morphology. Zug Switzerland: Springer; 2016.
- Lev-Yadun S. Defensive (anti-herbivory) Batesian mimicry in plants. Isr J Plant Sci. 2019;66:34–51. doi:10.1163/22238980-00001044.
- Ronel M, Malkiel H, Lev-Yadun S. Quantitative characterization of the thorn system of the common shrubs Sarcopoterium spinosum and Calicotome villosa. Isr J Plant Sci. 2007;55:63–72. doi:10.1560/IJPS.55.1.63.
- Shimizu T, Ohtani K, Hirakawa H, Ohshima K, Yamashita A, Shiba T, Ogasawara N, Hattori M, Kuhara S, Hayashi H. Complete genome sequence of Clostridium perfringens, an anaerobic flesh-eater. Proc Natl Acad Sci USA. 2002;99:996–1001. doi:10.1073/pnas.022493799.
- Jensen GB, Hansen BM, Eilenberg J, Mahillon J. The hidden lifestyles of Bacillus cereus and relatives. Environ Microbiol. 2003;5:631–640.
- Hodes RM, Teferedegne B. Tetanus in Ethiopia: analysis of 55 cases from Addis Ababa. E Afr Med J. 1990;67:887–893.
- Ergonul O, Erbay A, Eren S, Dokuzoguz B. Analysis of the case fatality rate of tetanus among adults in a tertiary hospital in Turkey. Eur J Clin Microbiol Infect Dis. 2003;22:188–190.
- Pascual FB, McGinley EL, Zanardi LR, Cortese MM, Murphy TV 2003. Tetanus surveillance, United States, 1998-2000. 52, SS03 1-8 Available from: http://www.cdc.gov/mmwr/preview/mmwrhtml/ss5203a1.htm.
- Willey JM, Sherwood LM, Woolverton CJ. Prescot, Harley, and Klein‘s Microbiology. 7th. New York (NY): McGraw Hill; 2008.
- O‘Neill C, Clarke G, Hodges G, Jordan P, Newman R, Pan -Q-Q, Liu F-S, Ge M, Chang Y-M, Toulson E. Silica fragments from millet bran in mucosa surrounding oesophageal tumours in patients in northern China. Lancet. 1982;319:1202–1206. doi:10.1016/S0140-6736(82)92335-2.
- Bhatt T, Coombs M, O‘Neill C. Biogenic silica fiber promotes carcinogenesis in mouse skin. Int J Cancer. 1984;34:519–528.
- Newman RH. Fine biogenic silica fibres in sugar cane: a possible hazard. Ann Occup Hyg. 1986;30:365–370.
- Hodson MJ, Smith RJ, van Blaaderen A, Crafton T, O‘Neill CH. Detecting plant silica fibres in animal tissue by confocal fluorescence microscopy. Ann Occup Hyg. 1994;38:149–160.
- O‘Neill CH, Hodges GM, Riddle PN, Jordan PW, Newman RH, Flood RJ, Toulson EC. A fine fibrous silica contaminant of flour in the high oesophageal cancer area of north-east Iran. Int J Cancer. 1980;26:617–628.
- Sangster AG, Hodson MJ, Wynn Parry D. Silicon deposition and anatomical studies in the inflorescence bracts of four Phalaris species with their possible relevance to carcinogenesis. New Phytol. 1983;93:105–122. doi:10.1111/j.1469-8137.1983.tb02696.x.
- Wynn Parry D, Hodson MJ. Silica distribution in the caryopsis and inflorescence bracts of foxtail millet [Setaria italica (L.) Beauv.] and its possible significance in carcinogenesis. Ann Bot. 1982;49:531–540. doi:10.1093/oxfordjournals.aob.a086278.
- Amre DK, Infante-Rivard C, Dufresne A, Durgawale PM, Ernst P. Case-control study of lung cancer among sugar cane farmers in India. Occup Environ Med. 1999;56:548–552.
- McCurdy SA, Ferguson TJ, Goldsmith DF, Parker JE, Schenker MB. Respiratory health of Californian rice farmers. Amer J Respir Crit Care Med. 1996;153:1553–1559. doi:10.1164/ajrccm.153.5.8630601.
- Dawkins R. The Extended Phenotype. Oxford (UK): Oxford University Press; 1982.
- Schaedelin FC, Taborsky M. Extended phenotypes as signals. Biol Rev. 2009;84:293–313. doi:10.1111/j.1469-185X.2008.00075.x.
- Rothschild M. Some observations on the relationship between plants, toxic insects and birds. In: Harborne JB, editor. Phytochemical Ecology. London (UK): Academic Press; 1972. p. 1–12.
- Rothschild M. The red smell of danger. New Sci. 1986;111:34–36.
- Inbar M, Izhaki I, Lupo I, Silanikove N, Glasser T, Gerchman Y, Perevolotsky A, Lev-Yadun S. Why do many galls have conspicuous colors? A new hypothesis. Arthropod-Plant Interact. 2010;4:1–6. doi:10.1007/s11829-009-9082-7.
- Rostás M, Maag D, Ikegami M, Inbar M. Gall volatiles defend aphids against a browsing mammal. BMC Evol Biol. 2013;13:193. doi:10.1186/1471-2148-13-193.
- Rand K, Bar E, Ben-Ari M, Lewinsohn E, Inbar M. The mono- and sesquiterpene content of aphid-induced galls on Pistacia palaestina is not a simple reflection of their composition in intact leaves. J Chem Ecol. 2014;40:632–642. doi:10.1007/s10886-014-0462-9.
- Eberl F, Hammerbacher A, Gershenzon J, Unsicker SB. Leaf rust infection reduces herbivore-induced volatile emission in black poplar and attracts a generalist herbivore. New Phytol. 2018;220:760–772. doi:10.1111/nph.14565.
- Mwando NL, Tamiru A, Nyasani JO, Obonyo MAO, Caulfield JC, Bruce TJA, Subramanian S. Maize chloretic mottle virus induces changes in host plant volatiles that attract vector thrips species. J Chem Ecol. 2018;44:681–689. doi:10.1007/s10886-018-0973-x.
- Rering CC, Beck JJ, Hall GW, McCartney MM, Vannette RL. Nectar-inhabiting microorganisms influence nectar volatile composition and attractiveness to a generalist pollinator. New Phytol. 2018;220:750–759. doi:10.1111/nph.14809.