ABSTRACT
Extracellular ATP is perceived by the purinoceptor P2K1, leading to induction of defense response in plants. Previously, we described the transcriptomic response to extracellular ATP in wild-type Arabidopsis seedlings and mutants of classical defense hormone signaling pathways (Jewell et al., 2019, Plant Physiol. 179: 1144–58), in which extracellular ATP was found to induce defense-related genes independently and also along with other defense signaling pathways. In the present study, we provide further analysis and discussion of the data that we neglected to describe in the previous transcriptomics report. Briefly, we describe transcriptomic differences between a P2K1 knockout mutant (dorn1) and wild-type seedlings in the absence of exogenous ATP as well as an analysis of genes more responsive to extracellular ATP in a P2K1 overexpression line. Finally, we describe an exaggerated response to extracellular ATP in the ein2 mutant and suggest testable explanations of this phenomenon.
Introduction
All life on earth uses adenosine-5-triphosphate (ATP) inside the cell as an energy currency and genetic constituent, while many organisms perceive ATP outside the cell as a danger signal,Citation1 also called a damage-associated molecular pattern (DAMP). In Arabidopsis thaliana, extracellular ATP as a DAMP is monitored by the L-type lectin receptor kinase P2K1, also known as DORN1.Citation2
Effective plant defense against biotrophic or necrotrophic pathogens typically relies on salicylate- or jasmonate/ethylene-based signaling, respectively,Citation3 while extracellular ATP signaling via the purinoceptor P2K1 have been reported to contribute to plant defense against pathogens of biotrophic and necrotrophic lifestyles.Citation4–Citation8 These findings led us to question how plant purinergic signaling interacts with the signaling pathways of jasmonate, ethylene, and salicylate.
In our recent publication,Citation9 we analyzed ATP-responsive transcriptomes in wild-type (WT) seedlings and mutant seedlings defective in either jasmonate, ethylene, or salicylate signaling. We also examined the transcriptomes of the P2K1 knockout mutant (dorn1-3) and a P2K1 overexpression line (OxP2K1). Our principal conclusions from this study were that (1) the ATP-responsive transcriptome fully depends on the functional receptor P2K1, (2) overexpression of P2K1 results in exaggerated transcriptomic response to ATP, (3) many ATP-responsive genes depend on one or more of the jasmonate, ethylene, or salicylate signaling pathways, (4) nearly 30% of the ATP-responsive transcriptome depends on jasmonate signaling, (5) almost half of the ATP-responsive transcriptome depend on neither jasmonate, ethylene, nor salicylate signaling (we call it "putative ATP-specific" pathway), and (6) CAMTA3 and the MYC transcription factors play an important role for downstream steps of P2K1-mediated signaling for ATP-responsive gene expression.
In this article, we further analysed the ATP-induced transcriptomes described in Jewell et al.Citation9 (BioProject accession no. PRJNA494862). We hope these new results described below provide additional insights about extracellular ATP signaling.
Differentially expressed genes in the dorn1 mutant
To examine if any genes were misregulated in the dorn1-3 mutant in the absence of exogenous stimulus, we compared gene expression between mock-treated mutant and WT seedlings with a cutoff at a false-discovery rate (FDR) threshold of 0.05 and an absolute fold-change threshold of 1.5. We identified only 21 genes differently expressed between dorn1-3 and WT (). This small number of differentially expressed genes could indicate that P2K1-mediated extracellular ATP signaling is not a major player in plant growth and development under non-stressed conditions (at least during the growth stage we tested). Of these 21 genes, eight genes were differentially regulated (FDR < 0.05) after ATP treatment in the ATP hyper-responsive P2K1 overexpression line, and none were significantly responsive to ATP in WT seedlings ().
Figure 1. Heatmap of genes differentially expressed between dorn1-3 and WT without addition of exogenous ATP (mock), and the response of these genes to extracellular ATP in OxP2K1 and WT. An asterisk indicates that the gene expression was significantly different in the given comparison (FDR < 0.05). Heatmap was drawn with Morpheus (https://software.broadinstitute.org/morpheus).
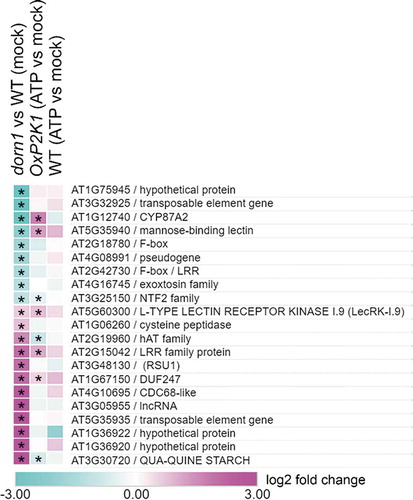
Notably, the gene encoding the extracellular ATP receptor P2K1 (AT5G60300) is marginally upregulated in all three comparisons: fold changes/FDR = 1.54/0.045 in dorn1 vs WT, 1.76/3.8e-9 in OxP2K1 (ATP vs mock), and 1.35/0.09 in WT (ATP vs mock) (). ATP-induced upregulation of P2K1 in WT and OxP2K1 is likely due to feedforward regulation by extracellular ATP signaling. However, it is surprising that the gene would be detected as upregulated in the dorn1-3 T-DNA insertion knockout mutant, which exhibits undetectable cytosolic calcium elevation and ATP-responsive gene expression upon exogenous ATP application.Citation2,Citation9 To explore this result further, we took a closer look at all reads of RNA-seq data mapped to AT5G60300 (the P2K1 locus) obtained from the dorn1-3 mutant and compared them to reads from WT. As shown in , there are clearly more reads mapped to AT5G60300 in the dorn1-3 mutant, particularly at the 5ʹ end of the gene. However, there are no reads obtained in the genomic span of 24,265,042 to 24,265,155, consistent with the annotation for the left border of the causative T-DNA insertion at 24,265,135 and indicating that the dorn1-3 mutant lacks full-length transcript. The upregulation of the 5ʹ end of the gene may be explained by feedback induction caused by loss of P2K1 function, or perhaps more likely by the presence of a cryptic promoter in the T-DNA. This truncated product could potentially cause a dominant negative effect on the phenotype in dorn1-3. Indeed, dorn1-1 and dorn1-2 have point mutations in the kinase domain and appeared to be semi-dominant loss-of-function mutations given that heterozygous mutant plants displayed intermediate phenotype between homozygous mutants and WT (see a supplementary figure in Choi et al.Citation2).
Figure 2. Plot of RNA-Seq reads mapped to the P2K1 locus in WT and the dorn1-3 mutant. The T-DNA insertion site is indicated, as well as the domain structure of the encoded protein. Both genotypes are displayed with the same scale (0–183 reads). The scale bar indicates nucleotide position on chromosome 5. Sashimi plot displayed with Integrative Genomics Viewer.Citation10.

Genes more responsive to ATP in OxP2K1
As shown in of Jewell et al.,Citation9 329 genes are greater than 2-fold more responsive to ATP in the P2K1-overexpressing line in comparison to WT, indicating that these genes are faithfully regulated by extracellular ATP in proportion to the amount of the purinoceptor P2K1. We were curious whether this subset of ATP-responsive genes displayed any peculiar characteristics with regard to dependency on the classic defense hormone signaling pathways. As shown in , the overall distribution of expression dependencies is similar between the subset of the over-responsive genes in OxP2K1 (329 genes) and the whole ATP transcriptome in WT (543 genes), with similar, though lesser, proportions of genes depending on COI1-, EIN2-, and NPR1-based signaling. One notable exception is that genes for the putative ATP-specific pathway are more enriched in the pool of 329 genes (42.9% versus 53.5%, P = 2.4e-10, hypergeometric distribution).
Figure 3. Overlap between genes responsive to ATP in ein2, but not WT, coi1, and npr1 (ein2-specific), and those responsive to ATP in OxP2K1.
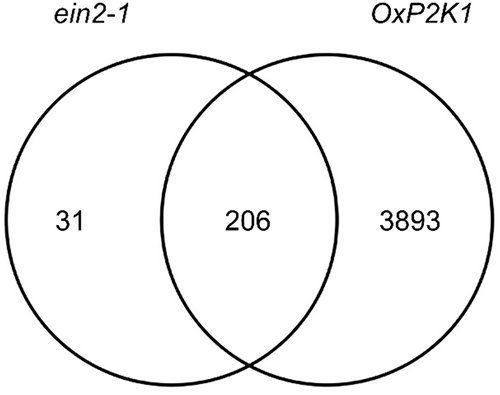
Table 1. Pathway dependencies of genes over-responsive to extracellular ATP in OxP2K1 and those responsive in WT.
Genes responsive to ATP in ein2, but not in WT, coi1, and npr1
Conspicuously, more genes were responsive to ATP in the ein2 mutant than in WT, coi1, or npr1 (797 versus 543, 356, and 572, respectively). Of these, 237 were uniquely responsive to ATP in this mutant, but not in WT or the other mutants while only 30 and 74 were uniquely responsive to ATP in coi1 and npr1, respectively (see Figure 4A in Jewell et al., 2019). This large disparity of ATP-responsive transcriptome in ein2 led us to examine these 237 genes in more detail. Similar to the other genotypes examined, these 237 genes were mostly induced (89%), rather than repressed in response to ATP. These ein2-unique genes were enriched in similar gene ontology terms (GO terms) as observed for WT (), while no gene ontology terms were enriched in coi1- and npr1-unique genes. Interestingly, 206 (87%) of these genes are also ATP-responsive in the ATP-hypersensitive OxP2K1 seedlings (). These results together lead us to speculate that EIN2-dependent ethylene signaling represses the ATP response of a subset of genes, and ATP hypersensitivity in the OxP2K1 line is sufficient to overcome this effect.
Table 2. GO term enrichment for 237 genes uniquely responsive to extracellular ATP in the ein2 mutant.
This effect could be explained in several non-mutually exclusive ways. For example, the P2K1 gene is mildly overexpressed in the ein2 mutant relative to WT (1.4-fold, FDR = 0.02), and as already mentioned, overexpressing P2K1 (in OxP2K1) increases the overall transcriptional response to extracellular ATP, with 7.5-fold more genes responding to extracellular ATP in this line than in WT ().
Additionally, ethylene signaling through EIN2 could have a negative effect on extracellular ATP signaling. Three positive regulators have been identified in the extracellular ATP signaling pathway: the reactive oxygen species-(ROS) producing enzyme RBOHD,Citation6 calmodulin binding transcription activator CAMTA3, and jasmonate signaling through COI1 and the MYC transcription factors.Citation8,Citation9,Citation11 Although RBOHD transcript levels were not significantly changed in the ein2 mutant,Citation9,Citation12 at least one ERF transcription factor has been reported to transcriptionally activate RBOHD.Citation13 In addition, ethylene signaling was reported to inhibit ROS burst via flavonol accumulation in guard cells.Citation14 Therefore, it seems that EIN2-mediated ethylene signaling could repress extracellular ATP response by attenuating ROS production (maybe through inhibition of RBOHD). Given a mutual interaction between calcium and ROS signaling,Citation15 it may also be possible that extracellular ATP-induced calcium signaling is perturbed by ethylene-mediated signaling pathway via attenuation of ROS production. Interestingly, aminocyclopropane-1-carboxylic acid, the direct precursor of ethylene, is reported to weaken the cytosolic calcium response induced by extracellular ATP.Citation16 With regard to CAMTA3, this class of transcription factors is strongly induced in response to ethylene treatment,Citation17 and we cannot detect a difference in CAMTA3 gene expression between ein2 and WT in our dataset (Fold change = 0.98, FDR = 0.93), so CAMTA3 does not seem to be negatively regulated by EIN2, at least transcriptionally. Though ethylene and jasmonate signaling are usually considered as working cooperatively, and mechanistic details of this have been described,Citation18,Citation19 this cooperativity does not always apply.Citation20 Therefore, a scheme in which ethylene signaling inhibits extracellular ATP response through a repressive role on jasmonate signaling is not entirely out of the question. This idea is supported by a recent report in which the ethylene-stabilized transcription factor EIN3 interacts with MYC2 to inhibit jasmonate-induced gene expression upon wound and herbivory.Citation21 Finally, in our dataset, there is higher expression of both MYC2 (fold change = 2.3, FDR = 1.1e-9) and MYC4 (fold change = 1.4, FDR = 9.9e-3) in ein2 relative to WT. This upregulation could lead to the greater transcriptional response we detected in ein2.
One could further speculate that unknown transcriptional repressors are constitutively downregulated in the ein2 mutant. A subset of ethylene response transcription factors, ERFs, are demonstrated to repress transcription by their ERF-associated amphiphilic repression (EAR) domains.Citation22 Of note, in our transcriptome data, a number of ERF transcription factors, including 2 EAR-containing ERFs, are underexpressed in ein2 relative to WT. In addition, six ERF genes were found to respond to ATP in WT, while 12 responded in ein2. While the GCC box, bound by ERF transcription factors, is marginally depleted in ATP-responsive genes in WT,Citation9 the GCC box is significantly enriched in ein2-unique genes (1.4-fold enriched, 11% of these promoters versus 8% across the Arabidopsis genome, P = 0.02). Thus, EAR-containing ERFs or another class of transcriptional repressors could fine tune the extracellular ATP-responsive transcriptome and a lack of ethylene signaling in ein2 or excessive extracellular ATP signaling in OxP2K1 is sufficient to abrogate this calibrating influence.
Conclusions and future perspective
Although our recent transcriptomics highlighted several aspects of extracellular ATP signaling, these undoubtedly present just a small fraction of the entire picture. Given that P2K is a unique purinoceptor,Citation23 structurally unlike animal P2X and P2Y purinoceptors, mechanisms of purinergic signaling in plants are expected to be completely dissimilar to those in animals.Citation24 In this case, a reverse genetics approach may be unlikely to be effective, and even any singular tactic, i.e., transcriptomics would limit our understanding. Thus, we need to integrate diverse omics approaches (e.g., epigenomics, proteomics, metabolomics, lipidomics, etc), with traditional tacks such as structure-function studies, forward genetics, and searching for P2K1-interacting proteins. These studies would likely each contribute complementary and synergistic information, and might provide the opportunity to unveil a more complete picture of mechanisms of purinergic signaling in plants.
Disclosure of potential conflicts of interest
No potential conflicts of interest were disclosed.
Acknowledgments
We are grateful to Dr. Natalia Moroz at Washington State University for critical comments on the manuscript, and to Dr. Nathan Havko at Michigan State University for suggesting Moriae Encomium. PPNS No. 0776, Department of Plant Pathology, College of Agriculture, Human and Natural Resource Sciences, Agricultural Research Center, Washington State University, Pullman, WA, 99164-6430, USA.
Additional information
Funding
References
- Verkhratsky A, Burnstock G. Biology of purinergic signalling: its ancient evolutionary roots, its omnipresence and its multiple functional significance. Bioessays. 2014;36:697–705. doi:10.1002/bies.201400024.
- Choi J, Tanaka K, Cao Y, Qi Y, Qiu J, Liang Y, Lee SY, Stacey G. Identification of a plant receptor for extracellular ATP. Science. 2014;343:290–294. doi:10.1126/science.343.6168.290.
- Glazebrook J. Contrasting mechanisms of defense against biotrophic and necrotrophic pathogens. Annu Rev Phytopathol. 2005;43:205–227. doi:10.1146/annurev.phyto.43.040204.135923.
- Chivasa S, Murphy AM, Hamilton JM, Lindsey K, Carr JP, Slabas AR. Extracellular ATP is a regulator of pathogen defence in plants. Plant J. 2009;60:436–448. doi:10.1111/j.1365-313X.2009.03968.x.
- Bouwmeester K, De Sain M, Weide R, Gouget A, Klamer S, Canut H, Govers F, Howlett BJ. The lectin receptor kinase LecRK-I.9 is a novel Phytophthora resistance component and a potential host target for a RXLR effector. PLoS Pathog. 2011;7:e1001327. doi:10.1371/journal.ppat.1001327.
- Chen D, Cao Y, Li H, Kim D, Ahsan N, Thelen J, Stacey G. Extracellular ATP elicits DORN1-mediated RBOHD phosphorylation to regulate stomatal aperture. Nat Commun. 2017;8:2265. doi:10.1038/s41467-017-02340-3.
- Balagué C, Gouget A, Bouchez O, Souriac C, Haget N, Boutet-Mercey S, Govers F, Roby D, Canut H. The arabidopsis thaliana lectin receptor kinase LecRK-I.9 is required for full resistance to pseudomonas syringae and affects jasmonate signalling. Mol Plant Pathol. 2017;18:937–948. doi:10.1111/mpp.12457.
- Tripathi D, Zhang T, Koo AJ, Stacey G, Tanaka K. Extracellular ATP acts on jasmonate signaling to reinforce plant defense. Plant Physiol. 2018;176:511–523. doi:10.1104/pp.17.01477.
- Jewell JB, Sowders JM, He R, Willis MA, Gang DR, Tanaka K. Extracellular ATP shapes a defense-related transcriptome both independently and along with other defense signaling pathways. Plant Physiol. 2019;179:1144–1158. doi:10.1104/pp.18.01301.
- Robinson JT, Thorvaldsdóttir H, Winckler W, Guttman M, Lander ES, Getz G, Mesirov JP. Integrative genomics viewer. Nat Biotechnol. 2011;29:24. doi:10.1038/nbt.1867.
- Tripathi D, Tanaka K. A crosstalk between extracellular ATP and jasmonate signaling pathways for plant defense. Plant Signal Behav. 2018;13:e1432229. doi:10.1080/15592324.2018.1432229.
- Mersmann S, Bourdais G, Rietz S, Robatzek S. Ethylene signaling regulates accumulation of the FLS2 receptor and is required for the oxidative burst contributing to plant immunity. Plant Physiol. 2010;154:391–400. doi:10.1104/pp.110.154567.
- Yao Y, He RJ, Xie QL, Zhao XH, Deng XM, He JB, Song L, He J, Marchant A, Chen X-Y, et al. ETHYLENE RESPONSE FACTOR 74 (ERF74) plays an essential role in controlling a respiratory burst oxidase homolog D (RbohD)-dependent mechanism in response to different stresses in Arabidopsis. New Phytol. 2017;213:1667–1681. doi:10.1111/nph.14278.
- Watkins JM, Hechler PJ, Muday GK. Ethylene-induced flavonol accumulation in guard cells suppresses reactive oxygen species and moderates stomatal aperture. Plant Physiol. 2014;164:1707–1717. doi:10.1104/pp.113.233528.
- Marcec MJ, Gilroy S, Poovaiah BW, Tanaka K. Mutual interplay of Ca(2+) and ROS signaling in plant immune response. Plant Sci. 2019;283:343–354. doi:10.1016/j.plantsci.2019.03.004.
- Waadt R, Krebs M, Kudla J, Schumacher K. Multiparameter imaging of calcium and abscisic acid and high-resolution quantitative calcium measurements using R-GECO1-mTurquoise in Arabidopsis. New Phytologist. 2017;216:303–320. doi:10.1111/nph.14706.
- Yang T, Poovaiah BW. A calmodulin-binding/CGCG box DNA-binding protein family involved in multiple signaling pathways in plants. J Biol Chem. 2002;277:45049–45058. doi:10.1074/jbc.M207941200.
- Lorenzo O, Piqueras R, Sanchez-Serrano JJ, Solano R. ETHYLENE RESPONSE FACTOR1 integrates signals from ethylene and jasmonate pathways in plant defense. Plant Cell. 2003;15:165–178. doi:10.1105/tpc.007468.
- Zhu Z, An F, Feng Y, Li P, Xue L, A M, Jiang Z, Kim J-M, To TK, Li W, et al.. Derepression of ethylene-stabilized transcription factors (EIN3/EIL1) mediates jasmonate and ethylene signaling synergy in Arabidopsis. Proc Natl Acad Sci U S A. 2011;108: 12539–44. doi:10.1073/pnas.1103959108.
- Kazan K. Diverse roles of jasmonates and ethylene in abiotic stress tolerance. Trends Plant Sci. 2015;20:219–229. doi:10.1016/j.tplants.2015.02.001.
- Song S, Huang H, Gao H, Wang J, Wu D, Liu X, Yang S, Zhai Q, Li C, Qi T, et al.. Interaction between MYC2 and ETHYLENE INSENSITIVE3 modulates antagonism between jasmonate and ethylene signaling in Arabidopsis. Plant Cell. 2014;26:263–279. doi:10.1105/tpc.113.120394.
- Ohta M, Matsui K, Hiratsu K, Shinshi H, Ohme-Takagi M. Repression domains of class II ERF transcriptional repressors share an essential motif for active repression. Plant Cell. 2001;13:1959–1968. doi:10.1105/tpc.010127.
- Choi J, Tanaka K, Liang Y, Cao Y, Lee SY, Stacey G. Extracellular ATP, a danger signal, is recognized by DORN1 in Arabidopsis. Biochem J. 2014;463:429–437. doi:10.1042/BJ20130619.
- Tanaka K, Choi J, Cao Y, Stacey G. Extracellular ATP acts as a damage-associated molecular pattern (DAMP) signal in plants. Front Plant Sci. 2014;5:446. doi:10.3389/fpls.2014.00446.