ABSTRACT
Apomixis in Hieracium praealtum follows a developmental pathway of apospory, where an unreduced embryo sac develops from a somatic ovule cell without meiosis. The avoidance of meiosis together with fertilization-independent seed formation leads to clonal progeny genetically identical to the maternal plant. We have previously described the initial developmental steps of aposporous embryo sac formation in H. praealtum and here, we cytologically observed more than 500 ovules with a focus on the later stages of embryo sac maturation. Aposporous embryo sac maturation is a stochastic process in H. praealtum with single or multiple embryo sacs formed, in addition to off-types and embryo sac abortion. The frequency of twin embryo sacs growing at the same rate is a rare event and, in most ovules, the additional embryo sac undergoes developmental arrest suggesting dominance or growth promotion of a single embryo sac. Observed deviations from the Polygonum-type embryo sac in H. praealtum indicate developmental plasticity during embryo sac maturation. Nevertheless, fertilization-independent seed formation successfully occurs.
Text
Female sexual development in most (~70%) flowering plants results in the formation of a single meiotically reduced female gametophyte or embryo sac of the Polygonum-type, which contains four distinct cell types including the egg and the central cell.Citation1 These cells fuse independently with two sperm cells during double fertilization to produce the embryo and endosperm in the seed, respectively. By contrast, several modes of apomixis or asexual seed formation result in embryo sac and embryo development without meiosis and fertilization. Therefore, the progeny that arise from apomictic reproduction are genetically identical to the mother plant. Apomixis occurs naturally in flowering plants, and about 75% of all currently known apomicts belong to three families, Asteraceae, Poaceae and Rosaceae.Citation2,Citation3 Apomixis is essentially absent in agriculturally important seed crop plants and is found in fruit trees such as mango, apple and Citrus.Citation4,Citation5
Hieracium praealtum (syn. Pilosella praealtum) is a facultative apomictic tetraploid species of the daisy family Asteraceae, which has been developed as a model to identify genes controlling apomixis.Citation6–Citation11 Apomixis in H. praealtum initiates with the formation of an aposporous initial (AI) cell, which is the somatic ovule cell that gives rise to the unreduced embryo sac via mitosis. This is controlled by the dominant LOSS OF APOMEIOSIS locus, which is activated by early events of sexual meiosis.Citation7,Citation12 Recent evidence by Juranić et al.Citation11 suggests that direct contact between the functional megaspore and AI cell promotes enlargement and rapid mitotic divisions of the AI cell to form an aposporous embryo sac and simultaneously leads to the demise of the sexual pathway. As one to four AI cells may differentiate per ovule, the first expanding AI to contact the functional megaspore will rapidly enlarge at the chalazal end of the ovule, resembling a fifth megaspore and initiating mitosis. While our previous paper focused on the mechanism of AI differentiation and early events of apomixis, this short report builds on previous work and aims to address the later developmental events of the aposporous female gametophyte during mitosis and cellularization. In this context, the focus has been on the occurrence of multiple embryo sacs within the same ovule to assess the success rates of multiple embryo sac maturation and subsequent embryo development.
An ovule clearing method, in combination with differential interference contrast microscopy and toluidine blue stained sections, described previously,Citation7,Citation13 was used to score the cytological observations of embryo sacs during capitulum development at stages 6, 8 and 10, defined by Koltunow et al.Citation14 (, ). The organisation and structure of the embryo sac in H. praealtum often differs from the typical Polygonum-type embryo sac, as antipodal cells are not present and in 13% of all ovules at stage 6, embryo sacs are accompanied by an enlarged AI cell(s) or a multinucleate cell at the chalazal end of the ovule (, ). Five categories of embryo sac types were observed during mitotic events of embryo sac development including a single embryo sac at the micropylar end of the ovule (), a single embryo sac at the chalazal end situated above a degenerated micropylar sac (), twin embryo sacs (), an aborted embryo sac () and off-types (-J) where an embryo formed in the chalazal embryo sac, or endosperm developed without an embryo in the chalazal embryo sac ().
Table 1. Analysis of embryo sac and early seed development in cleared ovaries of apomictic Hieracium praealtum (4x-1 = 35). Total ovules scored represent the number of ovules in five main categories, excluding subcategories.
Figure 1. Representative examples of embryo sac and embryo formation during aposporous development in H. praealtum.
(A) to (E) Cartoons depicting frequent types of embryo sacs observed in ovules of H. praealtum. (A) Embryo sac with enlarged AI cell at the top. (B) Single embryo sac. (C) Collapsed endothelium with embryo sac at the chalazal end. (D) Twin embryo sacs with developing embryos. (E) Aborted embryo sac resulting in collapsed endothelium. (F) to (J) Differential interference contrast images of off-type embryo sacs. (F) Two embryo sacs with embryo developing in the sac at the chalazal end, an AI is present within the top of the micropylar embryo sac. (G) An embryo sac developing endosperm but no embryo, in the top of this embryo sac is another. Bars = 50 µm. (H) Endosperm development without embryo in a chalazal embryo sac. (I) Collapsed endothelium with developing embryo at the chalazal end. (J) Lateral embryo development mid embryo sac. Arrowheads point toward structures within embryo sacs. Bars = 100 µm. (K) to (M) Toluidine Blue sections of ovules from stage 4 to 10. (K) Rare example of two functional AI cells developing at same rate. (L) Stage 6 with mature aposporous embryo sac and enlarged AI cell at the top. Bars = 50 µm. (M) Stage 8 with a globular embryo and enlarged AI cell at the top. (N) Stage 10 with a late globular stage embryo. Bars = 100 µm. Abbreviations: ai, aposporous intial cell; cc, central cell; ch, chalazal end; ec, egg cell; em, embryo; en, endosperm; et, endothelium; fai, functional AI cell; mp, micropylar end; pn, polar nuclei; st, stage.
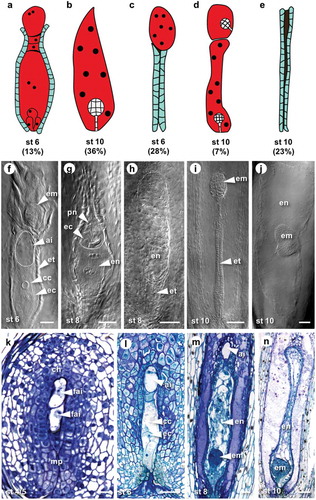
While twin embryo sacs were found to be present in up to 17% of ovules, only in a small proportion (0.7%) were two embryo sacs observed developing to maturity at the same rate (, ). In the majority of cases, the second embryo sac at the chalazal end of the ovule remained immature (, ). Multiple embryos were observed with the frequency of 7% at stage 10 of capitulum development () probably as a result of the differentiation of a second embryo sac during stages 6 to 10 from AI cells which may have resulted from two AI cells contacting the functional megaspore consistent with our previous findings.Citation11 The inhibition of multiple aposporous embryo sacs appears to be facilitated by callose deposition in cell walls between the micropylar aposporous embryo sac and other chalazally located AI cells, thereby forming a physical barrier.Citation11 If this is inefficient two embryo sacs may form. However, the delay in maturation of a second embryo sac while the micropylar-most embryo sac attains maturity suggests mechanisms that may promote micropylar embryo sac formation. The micropylar position of the embryo sac is preferred in Polygonum-type embryo sac formation in sexual reproduction.
Other deviations from single embryo sac development include abortion of the embryo sac characterized by a collapsed and compressed endothelium in 18–26% of examined ovules (, ). This result together with percentages of non-functional chalazal embryo sacs (16%) and off-types (8%) gives about 50% of potentially non-viable ovules () and offers an explanation as to why only 53% of H. praealtum seeds are germinable.Citation7 Observed unstable development of aposporous embryo sacs in H. praealtum raises the question of whether there are developmental penalties associated with apomixis. However, two other aposporous species H. piloselloides and H. caespitosum have higher seed germination rates of 64% and 79%, respectively, suggesting a lower abortion rate in these species.Citation7 Moreover, the early suppression of sexual development and the formation of a single aposporous embryo sac is more prevalent in H. piloselloides where the rates of seedlings derived from meiotic eggs are below 3% indicating a high penetrance of apomixis.Citation14,Citation15
Not all apomicts seem to have mechanisms that directly result in the cellular demise of the sexual pathway as observed in the Hieracium species described above. Co-existence of aposporous and sexually derived embryo sacs is a common occurrence in grasses that undergo apospory including Pennisetum spp., Poa pratensis, Paspalum spp. and Panicum maximum.Citation16–Citation20 In apomictic Paspalum malacophyllum, aposporous embryo sac and parthenogenetic embryo development seem to outcompete the sexual pathway in relation to embryo sac growth rates and seed types formed.Citation19 Other factors such as gene deregulation and ploidy levels have also been suggested to promote aposporous embryo sac growth in Paspalum.Citation19
While this study highlights the developmental plasticity of aposporous embryo sac formation in Hieracium praealtum, the position of the aposporous embryo sac in the micropylar region of the ovule appears preferential for viable seed formation via the apomictic pathway in aposporous Hieracium species. Even though this was not directly investigated previously, preferential asexual embryo formation in the micropylar region also appears to be the case in some aposporous grasses studied, for example, Poa pratensis and Panicum maximum.Citation21,Citation22 This implies that possibly maternally derived developmental and nutritional cues within the ovule, which have evolved to support the micropylar position of the sexually derived Polygonum-type embryo sac, may also be important for seeds formed via the apomictic pathway in some eudicot and monocot apomicts.
Disclosure of Potential Conflicts of Interest
No potential conflicts of interest were disclosed.
Additional information
Funding
References
- Yadegari R, Drews GN. Female gametophyte development. Plant Cell. 2004;16:S133–S141. doi:10.1105/tpc.018192.
- Carman JG. Asynchronous expression of duplicate genes in angiosperms may cause apomixis, bispory, tetraspory, and polyembryony. Bio J Linnean Soc. 1997;61:51–94. doi:10.1111/j.1095-8312.1997.tb01778.x.
- Hojsgaard D, Klatt S, Baier R, Carman JG, Horandl E. Taxonomy and Biogeography of Apomixis in Angiosperms and Associated Biodiversity Characteristics. CRC Crit Rev Plant Sci. 2014;33:414–427. doi:10.1080/07352689.2014.898488.
- Naumova T. Apomixis in Angiosperms: nucellar and integumentary embryony, Ed 1st. Boca Raton (FL, USA): CRC Press; 1993.
- Zhang SQ, Liang M, Wang N, Xu Q, Deng XX, Chai LJ. Reproduction in woody perennial Citrus: an update on nucellar embryony and self-incompatibility. Plant Reprod. 2018;31:43–57. doi:10.1007/s00497-018-0327-4.
- Catanach AS, Erasmuson SK, Podivinsky E, Jordan BR, Bicknell R. Deletion mapping of genetic regions associated with apomixis in Hieracium. Proc Natl Acad Sci USA. 2006;103:18650–18655. doi:10.1073/pnas.0605588103.
- Koltunow AMG, Johnson SD, Rodrigues JCM, Okada T, Hu YK, Tsuchiya T, Wilson S, Fletcher P, Ito K, Suzuki G, et al. Sexual reproduction is the default mode in apomictic Hieracium subgenus Pilosella, in which two dominant loci function to enable apomixis. Plant J. 2011;66:890–902. doi:10.1111/j.1365-313X.2011.04556.x.
- Okada T, Hu YK, Tucker MR, Taylor JM, Johnson SD, Spriggs A, Tsuchiya T, Oelkers K, Rodrigues JCM, Koltunow AMG. Enlarging cells initiating apomixis in Hieracium praealtum transition to an embryo sac program prior to entering mitosis. Plant Physiol. 2013;163:216–231. doi:10.1104/pp.113.219485.
- Rabiger DS, Taylor JM, Spriggs A, Hand ML, Henderson ST, Johnson SD, Oelkers K, Hrmova M, Saito K, Suzuki G, et al. Generation of an integrated Hieracium genomic and transcriptomic resource enables exploration of small RNA pathways during apomixis initiation. BMC Biol. 2016;14. doi:10.1186/s12915-016-0311-0.
- Bräuning S, Catanach A, Lord JM, Bicknell R, Macknight RC. Comparative transcriptome analysis of the wild-type model apomict Hieracium praealtum and its loss of parthenogenesis (lop) mutant. BMC Plant Biol. 2018;18:206. doi:10.1186/s12870-018-1423-1.
- Juranić M, Tucker MR, Schultz CJ, Shirley NJ, Taylor JM, Spriggs A, Johnson SD, Bulone V, Koltunow AM. Asexual female gametogenesis involves contact with a sexually-fated megaspore in apomictic Hieracium. Plant Physiol. 2018;177:1027–1049. doi:10.1104/pp.18.00342.
- Okada T, Ito K, Johnson SD, Oelkers K, Suzuki G, Houben A, Mukai Y, Koltunow AM. Chromosomes carrying meiotic avoidance loci in three apomictic eudicot Hieracium subgenus Pilosella species share structural features with two monocot apomicts. Plant Physiol. 2011;157:1327–1341. doi:10.1104/pp.111.181164.
- Koltunow AM, Johnson SD, Bicknell RA. Apomixis is not developmentally conserved in related, genetically characterized Hieracium plants of varying ploidy. Sex Plant Reprod. 2000;12:253–266. doi:10.1007/s004970050193.
- Koltunow AM, Johnson SD, Bicknell RA. Sexual and apomictic development in Hieracium. Sex Plant Reprod. 1998;11:213–230. doi:10.1007/s004970050144.
- Bicknell RA, Lambie SC, Butler RC. Quantification of progeny classes, in two facultatively apotnictic accessions of Hieracium. Hereditas. 2003;138:11–20.
- Nakagawa H. Embryo sac analysis and crossing procedure for breeding apomictic guineagrass (panicum-maximum jacq). Jarq-Jpn Agr Res Q. 1990;24:163–168.
- Albertini E, Porceddu A, Ferranti F, Reale L, Barcaccia G, Romano B, Falcinelli M. Apospory and parthenogenesis may be uncoupled in Poa pratensis: a cytological investigation. Sex Plant Reprod. 2001;14:213–217. doi:10.1007/s00497-001-0116-2.
- Ozias-Akins P. Apomixis: Developmental characteristics and genetics. CRC Crit Rev Plant Sci. 2006;25:199–214. doi:10.1080/07352680600563926.
- Hojsgaard DH, Martinez EJ, Quarin CL. Competition between meiotic and apomictic pathways during ovule and seed development results in clonality. New Phytol. 2013;197:336–347. doi:10.1111/j.1469-8137.2012.04381.x.
- Rios E, Kenworthy K, Blount A, Quesenberry K, Unruh B, Erickson J, Altpeter F, Munoz P. Breeding apomictic bahiagrass (Paspalum notatum Flugge) with improved turf traits. Plant Breed. 2017;136:253–260. doi:10.1111/pbr.12459.
- Yudakova OI, Shakina TN. Specific features of early embryogenesis in apomictic Poa pratensis L. Russ J Dev Biol. 2007;38:1–6. doi:10.1134/S1062360407010018.
- Chen L, Guan L. Ultrastructural mechanisms of aposporous embryo sac initial cell appearance and its developmental process in gametophytic apomicts of guinea grass (panicum maximum). In The Transmission Electron Microscope, editor. Maaz K, IntechOpen (London, UK), 2012;233. doi:10.5772/34912.