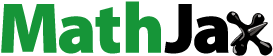
ABSTRACT
Salicylic acid (SA) may improve plant tolerance to abiotic stresses; however, little is known about the underlying mechanisms by which this is achieved. Here, we investigated the effects of exogenous SA application on seed germination in the halophyte Limonium bicolor (Kuntze) under salt stress. Specifically, we examined the effect of salt stress on seed germination, sugar and protein contents, amylase activity, and the contents of various hormones, both in the presence and absence of exogenous SA treatments. Germination was significantly suppressed by a 200 mM NaCl treatment but was significantly improved when 0.08 mM SA was concurrently applied. During germination, the seeds treated with SA had high levels of gibberellic acid (GA) and high levels of amylase and α-amylase activity, but low abscisic acid (ABA) contents. The SA treatment upregulated the expression of key genes involved in GA biosynthesis while downregulating those involved in ABA biosynthesis, thereby triggering a favorable hormonal balance between GA and ABA that enhanced seed germination under salt stress.
Introduction
Salt stress, a form of abiotic stress, substantially inhibits seed germination and constrains crop and biomass production.Citation1,Citation2,Citation3 Seed germination is a fragile and vital period in the life cycle of a plant and is directly related to the subsequent morphogenesis and growth of the seedling.Citation4–Citation8 A series of events occur during germination, including the uptake of water by the dry quiescent seed, the upregulation of many genes, the hydrolysis of macromolecular substances into smaller compounds, the establishment of a new embryonic structure, and the emergence of the radicle through the seed coat.Citation7,Citation9
Germination is a complex trait affected by both developmental and environmental factors.Citation6,Citation10–Citation12 The germination of a normal, well-developed seed is influenced by environmental factors, such as salt, drought, waterlogging, and hypoxia. The effects of salt stress on seed germination are particularly ubiquitous and extremely complex Zhang et al.Citation13–Citation15 High salinity in soils inhibits germination by reducing the soil water potential, which impairs the ability of seeds to absorb water, thus suppressing seed imbibition and embryo growth. The absorption of excessive concentrations of ions from saline soils also inhibits embryo development and the formation of new organs.Citation16–Citation19
Limonium bicolor (Kuntze) is a diploid perennial herb of the Plumbaginaceae family.Citation20,Citation21 The species is distributed throughout Northeast China, the Yellow River Basin provinces, northern Jiangsu Province, and Mongolia. The seeds and the plant as a whole have medicinal value (hemostasis and enriching blood), while the florets have great economic value (for flower production). L. bicolor is a halophyte that excretes excess salts through glands located on the leaf blades,Citation20,Citation21 enabling it to survive on saline soils in which other plants (including crops) cannot normally grow. This adaptation makes L. bicolor a pioneer plant for the improvement and utilization of saline-alkali land;Citation21,Citation22 however, despite its salt tolerance, its seeds still struggle to germinate when faced with high salt stress.Citation23–Citation25
Salicylic acid (SA) is a phytohormone that regulates the growth and development of plantsCitation23–Citation27 as well as their responses to stresses such as heat, salt, osmotic, and oxidative stress.Citation27–Citation32 Borsani et al.Citation31 and Eltayeb et al.,Citation28 showed that the exogenous application of SA can reduce the damage caused to plants by salt and osmotic stress. Two other phytohormones, abscisic acid (ABA) and gibberellic acid (GA), interact to regulate seed germination.Citation17,Citation33,Citation34 ABA functions as a negative regulator of this process, while GA positively regulates germination and releases seed dormancy. Little is known about the effects of SA on the ABA and GA contents of seeds during germination, which could be particularly important for the halophytes.
In this study, we applied exogenous SA to L. bicolor seeds to explore its effects on germination, particularly in seeds under salt stress, and to determine the physiological parameters affected by this treatment. The results of this study improve our understanding of the mechanism by which SA enhances plant tolerance to salt stress.
Materials and methods
Plant material and seed germination
All experiments were performed in a glasshouse at the Shandong Normal University, Jinan (36°67΄N, 116°98΄E), China, and the L. bicolor seeds were collected from Dongying, Shandong (37°43΄N, 118°67΄E), China. The seeds were first sterilized with 5% sodium hypochlorite for 5 min and then thoroughly rinsed with distilled water. The seeds were germinated in Petri dishes (50 seeds per dish) on filter paper soaked with 1/5 strength Hoagland nutrient solution containing 0 or 200 mM NaCl and 0, 0.08, 0.12, 0.16, 0.20, 0.24, or 0.28 mM SA. After 7 days, the various experimental indicators were measured.
Germination calculations
The germination indicators were quantified for three independent experiments, with 50 seeds per replicate. Germination was determined as the emergence of the radicle from the seed.
The calculation formula for each indicator data is as follows:
In the formula, Gt is the number of seeds germinated at a given time (Dt) measured in days after the start of the germination test.
Extraction and quantification of ion contents
A total of 0.3 g of seeds (including seed coats and embryos) from each treatment was placed in a test tube and mixed with 5 mL of distilled water, after which the mixture was boiled for 4 h. After cooling, the mixture was transferred to a 25-mL volumetric flask, and the Na+ and Cl – contents were measured using an ICS-1100 Ion Chromatography System (Dionex Corp., Sunnyvale, CA, USA).
Extraction and quantification of SA, GA, and ABA contents
The treated seed samples (15 mg) were homogenized in 1 mL of PBS (phosphate-buffered saline; pH 7.4) and shaken overnight at 4°C. The homogenate was centrifuged at 12,000 g for 10 min at 4°C, and the supernatant was used for the determination of the SA, GA, and ABA contents using plant SA, GA, and ABA enzyme-linked immunoassay kits, respectively (double antibody sandwich method; Huding Biological Technology Co., Shanghai, China). The specific steps were as follows: after loading the samples onto the enzyme label-coated plate, the reaction was carried out at 37°C for 30 min. Next, the enzyme label-coated plate was washed five times with washing buffer, the enzyme standard reagent was added, and the reaction was carried out at 37°C for 30 min. After five washes with washing buffer, a chromogenic solution was added and carried out at 37°C for 10 min. A stop solution was added to terminate the reaction, and the absorbance was measured at a wavelength of 450 nm.Citation35
Extraction and analysis of soluble sugar contents
To determine the sugar levels in the treated seeds, a 15-mg seed sample (fresh weight) was ground in 2 mL of 80% (v/v) ethanol solution. The homogenate was heated in a water bath (75°C) for 10 min and centrifuged at 5,000 g for another 10 min to remove the insoluble residue. The supernatant was used to produce a reaction solution, which comprised 0.5 mL of supernatant, 1.5 mL of neutral distilled water, 0.5 mL of anthrone reagent (1 g in 50 mL ethyl acetate), and 5 mL of concentrated sulfuric acid. The reaction solution was shaken and placed in a boiling water bath for 10 min. The reaction solution was then cooled to room temperature and its light absorption at 620 nm was measured using a UV spectrophotometer. The concentration of total soluble sugars was then determined according to Zhang.Citation36
Preparation of protein extracts
Proteins were extracted for use in a native PAGE as described by Bradford.Citation37 Briefly, 0.3 g of each seed sample was added to 3 mL of precooled extraction medium (50 mM PBS [pH 7.0] and 1% polyvinylpyrrolidone) and ground into a homogenate, which was then centrifuged at 15,000 g for 10 min at 4°C to obtain a supernatant. The supernatant was concentrated on an Amicon ULTRA 0.5-mL 10-kDa Ultracel-PL membrane-centrifugal filter (Millipore, UFC501096) during a 30-min centrifugation at 20,000 g at 10°C to obtain a high-purity protein sample. The protein concentration in the extract was determined using the Bradford, Citation37method.
Amylase and α-amylase activity assays
Amylase activity was measured as described by Jones and Varner.Citation38 Briefly, 0.03 g of each seed sample was ground in 3 mL of neutral distilled water to obtain the crude enzyme extract, which was then centrifuged at 5,000 g for 10 min. The supernatant was used to measure the amylase and α-amylase activity. The α-amylase activity assay was performed as follows: 1 mL of the enzyme extract was heated in a water bath at 70°C (±0.5°C) for 15 min and then cooled to room temperature. Next, 1 mL of citrate buffer (pH 5.6) and 4 mL of 0.4 M NaOH were added to the samples, which were shaken for 2 to 3 min and left to stand for 2 min. Immediately afterward, 2 mL of 1% starch was added to terminate the reaction, and the α-amylase activity was determined according to the light absorption of the sample at 620 nm. The enzyme units were determined as described by Jones and Varner.Citation38 The amylase activity assay was identical to the α-amylase activity, except that it was not heated at 70°C for 15 min.
Quantitative real-time PCR analysis
Total RNA was extracted from germinating seeds treated 2 days with salt, both in the presence and absence of exogenous SA treatments using the total plant RNA extraction kit (Vazyme, Nanjing, China) according to the manufacturer’s protocol. First-strand cDNA was synthesized using purified total RNA as a template, with oligo(dT)18 and random primers used as primers. This reaction was carried out at 37°C for 1 h in a total reaction volume of 20 μL to provide a final cDNA concentration of 200 to 500 ng/mL. The quantitative real-time PCR (qPCR) reaction mix comprised the following: 1 μL of cDNA as a template, 8.2 μL of double distilled water, 0.4 μL of forward primer (10 pmol/μL) (), 0.4 μL of reverse primer (10 pmol/μL) (), and 10 μL of ChamQ Universal SYBR qPCR master mix (Vazyme, Najing city, China). The qPCR assays were performed in a 96-well plate with a commercial SYBR-Green master mix kit (2 x ChamQ Universal SYBR qPCR Master Mix, Takara Bio, Kusatsu, Japan) using a fluorescence quantitative gene amplification instrument (LightCycler 480, Roche, Germany). The expression levels of the GA biosynthesis genes GA-20 oxidase and GA-3 oxidase (GA20ox) and (GA3ox) and the ABA biosynthesis genes NINE-CIS-EPOXYCAROTENOID DIOXYGENASE1 (NCED1) and NCED3 were analyzed according to Lin et al.Citation35 TUBULIN was used as the internal standard.
Table 1. Primers of ABA synthesis and decomposition, GA synthesis key genes for qPCR.
Statistical analysis
The data were analyzed using a two-way analysis of variance (ANOVA), and post hoc Duncan’s multiple range tests were conducted to identify any significant (P < .05) differences between the samples in different experimental conditions.
Results
Exogenous SA application promotes L. bicolor seed germination
The germination rate and germination potential of L. bicolor seeds significantly decreased when treated with 200 mM NaCl, while the germination rate, germination potential, and germination index of the seeds significantly increased when treated with 0.08 to 0.28 mM SA compared to the 0 mM SA condition (). Under the 200 mM NaCl treatment, increasing levels of SA caused the germination rate and germination potential of the seeds to first increase and then decrease. The germination index of seeds exposed to 200 mM NaCl was not significantly different when treated with no or low concentrations of SA but was significantly decreased in seeds treated with higher concentrations of SA. These results showed that a suitable concentration of exogenous SA can promote the germination of L. bicolor seeds under salt stress. The 0.08 and 0.24 mM SA treatments were therefore determined to be the optimum and inhibitory concentrations, respectively, for seed germination under salt stress and were used for the subsequent experiments.
Figure 1. Germination of L. bicolor seeds after 6 days of treatment with various concentrations of SA (0, 0.08, 0.12, 0.16, 0.20, 0.24, 0.28 mM): Germination rate (a), Germination potential (b), Germination index (c). Values are means ± SD of three replicates (n = 3). Bars with the different letters are significantly different at P < .05 according to Duncan’s multiple range tests.
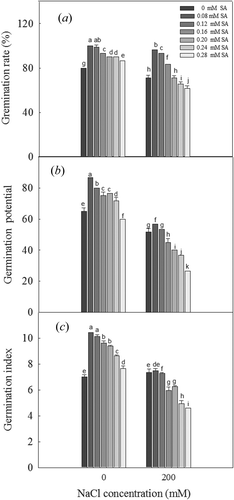
Exogenous SA application influences the SA content of the seed
The SA contents in the L. bicolor seeds were measured after the SA and NaCl treatments. The SA concentration was found to significantly increase in response to the 200 mM NaCl treatment even in the absence of SA application (). When exogenous SA was applied to the L. bicolor seeds, their SA concentrations significantly increased both in the presence and absence of salt stress. Under the 0 mM NaCl condition, the concentration of SA in the seeds increased when treated with increasing concentrations of exogenous SA, but under the 200 mM NaCl treatment, the optimal concentration of exogenous SA (0.08 mM), but not the higher concentration (0.24 mM), increased the SA concentration in the seed.
Figure 2. Salicylic acid concentration in L. bicolor seeds after two days of treatment with different concentrations of SA (0, 0.08, 0.24 mM) under 0 and 200 mM NaCl conditions. Values are means ± SD of three biological replicates (n = 3). Bars labeled with different letters are significantly different at P < .05 according to Duncan’s multiple range tests.
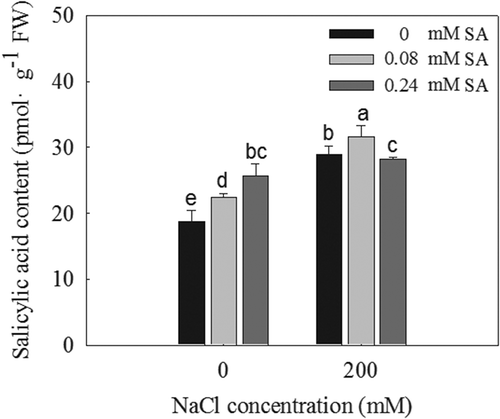
Exogenous SA application increases the Na+ contents of L. bicolor seeds subjected to salt stress
The Na+ and Cl – contents in the seeds, seed coats, and embryos were significantly increased in seeds under the 200 mM NaCl treatment relative to their contents in seeds exposed to 0 mM NaCl (). When combined with the 0 mM NaCl treatment, exogenous SA did not affect the Na+ and Cl – contents in the seeds, seed coats, or embryos; however, under the 200 mM NaCl treatment, both concentrations of exogenous SA significantly increased the Na+ contents of the seeds. Specifically, 0.08 mM SA increased the Na+ contents of the salt-stressed seed coats but not the embryos, and 0.24 mM SA increased the Na+ contents of the salt-stressed embryos and decreased the Na+ content of the seeds coats. Exogenous SA application doesn’t change the Cl− contents of L. bicolor seeds subjected to salt stress
Figure 3. Na+ content in seeds (a), coats (c), embryos (e), and Cl− content in seeds (b), coats (d), embryos (f) of L. bicolor seeds after 2 days of treatment with different concentrations of SA (0, 0.08, 0.24 mM) under 0 and 200 mM NaCl. Values are means ± SD of three replicates (n = 3). Bars with the different letters are significantly different at P < .05 according to Duncan’s multiple range tests.
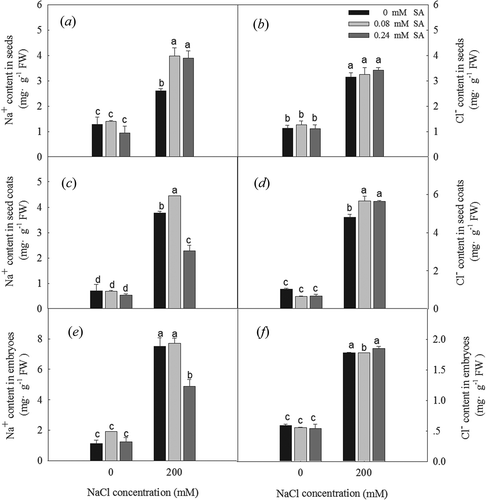
Exogenous SA application increased the sugar contents and reduced the protein contents of L. bicolor seeds subjected to salt stress
Changes in the soluble sugar and protein contents of the L. bicolor seeds during germination are shown in . Compared to the control, the sugar content of L. bicolor seeds under salt stress increased significantly, while the 0.08 and 0.24 mM SA treatments were found to significantly decrease the sugar contents in the seeds (). Compared to the control, the protein contents in the germinated seeds decreased significantly under the salt treatment, but increased in response to the 0.08 and 0.24 mM SA treatments (). This indicated that 200 mM NaCl and 0.24 mM SA inhibited the use of soluble sugars and the production of new proteins in the germinating seeds, which resulted in the accumulation of soluble sugars and a reduced protein content.
Figure 4. Soluble sugar content (a) and total protein content (b) of L. bicolor seeds after 2 days of treatment with different concentrations of SA (0, 0.08, 0.24 mM) under 0 and 200 mM NaCl. Values are means ± SD of three replicates (n = 3). Bars with the different letters are significantly different at P < .05 according to Duncan’s multiple range tests.
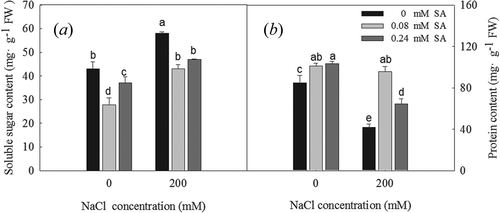
Exogenous SA application alters the amylase and α-amylase activity levels in L. bicolor seeds
The amylase () and α-amylase () activity levels were determined in the germinated L. bicolor seeds, revealing that they were significantly inhibited by the 200 mM NaCl treatment. The activity levels of amylase and α-amylase were significantly increased when treated with 0.08 mM SA under the control and 200 mM NaCl conditions, while α-amylase activity was significantly decreased in seeds treated with 0.24 mM SA under the control and 200 mM NaCl conditions. The 0.08 mM SA treatment therefore increases amylase and α-amylase activity in germinating seeds exposed to salt stress.
Figure 5. Amylase activity (a) and α-amylase activity (B) of L. bicolor seeds after 2 days of treatment with different concentrations of SA (0, 0.08, 0.24 mM) under 0 and 200 mM NaCl. Values are means ± SD of three replicates (n = 3). Bars with the different letters are significantly different at P < .05 according to Duncan’s multiple range tests.
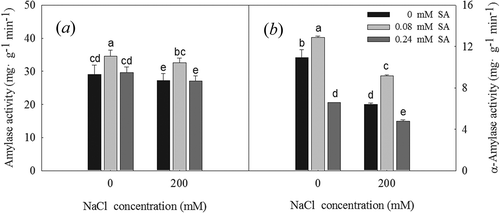
Exogenous SA application reduces the ABA contents and increases the GA contents of L. bicolor seeds
We next explored the effect of SA on the ABA and GA contents of the germinating seeds. The 200 mM NaCl treatment significantly increased the ABA content and reduced the GA content of the germinated seeds compared to the control condition (). This was also true of the 0.24 mM SA treatment; however, the 0.08 mM SA treatment significantly reduced the ABA content and increased the GA content in the seeds. These data suggest that SA affects the ABA and GA contents during seed germination.
Figure 6. ABA concentration (a) and GA (b) concentration of L. bicolor seeds after 2 days of treatment with different concentrations of SA (0, 0.08, 0.24 mM) under 0 and 200 mM NaCl. Values are means ± SD of three replicates (n = 3). Bars with the different letters are significantly different at P < .05 according to Duncan’s multiple range tests.
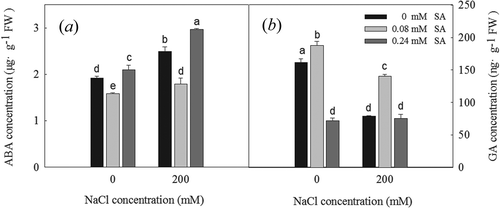
Exogenous SA application alters the expression of ABA and GA biosynthesis genes in L. bicolor seeds
To investigate how SA regulates the ABA and GA levels in response to salt stress, qRT-PCR was performed to analyze the expression of key genes involved in ABA (NCED1 and NCED3) and GA (GA20ox and GA3ox) biosynthesis in the L. bicolor seeds. After 2 days of germinating, NCED1 and NCED3 were significantly upregulated in seeds treated with 200 mM NaCl but were significantly downregulated in seeds treated with 0.08 mM SA alone or in combination with 200 mM NaCl, relative to the control condition ().
Figure 7. Effects of SA (0, 0.08 mM) on transcript levels of key enzymes genes involved in GA biosynthesis, ABA synthesis during germination of L. bicolor seeds under NaCl stress. Tubulin acted as the internal standard.
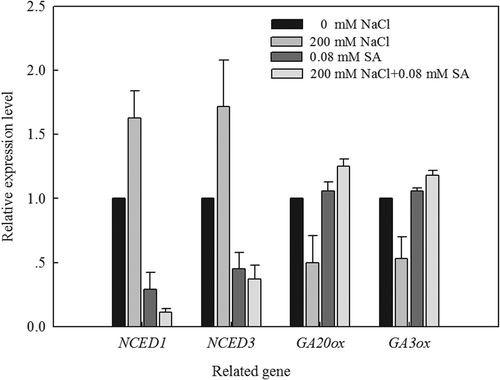
The expression levels of GA20ox and GA3ox were significantly downregulated in the seeds treated with 200 mM NaCl, but were significantly upregulated in those treated with 0.08 mM SA alone or in combination with 200 mM NaCl, relative to the control condition (), which contributed to the increased GA content.
Discussion
Salt stress is a prime adverse factor reducing plant growth and crop yields.Citation1,Citation3 The germination rate and germination potential of seeds, even those of halophytes, Citation8,Citation39,Citation40 is often inhibited by salt in the soil.Citation7,Citation41 Salt stress mainly causes osmotic stress and ion toxicity in plants.Citation7 Osmotic stress is believed to be particularly important in the inhibition of seed germination ;Citation8,Citation39,Citation40 for example, a seed germination and rehydration test indicated that the low germination rate of seeds under salt treatments was mainly due to the osmotic effect.Citation42 In this study, we found that a salt stress treatment significantly increased the Na+ content in the seeds.
As a plant growth regulator, SA was shown to overcome the inhibitory effect of salt stress on rice (Oryza sativa) germination by reducing the starch content in the grain,Citation43 while the application of exogenous SA was also found to alleviate the effects of salt stress on the germination of French marigold seeds (Tagetes patula L.).Citation39 In this study, we showed that soaking L. bicolor seeds with an appropriate concentration of SA (0.08 mM) could increase their germination rate (). Although the 0.08 mM SA treatment significantly increased the Na+ content of the salt-stressed seeds, this increase was mainly confined to the seed coats, not in the embryos (). This compartmentalization protects the embryo from Na+ poisoning and promotes germination.
L.bicolor seeds subjected to NaCl stress had greater soluble sugar contents than those in the control condition (), while seeds exposed to the 0.08 mM SA treatment had significantly lower soluble sugar contents than those exposed to salt stress. These differences in soluble sugar contents are closely related to the observed differences in germination rates, as was previously reported by Balibrea et al.Citation44 in tomato (Solanum lycopersicum) and by Khodary,Citation45 in maize (Zea mays). High SA contents in the seeds can promote the use of soluble sugars in the formation of new structures to stimulate the germination of L. bicolor seeds (, , ). Our exogenous SA treatment alleviated the inhibition of the formation of new proteins in seeds exposed to salt stress, thereby increasing their protein content to stimulate germination. By contrast, Singh,Citation46 reported that the soluble protein content decreased in water-stressed wheat (Triticum aestivum) seeds treated with (1 or 2 mM) SA. This may be due to differences in the mechanisms of water stress and salt stress or differences between halophytes and nonhalophyte plants. In this study, we found that an appropriate concentration of exogenous SA may stimulate the hydrolysis of carbohydrate storage substances and the formation of new proteins.
GA and ABA play important regulatory roles in germination.Citation33,Citation34 Here, we showed that the salt stress treatment decreased the GA concentration and increased the ABA concentration in the L. bicolor seeds, inhibiting their germination (). Sun,Citation47 reported that an appropriate concentration of GA in the seeds is critical for their germination, particularly in salt-stressed seeds, in which GA can increase the content of amylase and promote its activity. Maintaining the GA/ABA balance is important during the early stages of germination in salt-stressed seeds.Citation48 Here, we found that a suitable concentration of SA upregulated the expression of the key genes (GA20ox and GA3ox; ) of GA biosynthesis and increased the GA content of the seeds during germination, as was previously shown by Ogawa et al.Citation49 The key regulatory enzymes in ABA biosynthesis are the NCEDs .Citation50 We showed that the key genes encoding these enzymes in L. bicolor, LbNCED1 and LbNCED3, were downregulated during seed germination in the SA-treated seeds under salt stress, which resulted in the decreased ABA contents observed in the seeds.
Briggs et al.Citation15 suggested that increased levels of GA in the seeds promotes the biosynthesis of hydrolases (especially α-amylase). During the germination of the L. bicolor seeds, we showed that the amylase activity was greater when they were exposed to a pretreatment of SA compared to with NaCl stress alone (). An increase in amylase activity suggested that SA protects enzyme activity from being inhibited by high levels of salinity ().
In this study, SA was found to be positively involved in the germination of L. bicolor seeds under salt stress. Its exogenous application increased the amount of soluble protein and sugar decomposition in the seeds of this halophyte, thereby improving their germination rate. The SA treatment also affected the expression of the GA and ABA biosynthesis genes, which contributed to changes in the GA and ABA contents. This is the first study to elucidate the mechanism by which SA reduces the inhibition of salt stress on the germination of halophyte seeds and provides further insights into the role of SA in the response of halophytes to adverse conditions.
Author Contribution
Jing Liu and Lingyu Li wrote this manuscript; Jing Liu and Lingyu Li performed experiments; Jing Liu and Lingyu Li collected data, carried out all analyses and were involved in preparing figures; Fang Yuan and Min Chen conceptualized the idea. All authors read and approved the final manuscript.
Conflict of Interest
The authors declare that they have no competing interests.
Additional information
Funding
References
- Zhu JK. Plant salt tolerance. Trends Plant Sci. 2001;6:66–71.
- Munns R, James RA, Läuchli A. Approaches to increasing the salt tolerance of wheat and other cereals. J Exp Bot. 2006;57:1025–1043. doi:10.1093/jxb/erj100.
- Hasegawa PM. Sodium (Na+) homeostasis and salt tolerance of plants. Environ Exp Bot. 2013;92:19–31. doi:10.1016/j.envexpbot.2013.03.001.
- Song J, Zhou J, Zhao W, Xu HL, Wang FX, Xu YG, Wang L, Tian CY. Effects of salinity and nitrate on production and germination of dimorphic seeds applied both through the mother plant and exogenously during germination in Suaeda salsa. Plant Spec Biol. 2016;31:19–28. doi:10.1111/1442-1984.12071.
- Guo JR, Suo S, Wang BS. Sodium chloride improves seed vigour of the euhalophyte Suaeda salsa. Seed Sci Res. 2015;25:335–344. doi:10.1017/S0960258515000239.
- Song J, Shi W, Liu R, Xu YG, Sui N, Zhou JC, Feng G. The role of the seed coat in adaptation of dimorphic seeds of the euhalophyte Suaeda salsa to salinity. Plant Spec Biol. 2017;32:107–114. doi:10.1111/1442-1984.12132.
- Ibrahim EA. Seed priming to alleviate salinity stress in germinating seeds. J Plant Physiol. 2016;15:38–46. doi:10.1016/j.jplph.2015.12.011.
- Gul B, Ansari R, Flowers TJ, Khan MA. Germination strategies of halophyte seeds under salinity. Environ Exp Bot. 2013;92:4–18. doi:10.1016/j.envexpbot.2012.11.006.
- Bewley JD, Black M. Seeds: physiology of development and germination. NewYork: PlenumPress; 1994.
- Bentsink L, Koornneef M. Seed dormancy and germination. Curr Opin Plant Biol. 2002;5(1):33–36. doi:10.1199/tab.0119.
- Wang F, Xu YG, Wang S, Shi W, Liu R, Feng G, Song J. Salinity affects production and salt tolerance of dimorphic seeds of Suaeda salsa. Plant Physiol Bioch. 2015;95:41–48. doi:10.1016/j.plaphy.2015.07.005.
- Xu YG, Zhao YQ, Duan HM, Sui N, Yuan F, Song J. Transcriptomic profiling of genes in matured dimorphic seeds of euhalophyte Suaeda salsa. BMC Genomics. 2017;18(1):727–741. doi:10.1186/s12864-017-4104-9.
- Zhang S, Song J, Wang HFeng, G. Effect of salinity on seed germination, ion content and photosynthesis of cotyledons in halophytes or xerophyte growing in Central Asia. J Plant Ecol. 2010;3:259–267. doi:10.1093/jpe/rtq005.
- Zhao YQ, Yang Y, Song YP, Li Q, Song J. Analysis of storage compounds and inorganic ions in dimorphic seeds of euhalophyte Suaeda salsa. Plant Physiol Bioch. 2018;130:511–516. doi:10.1016/j.plaphy.2018.08.003.
- Briggs DE. Biochemistry of barley germination action of gibberellic acid on barley endosperm. J I Brewing. 1963;69(1):13–19. doi:10.1002/j.2050-0416.1963.tb06345.x.
- Zhu JK, Hasegawa PM, Bressan RA. Molecular aspects of osmotic stress in plants. Crit Rev Plant Sci. 1997;16:25. doi:10.1080/07352689709701950.
- Rajjou L, Duval M, Gallardo K, Catusse J, Bally J, Job C, Job D. Seed germination and vigor. Ann Rev Plant Boil. 2012;63:507–533. doi:10.1146/annurev-arplant-042811-105550.
- Shao Q, Han N, Ding T, Zhou F, Wang BS. SsHKT1;1 is a potassium transporter of the C3 halophyte Suaeda salsa that is involved in salt tolerance. Funct Plant Bi. 2014;41:790–802. doi:10.1071/FP13265.
- Zhang T, Song J, Fan J, Feng G. Effects of saline-waterlogging and dryness/moist alternations on seed germination of halophyte and xerophyte. Plant Spec Biol. 2015;30:231–236. doi:10.1111/1442-1984.12056.
- Yuan F, Lyu MJA, Leng BY, Zhu XG, Wang BS. The transcriptome of NaCl-treated Limonium bicolor, leaves reveals the genes controlling salt secretion of salt gland. Plant Mol Biol. 2016;91:241–256. doi:10.1007/s11103-016-0460-0.
- Yuan F, Lyu MJ, Leng BY, Zheng GY, Feng ZT, Li PH, Zhu XG, Wang BS. Comparative transcriptome analysis of developmental stages of the Limonium bicolor leaf generates insights into salt gland differentiation. Plant Cell Environ. 2015;38:1637–1657. doi:10.1111/pce.12514.
- Song J, Wang BS. Using euhalophytes to understand salt tolerance and to develop saline agriculture: suaeda salsa as a promising model. Ann Bot. 2015;115:541–553. doi:10.1093/aob/mcu194.
- Khan MIR, Mehar F, Per TS, Anjum NA, Khan NA. Salicylic acid-induced abiotic stress tolerance and underlying mechanisms in plants. Front Plant Sci. 2015;6:462–479. doi:10.3389/fpls.2015.00462.
- Csiszár J, Brunner S, Horváth E, Krisztina. B, Ködmön P, Riyazuddin R, Ágnes. G, Hurton Á, Csaba. P, Szabados L, et al. Exogenously applied salicylic acid maintains redox homeostasis in salt-stressed Arabidopsis gr1 mutants expressing cytosolic roGFP1. Plant Growth Regul. 2018;86:181–194. doi:10.1007/s10725-018-0420-6.
- Völz R, Kim SK, Mi JN, Mariappan KG, Guo XJ. The Trihelix transcription factor GT2-like 1 (GTL1) promotes salicylic acid metabolism, and regulates bacterial-triggered immunity. PLoS Genet. 2018;14:10. doi:10.1371/journal.pgen.1007708.
- Raskin I. Role of salicylic acid in plants. Ann Rev Plant Physiol and Plant. Mol Biol. 1992;43:439–463. doi:10.1146/annurev.pp.43.060192.002255.
- Dat JDH, Foyer CH, Scott IM. Effects of salicylic acid on oxidative stress and thermo tolerance in tobacco. J Plant Physiol. 2000;156(5):659–665. doi:10.1016/S0176-1617(00)80228-X.
- Eltayeb MA. Response of barley grains to the interactive e.ect of salinity and salicylic acid. Plant Growth Regul. 2005;45(3):215–224. doi:10.1007/s10725-005-4928-1.
- Shim IS, Momose Y, Yamamoto A. Inhibition of catalase activity by oxidative stress and its relationship to salicylic acid accumulation in plants. Plant Growth Regul. 2003;39(3):285–292. doi:10.1023/A:1022861312375.
- Clarke SM, Mur LAJ, Wood JE. Salicylic acid dependent signaling promotes basal thermo tolerance but is not essential for acquired thermo tolerance in Arabidopsis thaliana. The Plant J. 2004;38(3):432–447. doi:10.1111/j.1365-313X.2004.02054.x.
- Borsani O, Valpuesta V, Botella MA. Evidence for a role of salicylic acid in the oxidative damage generated by NaCl and osmotic stress in arabidopsis seedlings. Plant Physiol. 2001;126(3):1024–1030. doi:10.1104/pp.126.3.1024.
- Cutt JR, Klessig DF. Salicylic acid in plants: A changing perspective. Pharmaceu. Technol. 1992;16:25–34.
- Sunkar R, Zhu JK. Abscisic Acid (ABA)[J]. Encycl Biol Chem. 2004:6–11. doi:10.1016/B0-12-443710-9/00001-6.
- Rosental L, Nonogaki H, Fait A. Activation and regulation of primary metabolism during seed germination. Seed Sci Res. 2014;24:1–15. doi:10.1017/S0960258513000391.
- Lin J, Li JP, Yuan F, YANG Z, WANG BS, CHEN M. Transcriptome profiling of genes involved in photosynthesis in Elaeagnus angustifolia L. under salt stress. Photosynthetica. 2018;56(4):998–1009. doi:10.1007/s11099-018-0824-6.
- Zhang ZQ. Determination of the soluble sugars in plant materials. In: Shanghai Society of plant physiology experimental handbook for plant physiology. Shanghai: Shanghai Science and Technology Press; 1985. p. 134–138.
- Bradford MM. A rapid and sensitive method for the quantitation of microgram quantities of protein utilizing the principle of protein-dye binding. Anal Biochem. 1976;72:248–254. doi:10.1016/0003-2697(76)90527-3.
- Jones RL, Varner JE. The bioassayof gibberellins. Planta. 1967;72:155–161. doi:10.2307/23366568.
- Xu Y, Liu R, Sui N, Shi WW, Wang L, Tian CY, Song J. Changes in endogenous hormones and seed-coat phenolics during seed storage of two Suaeda salsa populations. Aust J Bot. 2016;64:325–332. doi:10.1071/bt16014.
- Zhou JC, Fu TT, Sui N, Gou JR, Feng G, Fan JL, Song J. The role of salinity in seed maturation of the euhalophyte Suaeda salsa. Plant Biosyst. 2016;150:83–90. doi:10.1080/11263504.2014.976294.
- Guo JR, Li YD, Han G, Song J, Wang BS. NaCl markedly improved the reproductive capacity of the euhalophyte Suaeda salsa. Funct Plant Biol. 2018;45:350–361. doi:10.1071/FP17181.
- Liu YY, Wang H, Yu FM. Effects of salt stress on seed germination of Limonium bicolor. Chin J Ecol. 2009;28(9):1794–1800. In Chinese
- Bouallègue SF, Nouairi I. Salicylic acid and hydrogen peroxide pretreatments alleviate salt stress in faba bean (Vicia faba) seeds during germination. Seed Sci & Technol. 2017:45. doi:10.15258/sst.2017.45.3.07.
- Balibrea ME, Dell’Amico J, Bolarín MC, Pérez-Alfocea F. Carbon partitioning and sucrose metabolism in tomato plants growing under salinity. Physiol Plant. 2000;110:503–511. doi:10.1111/j.1399-3054.2000.1100412.x.
- Khodary SEA. Effect of salicylic acid on the growth, photosynthesis and carbohydrate metabolism in salt-stressed maize plants. Int J Agri Biol. 2004;6:5–8. http://www.ijab.org/1560–8530/2004/06–1–5–8.
- Singh B, Usha K. Salicylic acid induced physiological and biochemical changes in wheat seedlings under water stress. Plant Growth Regul. 2003;39(2):137–141. doi:10.1023/A:1022556103536.
- Sun TP. Gibberellin metabolism, perception and signaling pathways in Arabidopsis. Plant Biol. 2008;6:103–131. doi:10.1199/tab.0103.
- Yamaguchi S. Gibberellin metabolism and its regulation. Annu Rev Plant Biol. 2001;59:225–251. doi:10.1007/s003440010039.
- Ogawa M, Hanada A, Yamauchi Y. Gibberellin biosynthesis and response during arabidopsis seed germination. Plant Cell. 2003;15(7):1591–1604. doi:10.1105/tpc.011650.ble.
- Qin XQ, Zeevaart JAD. Overexpression of a 9-cis-epoxycarotenoid dioxygenase gene in Nicotiana plumbaginifolia increases abscisic acid and phaseic acid levels and enhances drought tolerance. Plant Physiol. 2002;128:544–551. doi:10.1104/pp.010663.