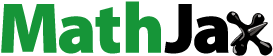
ABSTRACT
Stomatal responses to changes in leaf water status are critical for minimizing excessive water loss during soil drought. A major debate has surrounded the evolution of stomatal responses to water status and this debate has particularly focused on the evolution of the regulatory role of the drought hormone abscisic acid (ABA). Studies relying on the application of high levels of exogenous ABA have occasionally concluded that all stomata respond to ABA and that stomatal regulation in response to this hormone has not evolved over the past 450 million years. In contrast, studies which have investigated stomatal function in intact plants, as well as the role of endogenous ABA in regulating stomatal aperture, have found major evolutionary transitions in the functional regulation of stomata across land plant lineages. We show that endogenous ABA plays no role in closing the stomata of the fern Nephrolepis exaltata during natural soil drought, in contrast to a recent finding using isolated epidermis and exceptionally high levels of exogenous ABA. We conclude that stomatal behavior in intact plants has evolved over time, and may have shaped the evolutionary and ecological success of successive land plant lineages.
Leaves continuously regulate the rate of water loss to the atmosphere by modifying stomatal aperture in response to both environmental and endogenous signals. During periods of water limitation in particular, stomatal closure is a critical tool for vascular plant survival, minimizing transpiration and preventing plant dehydration.Citation1,Citation2 In angiosperms, stomatal closure during water stress is mediated by the phytohormone ABA;Citation3–Citation5 however, contradictory results fuel an ongoing debate about the evolutionary origin of this hormonal regulation of stomata.Citation6–Citation8
In contrast to vascular land plants, in which stomata mainly occur on leaves, in two lineages of bryophytes (the earliest diverging clades of land plants), the mosses and hornworts, stomata are restricted to the sporophyte.Citation9 An early report of stomata on the sporophyte in the moss Physcomitrella closing in response to exogenous ABA suggested an early evolutionary acquisition of stomatal control by this hormone.Citation8 A number of more recent studies on stomata of hornworts and mosses, however, demonstrate no stomatal responses to exogenous ABA.Citation10–Citation12 Furthermore, the stomata of hornworts and mosses are anatomically and physiologically constrained such that they lack the capacity to alter stomatal aperture once the pore opens.Citation10,Citation11,Citation13,Citation14 There is elegant evidence showing that the stomata of bryophytes open once at a single developmental stage and that this opening plays a primary role in sporophyte desiccation and spore discharge. This is the opposite function of vascular plant stomata, which close to prevent desiccation. The opening of some bryophyte stomata may even be by an opposite mechanism to that of vascular plant stomata, opening when the guard cells loose turgor.Citation15
The major evolutionary transition in stomatal function between bryophytes and tracheophytes makes the ferns and lycophytes which are the earliest diverging, extant lineages of vascular land plants, the most important groups of plants for reconstructing physiological evolution in the tracheophytes.Citation16 Understanding functional stomatal behavior in species from these early diverging lineages is essential for identifying the major evolutionary transition(s) that must have occurred in guard cells to cause the macro-shift in stomatal function between bryophytes and tracheophytes. Given the corresponding major shifts in ecological niche over time (from perennial swamp to seasonally dry uplands) and the association of these shifts with the emergence of new lineages of vascular plant,Citation17 physiological explanations for these transitions remain surprisingly limited.Citation18 It is tempting to hypothesize that the evolution of stomatal function, which is so critical for angiosperm survival in dry environments,Citation2 may have played a role over evolutionary time in the invasion of increasingly drier habitats by terrestrial plants.Citation19 It seems that the evolution of the unique dumbbell stomata of grasses is associated with increased stomatal speed and responsiveness,Citation20 suggesting that there is indeed a possibility that stomatal function has evolved across the tracheophytes. Furthermore, there is evidence of major changes across tracheophyte lineages in stomatal regulation in response to light and as well as CO2 concentration when altered in the dark,Citation21 these changes have been associated with adaptations to light environments.
To address whether differences in the regulation of stomatal responses to leaf water status occur across the lineages of vascular plants, in an early study Brodribb and McAdamCitation22 found that the stomata of a diversity of fern and lycophyte species were unresponsive (as measured by leaf gas exchange) to the feeding of exogenous ABA into the transpiration stream. Subsequently, in other species of fern and lycophyte, a lack of stomatal responses to ABA application has been found.Citation23–Citation25 The most convincing data indicating that stomatal responses to changes in leaf water status in fern and lycophyte species are not regulated by ABA is the observation that the stomata of drought-stressed fern and lycophyte species rapidly reopen to pre-drought levels when instantaneously rehydrated, despite high endogenous levels of foliar ABA accumulated during drought.Citation26–Citation28 This rapid reopening of stomata when endogenous ABA levels are high is never observed in seed plants, in which the accumulated endogenous ABA keeps stomata closed.Citation26,Citation29,Citation30 Instead of the metabolic regulation of stomatal responses to changes in leaf water status via ABA as typically observed in angiosperms,Citation3,Citation31,Citation32 the stomatal responses to changes in leaf water status in a diversity of fern and lycophyte species can be predicted accurately by a simple model that assumes guard cell turgor changes passively with leaf turgor.Citation22,Citation33,Citation34 The classical ABA signaling pathway responsible for closing stomata in angiosperms has also been found to regulate gametophyte sex determination and spore dormancy in the fern Ceratopteris richardii and not stomata, suggesting alternative ancestral functions for this plant hormone signaling pathway.Citation35
The conclusions from these data have, however, received continual criticism from studies in which stomatal aperture or leaf gas exchange are monitored in some fern and lycophyte species following the application of high levels of exogenous ABA.Citation6,Citation23,Citation24,Citation36 These contrasting results are championed as evidence that stomata from all species of land plant are functionally responsive to ABA, and consequently that stomatal evolution has played no role the ecological differentiation or evolution of vascular plant lineages.Citation6 Our recent work sought to investigate these conclusions by comprehensively examining the stomatal responses to changes in leaf water status in the fern species Athyrium filix-femina, which has been shown to have stomata that are closed by less than 20% following the spraying of leaves with exogenous ABA as high as 10 μM.Citation24 This applied concentration is an ideal standard treatment to elicit a saturating stomatal response to ABA in Arabidopsis and is widely used by cell and molecular biologists for this purpose. By virtue of this, it is indeed a high concentration, especially when considering the sensitivity of angiosperm stomata to ABA (), the levels of ABA synthesized by plants during drought stress,Citation48–Citation51 and the levels of ABA measured in closed stomata during drought stress in angiosperms,Citation52 which may even be synthesized in the guard cells.Citation53 We utilized a unique variety of this fern species as well as the wild-type to examine whether stomatal responses could be predicted by the simple assumption that guard cell turgor changes in unison with leaf turgor when leaf water status changes. We found that stomatal responses to changes in leaf water status in A. filix-femina were highly predictable, not requiring any metabolic input to explain responses to changes in vapor pressure difference. Furthermore, we found that the high levels of exogenous ABA required to close stomata by the reported small degree in this speciesCitation24 do not accumulate in planta when naturally water stressed.Citation28 During water stress A. filix-femina leaves start synthesizing ABA after stomata are closed, and this endogenous ABA does not restrict stomatal reopening when plants or leaves are rehydrated.Citation28
Table 1. The sensitivity of stomatal aperture in isolated epidermis of angiosperms exposed to exogenous ABA levels equal or lower than 10 μM.
A recent study has reported that the stomata of N. exaltata are not closed by exogenous ABA application, even when levels as high as 100 µM are applied to leaves.Citation25 These results are in direct conflict with those presented recently by Cai et al.Citation6 in which stomatal aperture in isolated epidermis of the same species was reported to close by approximately 25% in response to exposures ranging from 50 to 200 μM exogenous ABA. How can these two contrary results in the same species be reconciled? Despite differences in methodology, neither of these two recent and conflicting studies conducted experiments to examine the effect of endogenous ABA synthesized by the plant on stomatal function in this species. To address this particular limitation, we specifically examined the role of endogenous ABA synthesized by the plant in controlling stomatal responses to changes in leaf water status in N. exaltata. Like other fern species, endogenous ABA levels in N. exaltata were found to be very low under well-watered conditions ().Citation26 During natural soil water deficit, stomata closed and the level of endogenous ABA increased. To test whether this enhanced endogenous level of ABA played any role in closing stomata during drought stress, as it does in seed plants, we employed a simple experiment based on strong inference.Citation54 Two alternative hypotheses for the mechanism driving stomatal closure during drought are high levels of ABA (or other metabolic signals) that are synthesized by the plant during drought, or low turgor, which biophysically closes stomata. An experiment to systematically test which of these alternative possibilities are closing the stomata during drought is the monitoring of leaf gas exchange when a drought-stressed stem is excised under water, relaxing the tension on the water column. Instantaneous rehydration from a water stressed state rapidly increases cell turgor and therefore immediately eliminates the biophysical loss of cell turgor as a driving mechanism of stomatal closure. When instantaneously rehydrated, the stomata of N. exaltata rapidly reopened to predrought levels (), despite the maintenance of high levels of naturally synthesized, endogenous ABA accumulated in the leaves (). This result completely excludes the hypothesis that endogenous ABA accumulated naturally by the plant during drought (or indeed any other metabolic signal) plays a role in closing stomata during water stress in this species. A similar response has been observed in a number of other fern and lycophyte species.Citation26–Citation28 Our results rule out any role of endogenous ABA functionally regulating stomatal closure during soil drought in N. exaltata. Whether endogenous ABA changes the rate of stomatal responses to fluctuating light intensity as recently hypothesized by Grantz et al.Citation25 remains untested. It should be noted though that this novel and alternative role for ABA in regulating stomatal responses is very different from the effect of ABA driving stomatal closure.
Table 2. Leaf water potential and foliar ABA level for the control plants and two drought-stressed and rehydrated individuals of Nephrolepis exaltata from .
Figure 1. Highest endogenous ABA levels synthesized naturally by the fern Nephrolepis exaltata do not play a role in closing stomata during water stress.
The response of stomatal conductance to leaf rehydration through the stipe in two drought stressed individuals of N. exaltata (black and white circles). Dotted lines represent the stomatal conductance (mean ± SD) of three well-watered individuals of N. exaltata. For leaf water potential and foliar ABA levels (see ).
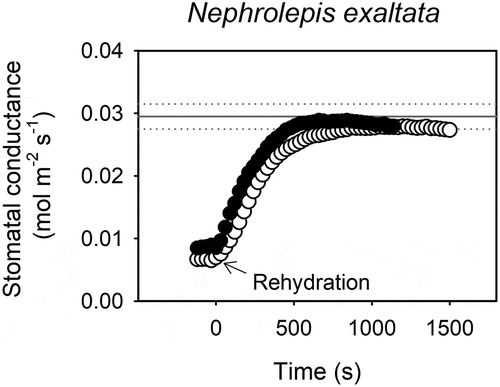
Our results indicating no role of endogenous ABA driving stomatal closure during water stress call into question the proposition by Cai et al.Citation6 of a meaningful physiological function of ABA in regulating stomatal aperture during drought in N. exaltata. Like other ferns, the ABA naturally synthesized by N. exaltata is neither responsible for stomatal closure under drought conditions, nor prevents stomatal reopening following rehydration.Citation26–Citation28 Both of these effects of ABA on stomata have been well-characterized physiologically and at a molecular level in angiosperms.Citation3,Citation26,Citation29–Citation32,Citation55 We believe that the two contrasting theories on stomatal evolution proposed over the past decade stem from a very different approach to investigating this question, particularly the use of experimental designs by one camp that cannot make a strong inference about stomatal function in intact plants. A common method employed by studies which conclude that stomatal function is unchanged across land plant lineages is the use of solutions of unnatural and extremely high levels of exogenous ABA and isolated epidermis floated in these solutions to determine the effect of this hormone on stomata (). This is despite a long and well-documented history of artifacts when working with epidermal peels, resulting from the physical stress of removing epidermal strips, mesophyll contamination, epidermal cell death,Citation58 and lack of replication with studies observing stomatal function in intact leaves or plants.Citation59,Citation60 In contrast, most studies which have employed gas exchange or direct stomatal observation in live and intact leaves, as well as experiments testing the effect of endogenous ABA on stomatal aperture during drought, have found little or no functional effect of ABA on stomatal responses to changes in leaf water status in fern and lycophyte species.
Figure 2. Comparison between the endogenous levels of ABA found in plants of Nephrolepis exaltata and the exogenous levels of ABA applied in two different studies using the same species.
Endogenous levels of ABA (mean ± SD; log scale) found in plants of N. exaltata under well-watered (n = 3) and water stress conditions (n = 2) (data from ) and exogenous levels of ABA applied to individuals from the same species in the studies from Cai et al.Citation6 (black solid and black dotted lines represent maximum concentration used in the dose–response curve and concentration chosen in further experiments, respectively) and Grantz et al.Citation25(gray solid line represents maximum concentration used in experiments). The red horizontal line represents 0.1 μM, corresponding to the cytosolic concentration of ABA required to fully activate ABA receptors in angiosperms.Citation56 To estimate the endogenous levels of ABA in terms of volume (molarity) rather than weight (gram), we utilized the same foliar ABA concentrations from , but in terms of FW, and the relationship between RWC and water potential for this species (Equation 1). It should be noted that in a water-stressed plants 15% of the ABA in the leaf remains bound in the chloroplasts, the rest is assumed to be cytosolic,Citation57 meaning that we our calculated molar concentrations of ABA from foliar measurements are an overestimate of cytosolic levels.
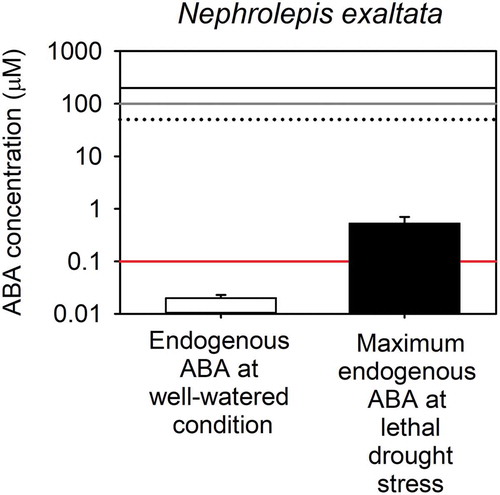
The behavior of fern and lycophyte stomata in an intact plant is very different from the stomatal behavior of seed plants, particularly in terms of stomatal responses to changes in leaf water status.Citation61 It is possible that this transition in stomatal behavior influenced major ecological transitions that occurred as the seed plant lineages first emerged during the late Carboniferous and early Permian, invading seasonally dry environments.Citation62 Discovering the molecular mechanisms underpinning this major evolutionary transition in how stomata perceive and respond to water limitation is consequently a major future research challenge. Future work examining the presence and function of ABA receptors, signaling proteins and anion channels in fern guard cells, as well as the capacity of ferns to synthesize ABA during water stress will reveal critical insights into the evolution of the stomatal response to ABA.
Material and methods
Plant material
Individuals of N. exaltata were grown in a 2:1 mix of Indiana Miami topsoil and ground pine bark under greenhouse conditions set at a night/day temperatures of 16/23ºC, and natural photoperiod. Plants were grown under a 75% shade cloth resulting in light intensity of approximately 300 μmol m−2 s–1. They received daily watering and a weekly application of liquid fertilizer, and a misting fan was used to maintain relative humidity at or above 70% during the day.
Leaf gas exchange, water potential and foliar ABA
Fully hydrated plants were used to assess initial leaf gas exchange and collect samples for foliar ABA quantification. Leaf gas exchange was measured using an infrared gas analyzer (LI-6800, Licor Biosciences). Conditions in the leaf cuvette were controlled for the duration of the experiment; light intensity was set at 500 μmol m−2 s−1, temperature to 23°C, VPD at 1.0 kPa, and CO2 concentration at 400 μmol mol−1. Following leaf gas exchange assessment, measurements of the water potentials were performed immediately using a Scholander pressure chamber (615D, PMS Instrument Company), and leaves were then harvested for foliar ABA analysis. Samples were weighted, covered in cold 80% methanol in water (v v−1), and immediately stored at −30°C. Samples were further purified, and ABA levels quantified by physicochemical methods with an added internal standard using HPLC-MS according to McAdam.Citation63 Foliar ABA levels were expressed in terms of DW, which was quantified after ABA determination by weighing the dry mass of the sample used for analysis.
After the initial characterization of plants, two individuals were drought stressed by withholding water under greenhouse conditions. One frond from each plant was then enclosed in the cuvette of the LI-6800; the terminal end of the frond was taken for water potential measurements. Using the LI-6800, we measured the kinetics of stomatal reopening following instantaneous rehydration thought the stipe, which took place by the excision of the stipe under water and recutting of the end for the duration of the experiment to ensure no blockage of the xylem occurred from mucilage. After leaf gas exchange stabilization, leaves were collected for final water potential measurements and foliar ABA quantification as described above.
Foliar ABA levels were calculated terms of molarity based on the water potential and relationship between relative water content and leaf water potential determined by pressure–volume curves according to the following equation:
where ABA (M) is ABA on a molar basis, ABA FW unit is ng g−1 FW, RWC ranges from 0 to 1.0, and the molar weight of ABA is 264.321 g mol−1.
Disclosure of Potential Conflicts of Interest
No potential conflicts of interest were disclosed.
Abbreviations
Additional information
Funding
References
- Brodribb TJ, McAdam SAM. Evolution of the stomatal regulation of plant water content. Plant Physiol. 2017;174:639–649. doi:10.1104/pp.16.01930.
- Martin-StPaul N, Delzon S, Cochard H. Plant resistance to drought depends on timely stomatal closure. Ecol Lett. 2017;20:1437–1447. doi:10.1111/ele.12851.
- Kim T, Bohmer M, Hu H, Nishimura N, Schroeder JI. Guard cell signal transduction network : advances in understanding abscisic acid, CO2, and Ca2+ signaling. Annu Rev Plant Biol. 2010;61:561–591. doi:10.1146/annurev-arplant-042809-112226.
- Daszkowska-Golec A, Szarejko I. Open or close the gate - stomata action under the control of phytohormones in drought stress conditions. Front Plant Sci. 2013;4:138. doi:10.3389/fpls.2013.00138.
- Yoshida T, Fernie AR. Remote control of transpiration via ABA. Trends Plant Sci. 2018;23:755–758. doi:10.1016/j.tplants.2018.07.001.
- Cai S, Chen G, Wang Y, Huang Y, Marchant DB, Wang Y, Yang Q, Dai F, Hills A, Franks PJ, et al. Evolutionary conservation of ABA signaling for stomatal closure. Plant Physiol. 2017;174:732–747. doi:10.1104/pp.16.01930.
- Sussmilch FC, Roelfsema MRG, Hedrich R. On the origins of osmotically driven stomatal movements. New Phytol. 2019;222:84–90. In Press. doi:10.1111/nph.2019.222.issue-1.
- Chater C, Kamisugi Y, Movahedi M, Fleming A, Cuming AC, Gray JE, Beerling DJ. Regulatory mechanism controlling stomatal behavior conserved across 400 million years of land plant evolution. Curr Biol. 2011;21:1025–1029. doi:10.1016/j.cub.2011.04.032.
- Edwards D, Kerp H, Hass H. Stomata in early land plants: an anatomical and ecophysiological approach. J Exp Bot. 1998;49:255–278. doi:10.1093/jxb/49.Special_Issue.255.
- Pressel S, Renzaglia KS, Clymo RSD, Duckett JG. Hornwort stomata do not respond actively to exogenous and environmental cues. Ann Bot. 2018;122:45–57. doi:10.1093/aob/mcy045.
- Renzaglia KS, Villarreal JC, Piatkowski BT, Lucas JR, Merced A. Hornwort stomata: architecture and fate shared with 400-million-year-old fossil plants without leaves. Plant Physiol. 2017;174:788–797. doi:10.1104/pp.16.01930.
- Duckett JG, Pressel S. The evolution of the stomatal apparatus: intercellular spaces and sporophyte water relations in bryophytes — two ignored dimensions. Philos Trans R Soc B Biol Sci. 2017;373:20160498. doi:10.1098/rstb.2016.0498.
- Pressel S, Goral T, Duckett JG. Stomatal differentiation and abnormal stomata in hornworts. J Bryol. 2014;36:87–103. doi:10.1179/1743282014Y.0000000103.
- Field KJ, Duckett JG, Cameron DD, Pressel S. Stomatal density and aperture in non-vascular land plants are non-responsive to above-ambient atmospheric CO2 concentrations. Ann Bot. 2015;115:915–922. doi:10.1093/aob/mcv021.
- Duckett JG, Pressel S, Kmy P, Renzaglia KS. Exploding a myth: the capsule dehiscence mechanism and the function of pseudostomata in Sphagnum. New Phytol. 2009;183:1053–1063. doi:10.1111/j.1469-8137.2009.02905.x.
- Kenrick P, Crane PR. The origin and early evolution of plants on land. Nature. 1997;389:33–39. doi:10.1038/37918.
- Hotton CL, Hueber FM, Griffing DH, Bridge JS. Early terrestrial plant paleoenvironments: an example from the Emsian of Gaspé, Canada. In: Gensel PG, Edwards D, editors. Early land plants and their environments. New York: Columbia University Press; 1998.
- Raven JA. Selection pressures on stomatal evolution. New Phytol. 2002;153:371–386. doi:10.1046/j.0028-646X.2001.00334.x.
- Hetherington AM, Woodward FI. The role of stomata in sensing and driving environmental change. Nature. 2003;424:901–908. doi:10.1038/nature01843.
- Raissig MT, Matos JL, Gil MXA, Kornfeld A, Bettadapur A, Abrash E, Allison HR, Badgley G, Vogel JP, Berry JA, et al. Mobile MUTE specifies subsidiary cells to build physiologically improved grass stomata. Science. 2017;355:1215–1218. doi:10.1126/science.aal3254.
- Doi M, Shimazaki K. The stomata of the fern Adiantum capillus-veneris do not respond to CO2 in the dark and open by photosynthesis in guard cells. Plant Physiol. 2008;147:922–930. doi:10.1104/pp.107.115535.
- Brodribb TJ, McAdam SAM. Passive origins of stomatal control. Science. 2011;582–585. doi:10.1126/science.1197985.
- Soni DK, Ranjan S, Singh R, Khare PB, Pathre UV, Shirke PA. Photosynthetic characteristics and the response of stomata to environmental determinants and ABA in Selaginella bryopteris, a resurrection spike moss species. Plant Sci. 2012;191–192:43–52. doi:10.1016/j.plantsci.2012.04.011.
- Hõrak H, Kollist H, Merilo E. Fern stomatal responses to ABA and CO2 depend on species and growth conditions. Plant Physiol. 2017;174:672–679. doi:10.1104/pp.16.01930.
- Grantz DA, Linscheid BS, Grulke NE. Differential responses of stomatal kinetics and steady state conductance to abscisic acid in a fern: comparison with a gymnosperm and an angiosperm. New Phytol. 2019;In Press. doi:10.1111/nph.15736.
- McAdam SAM, Brodribb TJ. Fern and lycophyte guard cells do not respond to endogenous abscisic acid. Plant Cell. 2012;24:1510–1521. doi:10.1105/tpc.112.098962.
- Brodribb TJ, McAdam SAM, Carins Murphy MR. Xylem and stomata, coordinated through time and space. Plant Cell Environ. 2017;40:872–880. doi:10.1111/pce.12817.
- Cardoso AA, Randall JM, McAdam SAM. Hydraulics regulate stomatal responses to changes in leaf water status in the fern Athyrium filix-femina. Plant Physiol. 2019;179:533–543. doi:10.1104/pp.18.01412.
- Christmann A, Hoffmann T, Teplova I, Grill E, Muller A. Generation of active pools of abscisic acid revealed by in vivo imaging of water-stressed Arabidopsis. Plant Physiol. 2005;137:209–219. doi:10.1104/pp.104.053082.
- Yan J, Tsuichihara N, Etoh T, Iwai S. Reactive oxygen species and nitric oxide are involved in ABA inhibition of stomatal opening. Plant Cell Environ. 2007;30:1320–1325. doi:10.1111/pce.2007.30.issue-10.
- Schroeder JI, Allen GJ, Hugouvieux V, Kwak JM, Waner D. Guard cell signal transduction. Annu Rev Plant Physiol Plant Mol Biol. 2001;52:627–658. doi:10.1146/annurev.arplant.52.1.627.
- Wilkinson S, Davies WJ. ABA-based chemical signalling: the co-ordination of responses to stress in plants. Plant Cell Environ. 2002;25:195–210. doi:10.1046/j.0016-8025.2001.00824.x.
- Martins SCV, Mcadam SAM, Deans RM, Damatta FM, Brodribb TJ. Stomatal dynamics are limited by leaf hydraulics in ferns and conifers: results from simultaneous measurements of liquid and vapour fluxes in leaves. Plant Cell Environ. 2016;39:694–705. doi:10.1111/pce.12668.
- Deans RM, Brodribb TJ, Mcadam SAM. An integrated hydraulic-hormonal model of conifer stomata predicts water stress dynamics. Plant Physiol. 2017;174:478–486. doi:10.1104/pp.16.01930.
- Mcadam SAM, Brodribb TJ, Banks JA, Hedrich R, Atallah NM, Cai C, Geringer MA, Lind C, Nichols DS, Stachowski K, et al. Abscisic acid controlled sex before transpiration in vascular plants. Proc Natl Acad Sci U S A. 2016;113:12862–12867. doi:10.1073/pnas.1606614113.
- Ruszala EM, Beerling DJ, Franks PJ, Chater C, Casson SA, Gray JE, Hetherington AM. Land plants acquired active stomatal control early in their evolutionary history. Curr Biol. 2011;21:1030–1035. doi:10.1016/j.cub.2011.04.044.
- De Silva DLR, Cox RC, Hetherington AM, Mansfield TA. The role of abscisic acid and calcium in determining the behaviour of adaxial and abaxial stomata. New Phytol. 1986;104:41–51. doi:10.1111/j.1469-8137.1986.tb00632.x.
- Raschke K. Action of abscisic acid on guard cells. In: Zeiger GDFE, Cowan IR, editors. Stomatal function. Stanford (USA): Stanford Univ Press; 1987. p. 253–279.
- Raghavendra AS, Reddy KB. Action of proline on stomata differs from that of abscisic acid, G-substances, or methyl jasmonate. Plant Physiol. 1987;83:732–734.
- Shen L, Sun P, Bonnell VC, Edwards KJ, Hetherington AM, McAinsh MR, Roberts MR. Measuring stress signaling responses of stomata in isolated epidermis of graminaceous species. Front Plant Sci. 2015;6:553. doi:10.3389/fpls.2015.00533.
- McAdam SAM, Brodribb TJ. The evolution of mechanisms driving the stomatal response to vapor pressure deficit. Plant Physiol. 2015;167:833–843. doi:10.1104/pp.114.252940.
- Mustilli AC, Merlot S, Vavasseur A, Fenzi F, Giraudat J. Arabidopsis OST1 protein kinase mediates the regulation of stomatal aperture by abscisic acid and acts upstream of reactive oxygen species production. Plant Cell. 2002;14:3089–3099.
- Liang YK, Dubos C, Dodd IC, Holroyd GH, Hetherington AM, Campbell MM. AtMYB61, an R2R3-MYB transcription factor controlling stomatal aperture in Arabidopsis thaliana. Curr Biol. 2005;15:1201–1206. doi:10.1016/j.cub.2005.06.041.
- Jung C, Seo JS, Han SW, Koo YJ, Kim CH, Song SI, Nahm BH, Choi YD, Cheong -J-J. Overexpression of AtMYB44 enhances stomatal closure to confer abiotic stress tolerance in transgenic Arabidopsis. Plant Physiol. 2008;146:623–635. doi:10.1104/pp.107.110361.
- Savchenko T, Kolla VA, Wang C-Q, Nasafi Z, Hicks DR, Phadungchob B, Chehab WE, Brandizzi F, Froehlich J, Dehesh K. Functional convergence of oxylipin and ABA pathways controls stomatal closure in response to drought. Plant Physiol. 2014;164:1151–1160. doi:10.1104/pp.113.234310.
- Santamaria JM, Davies WJ, Atkinson CJ. Stomata of micropropagated Delphinium plants respond to ABA, CO2, light and water potential, but fail to close fully. J Exp Bot. 1993;44:99–107. doi:10.1093/jxb/44.1.99.
- Garcıa-Mata C, Lamattina L. Nitric oxide and abscisic acid cross talk in guard cells. Plant Physiol. 2002;128:790–792.
- Huang D, Wu W, Abrams SR, Cutler AJ. The relationship of drought-related gene expression in Arabidopsis thaliana to hormonal and environmental factors. J Exp Bot. 2008;59:2991–3007. doi:10.1093/jxb/ern155.
- Jager CE, Symons GM, Ross JJ, Reid JB. Do brassinosteroids mediate the water stress response? Physiol Plant. 2008;133:417–425. doi:10.1111/j.1399-3054.2008.01057.x.
- Cornish K, Zeevaart JAD. Abscisic acid accumulation by in situ and isolated guard cells of Pisum sativum L. and Vicia faba L. in relation to water stress. Plant Physiol. 1986;81:1017–1021. doi:10.1104/pp.81.4.1017.
- Kitsaki CK, Drossopoulos JB. Environmental effect on ABA concentration and water potential in olive leaves (Olea europaea L. cv “Koroneiki”) under non-irrigated field conditions. Environ Exp Bot. 2005;54:77–89. doi:10.1016/j.envexpbot.2004.06.002.
- Harris MJ, Outlaw WH Jr, Mertens R, Weiler EW. Water-stress-induced changes in the abscisic acid content of guard cells and other cells of Vicia faba L. leaves as determined by enzyme-amplified immunoassay. Proc Natl Acad Sci U S A. 1988;85:2584–2588. doi:10.1073/pnas.85.8.2584.
- Bauer H, Ache P, Lautner S, Fromm J, Hartung W, Al-Rasheid KAS, Sonnewald S, Sonnewald U, Kneitz S, Lachmann N, et al. The stomatal response to reduced relative humidity requires guard cell-autonomous ABA synthesis. Curr Biol. 2013;23:53–57. doi:10.1016/j.cub.2012.11.022.
- Platt JR. Strong inference. Science. 1964;146:347–353. doi:10.1126/science.146.3642.347.
- Takemiya A, Shimazaki K. Phosphatidic acid inhibits blue light-induced stomatal opening via inhibition of protein phosphatase. Plant Physiol. 2010;153:1555–1562. doi:10.1104/pp.110.155689.
- Ma Y, Szostkiewicz I, Korte A, Moes D, Yang Y, Christmann A, Grill E. Regulators of PP2C phosphatase activity function as abscisic acid sensors. Science. 2009;324:1064–1068.
- Loveys BR. The intracellular location of abscisic acid in stressed and non‐stressed leaf tissue. Physiol Plant. 1977;40:6–10. doi:10.1111/ppl.1977.40.issue-1.
- Weyers JDB, Travis AJ. Selection and preparation of leaf epidermis for experiments on stomatal physiology. J Exp Bot. 1981;32:837–850.
- Shope JC, Peak D, Mott KA. Stomatal responses to humidity in isolated epidermes. Plant Cell Environ. 2008;31:1290–1298. doi:10.1111/j.1365-3040.2008.01844.x.
- Lee J, Bowling DJF. Effect of the mesophyll on stomatal opening in Commelina communis. J Exp Bot. 1992;43:951–957. doi:10.1093/jxb/43.7.951.
- Lösch R. Responses of stomata to environmental factors - experiments with isolated epidermal strips of Polypodium vulgate. Oecologia. 1979;39:229–238. doi:10.1007/BF00348071.
- Dimichele WA, Pfefferkorn HW, Gastaldo RA. Response of late carboniferous and early permian plant communities to climate change. Annu Rev Earth Planet Sci. 2001;29:461–487. doi:10.1146/annurev.earth.29.1.461.
- McAdam SAM. Physicochemical quantification of abscisic acid levels in plant tissues with an added internal standard by ultra-performance liquid chromatography. Bio-Protocol. 2015;5:1–13.