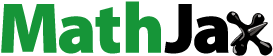
ABSTRACT
Melatonin involves in improving tolerance to abiotic and biotic stresses by regulating various biological processes. However, little is known about the underlying mechanism. Here, we investigated the effects of exogenous melatonin on seed germination in the halophyte Limonium bicolor under salt stress. Specifically, we examined the effect of salt stress on seed germination, melatonin concentration, and changes in the concentrations of nutrients, amylase activity, and hormones in L. bicolor seeds with and without pre-treatment with melatonin. Seed germination was significantly suppressed under a 200 mM NaCl treatment, but pre-treatment with melatonin significantly improved seed germination under salt stress. During seed germination, seeds pre-treated with melatonin contained high levels of melatonin and gibberellic acid (GA), low levels of abscisic acid (ABA), and high levels of amylase and alpha-amylase activity. Melatonin treatment upregulated the expression of key genes involved in GA biosynthesis (GA20ox and GA3ox), downregulated key genes involved in ABA biosynthesis (LbNCED1 and LbNCED3), and upregulated ABA 8ʹ-hydroxylase genes (LbCYP707A1 and LbCYP707A2), which mediate the changes in GA and ABA levels in seeds during germination. A high melatonin concentration in seeds promotes the utilization of nutrients and the synthesis of new proteins to enhance seed germination.
Introduction
Seed germination is a critical stage of the plant’s lifecycle in which static seeds are converted into highly active, growing seedlings.Citation1,Citation2 Seed germination begins with water uptake by macromolecular substances in seeds and is attenuated by embryo and endosperm expansion, which terminate in two successive stages: seed coat and endosperm rupture.Citation3,Citation4 Due to their sessile nature, most plant species remain at the same place throughout their lifecycles, where their seeds germinate. Therefore, endogenous and environmental cues must precisely mediate the timing of seed germination to ensure the reproduction of offspring.Citation5
Germination is a highly dynamic process in which macromolecules are re-synthesized to build new structures and are converted into micromolecules. Therefore, the germination process involves the activation of enzymatic pathways (e.g., amylases and proteases), energy acquisition, the building of new structures (e.g., via the phenylpropanoid pathway and laccase), and the metabolism of functional compounds such as hormones and regulators.Citation6 Many hypotheses about the regulation of seed dormancy and germination have been proposed and rejected,Citation7 and the regulatory mechanisms underlying seed dormancy and germination-induced changes remain unclear. Most of the proposed mechanisms involve certain plant hormones, especially abscisic acid (ABA) and gibberellic acid (GA). ABA functions as a positive regulator that induces and maintains seed dormancy and as a negative regulator of seed germination. GA positively regulates seed germination and can release dormancy. ABA and GA interact to regulate seed germination.Citation1,Citation6
Salinity (mainly referring to Na+) is a major abiotic stress in plants.Citation8–Citation10 Most plant species are sensitive to salt stress during seed germination and early seedling establishment.Citation11 High Na+ concentrations in saline soils inhibit seed germination, mainly because salt reduces the soil water potential, which impairs the ability of seeds to absorb water from the soil, thus suppressing seed imbibition and embryo growth.Citation1,Citation12,Citation13 Moreover, high Na+ concentrations appear to inhibit seed germination by restricting the availability of energy and metabolites. Though halophytes can complete their life cycle under above 200 mM NaCl, the germination was also affected under salinity.Citation14
Increasing evidence indicates that melatonin (N-acetyl-5-methoxytryptamine) plays vital roles in plant growth and development by improving tolerance to abiotic and biotic stresses by regulating various biological processes.Citation15–Citation21 The endogenous melatonin content of plants differs among species and can be modulated through genetic transformation.Citation22,Citation23 Melatonin application to the seeds of various plant species (Brassica oleracea, Cucumis sativus, Zea mays, and Vigna radiata) promoted seed germination and seedling development under environmental tress.Citation24–Citation27
To date, much of the work examining the roles of melatonin in plants has focused on its physiological effects on plant growth and development, and little is known about its influence on seed germination mechanisms. Limonium bicolor, a salt-secreting halophyte, discharges excess salt from the plant body through salt glands located on the leaf blades. L. bicolor has a high medicinal value and can improve salinity due to its salt tolerance. However, its seed germination is sensitive to salt and increasing its seed germination rate is very important for improving the use of saline-alkali land. In this study, we exogenously treated the seeds of L. bicolor with melatonin under salt stress and observed their germination and associated physiological parameters. The results of this study improve our understanding about the mechanism by which melatonin enhances plant tolerance to salt stress.
Materials and methods
Plant materials
All experiments were performed in a greenhouse at Shandong Normal University, Jinan (36°67΄N, 116°98΄E), China. The Limonium bicolor seeds were collected in Dongying city, Shandong Province (37°43΄N, 118°67΄E), China. The seeds were soaked in 0, 1, 50, 100, 200, 300, or 500 µM melatonin for 12 hours and dried to their initial weight.
Seed germination
Sterilized seeds were plated on sterilized filter paper in 9 cm × 9 cm Petri dishes and incubated at 22°C under a 16:8 h light:dark photoperiod. The seeds were watered daily with 0, 100 mM, or 200 mM NaCl dissolved in 1/5 strength Hoagland nutrient solution. Germination was quantified based on radicle emergence from three replicate experiments, with 100 seeds per replicate.
The calculation formula for each indicator is as follows:
In the formula, Gt is the number of seeds germinated at a given time (Dt) measured in days after the start of the germination test.
Determination of Na+ and Cl− concentrations
0.1 grams of seeds (seed coats or embryo) were crushed in 1.5 mL of distilled water. And then were boiled in distilled water (10 mL) for 3 h, and then supplemented evaporated water to 10 mL and the solution was filtered. The concentrations of Na+ and Cl− in the filtrate were determined with the Dionex ICS-1100 Ion Chroma-tography System (Dionex Corp., Sunnyvale, CA, USA).
Extraction and analysis of sugars
To determine sugar levels in L. bicolor seeds, 15 mg (FW) seed samples were homogenized in 2 mL of 80% (v/v) ethanol solution with a mortar and pestle. And then add 80% (v/v) ethanol solution until the volume is 10 ml. The homogenate was heated in a water bath at 75°C for 10 min and centrifuged at 5,000 g for 10 min to remove the insoluble residue. The supernatants (diluted with 80% ethanol to 10 ml) were further processed prior to soluble sugar measurements according to Liu.Citation28
Preparation of protein extracts
Protein extraction for native PAGE was performed as described in the “Enzyme Extraction and Assay” section.Citation29 To increase the protein concentration in the extracts and for protein purification, the supernatants were concentrated on Amicon ULTRA-0.5 mL 10 kDa Ultracel-PL membrane–centrifugal filters (Millipore, UFC501096) at 20,000 g for 30 min at 10°C. Protein concentration in the extracts was determined using the Bradford (1976) method.
Amylase and α-amylase activity assays
Amylase activity was measured as described by Jacobsen and Varner (1967).Citation30 100 sterilized seeds were pre-incubated in various concentrations of melatonin at 36°C for 12 h. The seeds were placed on filter paper moistened with different concentrations of NaCl and were incubated for 12 h at 27°C. And then seeds were minced in 2 ml pre-chilled PBS (phosphate buffer saline) solution. A crude enzyme solution was prepared by clarifying the supernatant via centrifugation at 5000 g for 10 min. 1 mL aliquot of diluted supernatant was used in the amylase and α-amylase assays. Amylase and α-amylase activity analysis was carried out using a plant amylase and plant α-amylase Enzyme-linked Immunoassay Kit (double antibody sandwich method, Huding Biological Technology Co., Shanghai, China), respectively. The enzyme units were determined as described by Jacobsen and Varner (1967).
Extraction and determination of GA and ABA concentrations
Each seed sample (15 mg) was homogenized in 1 mL of distilled water and shaken at 4°C overnight prior to the determination of endogenous GA and ABA concentrations. The homogenate was centrifuged at 12,000 g for 10 minutes at 4°C, and the supernatant was aspirated and collected. GA and ABA analysis was carried out using a Plant Gibberellin (GA) and Plant Abscisic Acid (ABA) Enzyme-linked Immunoassay Kit (double antibody sandwich method, Huding Biological Technology Co., Shanghai, China), respectively.
Quantitative real-time PCR analysis
The RNA was extracted from the seeds treated with NaCl and melatonin for two days and reverse transcribed into cDNA. Genes for GA synthesis (GA20ox, GA3ox), ABA synthesis genes (LbNCED1, LbNCED3) and decomposition genes (LbCYP707A1, LbCYP707A2) were selected for qRT-PCR validation. Primers for these 6 genes were designed using Beacon Desiger (Supplementary table). The tubulin gene of the species was used as an internal standard. Real-time PCR was performed with Ace Universal AYBR qPCR Master Mix kit and on a real-time quantitative PCR instrument (Bio-Rad, California, USA). The 2-△△Ct method was used to calculate the relative expression of each gene.Citation31
Statistical analysis
The SPSS software package (IBM, SPSS statistics 19.0) was used in the statistical analyzes in the study. Analysis of variance (ANOVA) was performed for determination of the statistical significances. Duncan’s multiple range tests were carried out to identify significant differences between values at P < .05.
Results
The effects of salt stress and melatonin on L. bicolor seed germination
Salt stress inhibited the germination of L. bicolor seeds. As the salt concentration increased, the germination rate significantly decreased (). The germination rate, germination potential (), and germination index () of the seeds were significantly reduced under a 200 mM NaCl treatment. The germination ability of the seeds initially increased, followed by a decrease, in response to increasing melatonin concentration. Under a 0 mM NaCl (CK) treatment, the germination ability of L. bicolor seeds significantly increased in response to a 1 µM melatonin treatment, whereas under a 100 and 200 mM NaCl treatment, the germination ability of the seeds significantly increased in response to a 200 µM melatonin treatment. Melatonin concentrations ranging from 50–300 µM, especially 200 µM, significantly alleviated the inhibited germination rate (), germination potential (), and germination index () of L. bicolor seeds in response to salt treatment. We therefore used 200 µM melatonin treatment for subsequent experiments.
Figure 1. Germination of L. bicolor seeds after 7 days of treatment with various concentrations of melatonin (MT) (0 µM, 1 µM, 50 µM, 100 µM, 200 µM, 300 µM, and 500 µM) and NaCl (CK (0 mM), 100 mM, and 200 mM). A: germination rate; B: germination potential; C: germination index. Values are mean ± standard deviation of three biological replicates. Bars labeled with different letters are significantly different at P < .05 according to Duncan’s multiple range tests.
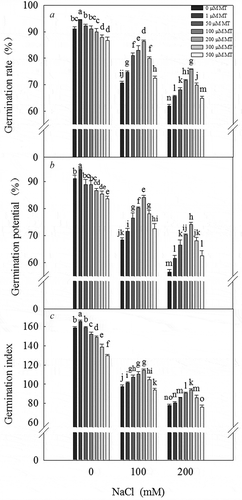
Melatonin concentration in L. bicolor seeds
The melatonin concentration significantly increased in response to a 200 mM NaCl treatment. When exogenous melatonin was applied to L. bicolor seeds under salt stress, the melatonin concentration significantly increased to ~1.5 times that observed in the 200 mM NaCl treatment (). Under a 0 mM NaCl (CK) treatment, exogenous melatonin did not increase the melatonin concentration in seeds, but under a 200 mM NaCl treatment, exogenous melatonin significantly increased the melatonin concentration in seeds.
Figure 2. Melatonin concentration of L. bicolor seeds after two days of treatment with different concentrations of melatonin (MT) (0 µM, 200 µM) under 0 and 200 mM NaCl conditions. Values are mean ± standard deviation of three biological replicates. Bars labeled with different letters are significantly different at P < .05 according to Duncan’s multiple range tests.
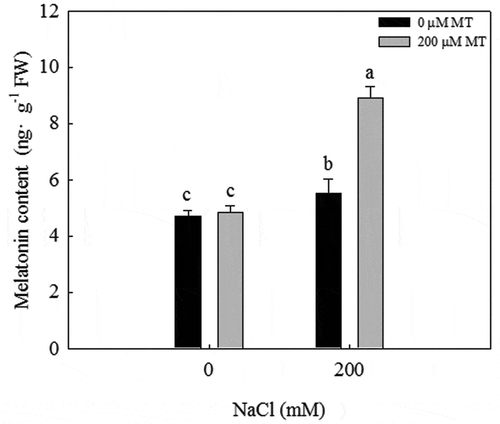
Germination rates of L. bicolor seeds under various treatments
Whereas 89% of the seeds germinated at 7 d of incubation under normal conditions (CK), only 62.2, 80, and 67.8% of the seeds germinated under 200 mM NaCl, 20 mM LiCl, and 400 mM mannitol treatment, respectively. Treatment with 200 µM melatonin significantly alleviated the inhibition of seed germination by NaCl and mannitol ().
Figure 3. Germination rates of L. bicolor seeds after 7 days of treatment with CK, 200 mM NaCl, 20 mM LiCl, and 400 mM mannitol after pre-treatment with 0 and 200 µM melatonin (MT). Values are mean ± standard deviation of three biological replicates. Bars labeled with different letters are significantly different at P < .05 according to Duncan’s multiple range tests.
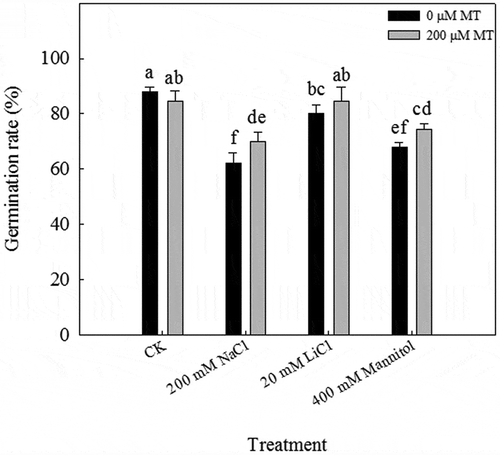
Effects of melatonin on Na+ and Cl – contents in seeds, seed coats, and embryos
The Na+ concentration in the seed (), seed coat (), and embryo () and the Cl – concentration in the seed () and seed coat () were significantly greater under a 200 mM NaCl treatment, and pretreatment with melatonin did not alter the concentrations of these ions in seeds, seed coats, or embryos. The increase in ion concentrations in the seeds was mainly due to the increase in ion concentrations in the seed coat.
Figure 4. Na+ and Cl – concentration in seeds, seed coats, and embryos of L. bicolor after 2 days of treatment with different concentrations of melatonin (MT) (0 µM, 200 µM) under 0 and 200 mM NaCl conditions. A: Na+ concentration in seeds; B: Cl – concentration in seeds; C: Na+ concentration in seed coats; D: Cl – concentration in seed coats; E: Na+ concentration in embryos; F: Cl – concentration in embryos. Values are mean ± standard deviation of three biological replicates. Bars labeled with different letters are significantly different at P < .05 according to Duncan’s multiple range tests.
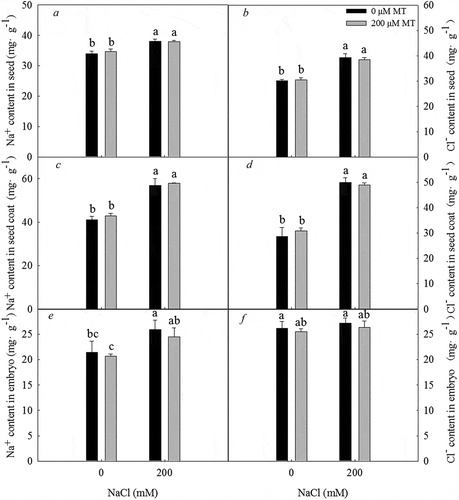
Effects of melatonin on soluble sugar and total protein concentrations
Treatment with 200 mM NaCl led to an increase in soluble sugar concentration and a decrease in protein concentration. Under a 200 µM melatonin treatment, a marked decrease in soluble sugar concentration and an increase in protein concentration were detected (P < .05) (). Therefore, melatonin treatment decreases the soluble sugar concentration and increases the protein concentration in L. bicolor seeds under salt stress.
Figure 5. Soluble sugar concentration and total protein concentration in L. bicolor seeds after 2 days of treatment with different concentrations of melatonin (MT) (0 µM, 200 µM) under 0 and 200 mM NaCl conditions. A: soluble sugar concentration; B: total protein concentration. Values are mean ± standard deviation of three biological replicates. Bars with different letters are significantly different at P < .05 according to Duncan’s multiple range tests.
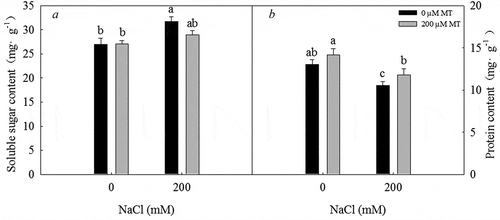
Effects of melatonin on amylase and α-amylase activity
Treatment with 200 mM NaCl significantly inhibited amylase and α-amylase activity in L. bicolor seeds. In contrast, the activities of these enzymes significantly increased in response to treatment with 200 µM melatonin under 200 mM NaCl. Thus, melatonin increases amylase () and α-amylase () activity in L. bicolor seeds under salt stress.
Figure 6. Amylase and α-amylase activity in L. bicolor seeds after 1 day of treatment with different concentrations of melatonin (MT) (0 µM, 200 µM) under 0 and 200 mM NaCl conditions. A: amylase activity; B: α-amylase activity. Values are mean ± standard deviation of three biological replicates. Bars with different letters are significantly different at P < .05 according to Duncan’s multiple range tests.
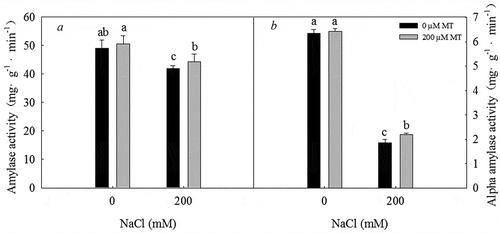
Effects of melatonin on gibberellic acid (GA) and abscisic acid (ABA) concentrations
200 mM NaCl treatment significantly decreased the GA concentration under 0 µM melatonin treatment in germinating seeds after two days. 200 µM melatonin treatment significantly increased the GA concentration in seeds (). In contrast, the ABA concentration in germinating seeds after two days significantly increased in response to treatment with 200 mM NaCl and significantly decreased in response to treatment with 200 µM melatonin (). Thus, melatonin appears to play a role in regulating GA and ABA concentrations during seed germination.
Figure 7. GA and ABA concentrations in L. bicolor seeds after 2 days of treatment with different concentrations of melatonin (MT) (0 µM, 200 µM) under 0 and 200 mM NaCl conditions. A: GA concentration; B: ABA concentration. Values are mean ± standard deviation of three biological replicates. Bars with different letters are significantly different at P < .05 according to Duncan’s multiple range tests.
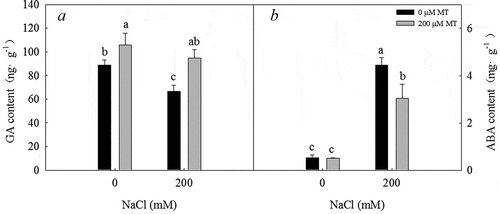
Effects of melatonin on the expression of GA and ABA biosynthesis and ABA hydroxylase genes
Both GA 20-oxidase (GA20ox) and GA 3-oxidase (GA3ox), which are involved in key steps of GA biosynthesis in seeds, were significantly downregulated in response to a 200 mM NaCl treatment, but significantly upregulated in response to 200 µM melatonin and 200 µM melatonin combined with 200 mM NaCl (). These effects contribute to the increase in GA content during the subsequent germination periods.
Figure 8. Effects of 200 µM melatonin on the transcript levels of key GA biosynthesis, ABA biosynthesis, and hydroxylase genes during L. bicolor seed germination under NaCl stress. Tubulin was used as an internal standard. Values are mean ± standard deviation of three biological replicates. Bars with different letters are significantly different at P < .05 according to Duncan’s multiple range tests.
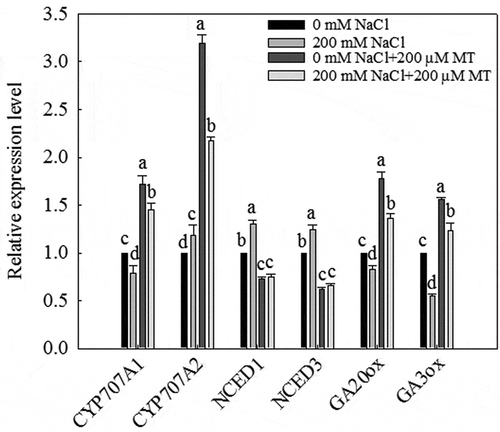
Both genes were significantly upregulated by a 200 mM NaCl treatment on the second day of seed germination, but significantly downregulated by treatment with 200 µM melatonin or 200 µM melatonin plus 200 mM NaCl (). These results indicate that melatonin reduces ABA biosynthesis under salt stress. The activity of the ABA 8ʹ-hydroxylases LbCYP707A1 and LbCYP707A2 causes a rapid decrease in ABA levels during seed germination. LbCYP707A1 was downregulated by the 200 mM NaCl treatment but was significantly upregulated by treatment with 200 µM melatonin or 200 µM melatonin plus 200 mM NaCl. Finally, LbCYP707A2 was significantly upregulated by treatment with 200 µM melatonin or 200 µM melatonin plus 200 mM NaCl.
Discussion
Exogenous melatonin significantly promoted the germination of L. bicolor seed under salt stress. Melatonin is considered to be a biostimulator, as it is a non-toxic substance of natural origin that can improve and stimulate plant health.Citation32,Citation33 Endogenous melatonin concentrations differ not only among plant species but also among varieties of the same species.Citation34 An analysis of endogenous melatonin contents in different plant organs and seeds revealed that the highest levels of this compound are found in reproductive organs, particularly seeds,Citation33,Citation35 which might be related to its role in the process of seed germination. In addition, increasing evidence suggests that changes in melatonin contents might result from various types of environmental stress.Citation36
Salt stress is a major abiotic stress.Citation8–Citation10 Both halophytes and non-halophytes are highly sensitive to salt stress during seed germination and early seedling establishment.Citation7,Citation10,Citation11 Seed germination is one of the most critical stages of the plant’s lifecycle and is greatly affected by salinity.Citation37 Many studies have shown that high salinity levels completely inhibit seed germination and that low levels induce seed dormancy.Citation38 In addition, salinity disrupts the hormonal balance in plantsCitation14,Citation39 and reduces the ability to use stored substances in seeds.Citation40 In the current study, we found that high salt stress impaired seed germination, decreased the GA concentration, increased the ABA concentration, and reduced the utilization of seed reserves in L. bicolor (, , and ). High salt levels have two major effects on plants: the induction of osmotic stress and ion toxicity.Citation14 The current results indicate that the low germination rate of L. bicolor seeds under salt treatment was mainly due to the osmotic effect of high salt levels ().
Melatonin promotes L. bicolor seed germination under salt stress (). The inhibitory effect of high salinity on L. bicolor seed germination was alleviated by the application of 200 µM melatonin, which is consistent with its previously reported effects on seed germination.Citation41 We found that the endogenous melatonin concentration in L. bicolor seeds increased under salt stress. Moreover, exogenous melatonin treatment significantly increased melatonin levels in seeds, while there were no changes in melatonin levels under control conditions (). Therefore, in this study, we demonstrated that exogenous melatonin increases the melatonin concentration in L. bicolor seeds and promotes seed germination under salt stress.Citation21
The high melatonin concentration in seeds upregulated the expression of key genes (GA20ox and GA3ox, ) involved in GA biosynthesis, Citation42 which led to an increase in GA concentration during the subsequent germination periods. Nine-cis-epoxycarotenoid dioxygenase (NCED) is a key regulatory enzyme involved in ABA biosynthesis.Citation43 The key genes responsible for ABA biosynthesis in L. bicolor, LbNCED1 and LbNCED3 were downregulated during seed germination in melatonin-pretreated seeds under salt stress. The transcriptional levels of the ABA 8ʹ-hydroxylase genes LbCYP707A1 and LbCYP707A2, which play major roles in the rapid decrease in ABA content during seed germination, Citation44 were upregulated during seed germination in melatonin-pretreated seeds under salt stress. It appears that the downregulated expression of LbNCED1 and LbNCED3 and the upregulated expression of LbCYP707A1 and LbCYP707A2 are responsible for the decrease in ABA content in these seeds.
The quantity and utilization of seed reserves directly affect early seedling growth.Citation7 Seed germination in L. bicolor is negatively affected by salinity stress, as observed in other plants.Citation45,Citation46 Seed germination involves two distinct metabolic processes: (1) enzymatic hydrolysis of seed storage proteins and (2) the formation of new cellular structures.Citation47,Citation48 The reduced germination rate of L. bicolor is due to reduced nutrient availability under salt stress (). Melatonin promotes soluble sugar utilization and the synthesis of new proteins, thereby increasing the germination rate of L. bicolor seeds under salt stress. However, the mechanism by which melatonin promotes nutrient utilization in seeds is not clear. Perhaps the increase in GA levels in seeds promotes the synthesis of hydrolases (especially α-amylase) (Peng and Harberd, 2002).Citation49 To explore how melatonin improves nutrient utilization during seed germination, we measured amylase activity in seeds. During seed germination, amylase activity rapidly decreased under NaCl stress compared to the control (). This reduction in amylase activity was less when plants were pretreated with melatonin, confirming that melatonin protects enzymes from high salinity-induced damage. Indeed, melatonin stimulates the secretion of pancreatic amylase and l-tryptophan (the precursor of melatonin) as well as enzyme secretion in rats.Citation50 However, the mechanism by which melatonin affects amylase activity in plants is unclear.
In this study, we showed that exogenous melatonin improves L. bicolor seed germination under salt stress. Melatonin promotes ABA catabolism and GA biosynthesis under salt stress conditions and regulates seed germination by promoting nutrient utilization and protein synthesis in seeds. This is the first study exploring the mechanism by which melatonin mitigates the inhibitory effects of salt stress on seed germination in halophytes. It may provide a new idea for MT modulation in the regulation of plant salt defenses. This study represents a step toward defining the role of melatonin in the response to adverse conditions in halophytes. Moreover, these results provide a theoretical basis for MT to alleviate salt stress in L. bicolor.
Authors’ Contribution Statement
Junpeng Li and Chen Zhao wrote this manuscript; Mingjing Zhang and Chen Zhao performed experiments; Junpeng Li and Mingjing Zhang collected data, carried out all analyses and were involved in preparing figures; Fang Yuan and Min Chen conceptualized the idea. All authors read and approved the final manuscript.
Conflict of Interest
The authors declare that they have no competing interests.
Supplemental Material
Download MS Word (16.5 KB)Supplementary material
Supplemental data for this article can be accessed on publisher’s website.
Additional information
Funding
References
- Rajjou L, Duval M, Gallardo K, Catusse J, Bally J, Job C, Job D. Seed germination and vigor. Annu Rev Plant Biol. 2012;63:507–533. doi:10.1146/annurev-arplant-042811-105550.
- Finch-Savage WE, Leubner-Metzger G. Seed dormancy and the control of germination. New Phytol. 2006;171:501–523. doi:10.1111/j.1469-8137.2006.01756.x.
- Afreen F, Zobayed AMS, Kozai T. Melatonin in Glycyrrhiza uralensis; response of plant root to spectral quality of light and UV-B radiation. J Pineal Res. 2006;41:108–115. doi:10.1111/j.1600-079X.2006.00352.x.
- Bewley JD. Seed germination and dormancy. Plant Cell. 1997;9:1055–1066. doi:10.1105/tpc.9.1.97.
- Finkelstein R, Reeves W, Ariizumi T, Steber C. Molecular aspects of seed dormancy. Annu Rev Plant Biol. 2008;59:387–415. doi:10.1146/annurev.arplant.59.032607.092740.
- Rosental L, Nonogaki H, Fait A. Activation and regulation of primary metabolism during seed germination. Seed Sci Res. 2014;24:1–15. doi:10.1017/S0960258513000391.
- Guo J, Li Y, Han G, Song J, Wang BS. NaCl markedly improved the reproductive capacity of the euhalophyte Suaeda salsa. Funct Plant Biol. 2018;45(3):350–361. doi:10.1071/FP17181.
- Feng Z-T, Deng Y-Q, Zhang S-C, Liang X, Yuan F, Hao J-L, Zhang J-C, Sun S-F, Wang B-S. K+ accumulation in the cytoplasm and nucleus of the salt gland cells of Limonium bicolor accompanies increased rates of salt secretion under NaCl treatment using NanoSIMS. Plant Sci. 2015;238:286–296. doi:10.1016/j.plantsci.2015.06.021.
- Han G, Wang M, Yuan F, Sui N, Song J, Wang B. The CCCH zinc finger protein gene AtZFP1 improves salt resistance in Arabidopsis thaliana. Plant Mol Biol. 2014;86:237–253. doi:10.1007/s11103-014-0226-5.
- Zhu JK. Plant salt tolerance. Trends Plant Sci. 2001;6:66–71. doi:10.1016/S1360-1385(00)01838-0.
- Cheng Y, Tian Q, Zhang WH. Glutamate receptors are involved in mitigating effects of amino acids on seed germination of Arabidopsis thaliana under salt stress. Environ Exp Bot. 2016;130:68–78. doi:10.1016/j.envexpbot.2016.05.004.
- Shao Q, Han N, Ding T, Zhou F, Wang B. SsHKT1;1 is a potassium transporter of the C3 halophyte Suaeda salsa that is involved in salt tolerance. Funct Plant Biol. 2014;41:790–802. doi:10.1071/FP13265.
- Daszkowska-Golec A. Arabidopsis seed germination under abiotic stress as a concert of action of phytohormones. OMICS. 2011;15:763–774. doi:10.1089/omi.2010.0113.
- Song J, Wang B. Using euhalophytes to understand salt tolerance and to develop saline agriculture: Suaeda salsa as a promising model. Ann Bot London. 2015;115:541–553. doi:10.1093/aob/mcu194.
- Zhan N, Sun Q, Zhang H, Cao Y, Weeda S, Ren S, Guo YD. Roles of melatonin in abiotic stress resistance in plants. J Exp Bot. 2015;66:647–656. doi:10.1093/jxb/eru336.
- Hardeland R. Melatonin in plants and other phototrophs: advances and gaps concerning the diversity of functions. J Exp Bot. 2015;66:627–646. doi:10.1093/jxb/eru386.
- Nawaz MA, Huang Y, Bie Z, Reiter RJ, Niu M, Hameed S. Melatonin: current status and future perspectives in plant science. Front Plant Sci. 2016;6:1230. doi:10.3389/fpls.2015.01230.
- Shi H, Chen Y, Tan DX, Reiter RJ, Chan Z, He C. Melatonin induces nitric oxide and the potential mechanisms relate to innate immunity against bacterial pathogen infection in Arabidopsis. J Pineal Res. 2015;59:102–108. doi:10.1111/jpi.12244.
- Arnao MB, Hernández-Ruiz J. The physiological function of melatonin in plants plant. Signal Behav. 2006;1:89–95. doi:10.4161/psb.1.3.2640.
- Park S, Lee DE, Jang H, Byeon Y, Kim YS, Back K. Melatoninrich transgenic rice plants exhibit resistance to herbicide-induced oxidative stress. J Pineal Res. 2013;54:258–263. doi:10.1111/j.1600-079X.2012.01029.x.
- Wei W, Li QT, Chu YN, Reiter RJ, Yu XM, Zhu DH, Zhang WK, Ma B, Lin Q, Zhang JS. Melatonin enhances plant growth and abiotic stress tolerance in soybean plants. J Exp Bot. 2014;66:695–707. doi:10.1093/jxb/eru457.
- Byeon Y, Back K. An increase in melatonin in transgenic rice causes pleiotropic phenotypes, including enhanced seedling growth, delayed flowering, and low grain yield. J Pineal Res. 2014;56:408–414. doi:10.1111/jpi.2014.56.issue-4.
- Okazaki M, Higuchi K, Aouini A, Ezura H. Lowering intercellular melatonin levels by transgenic analysis of indoleamine 2, 3-dioxygenase from rice in tomato plants. J Pineal Res. 2010;49:239–247. doi:10.1111/j.1600-079X.2010.00788.x.
- Kołodziejczyk I, Bałabusta M, Szewczyk R, Posmyk MM. The levels of melatonin and its metabolites in conditioned corn (Zea mays L.) and cucumber (Cucumis sativus L.) seeds during storage. Acta Physiol Plant. 2015;37:105–125. doi:10.1007/s11738-015-1850-7.
- Janas KM, Ciupinska E, Posmyk MM. Melatonin applied by hydropriming, as phytobiostimulator improving corn (Zea mays L.) seedlings growth at abiotic stresses conditions. In: Li S, Wang Y, Cao F, Huang P, Zhang Y, editors. Progress in Environmental Science and Technology Vol II A. Princeton (NJ): Science Press USA Inc.; 2009. p. 383–388.
- Szafranska K, Szewczyk R, Janas KM. Involvement of melatonin applied to Vigna radiata L. seeds in plant response to chilling stress. Central Europ J Biol. 2014;9:1117–1126.
- Posmyk MM, Bałabusta M, Wieczorek M, Sliwinska E, Janas KM. Melatonin applied to cucumber (Cucumis sativus L.) seeds improves germination during chilling stress. J Pineal Res. 2009a;46:214–223. doi:10.1111/j.1600-079X.2008.00652.x.
- Liu G. Comparison of different colorimetric methods for sulfonium sulfonate. Tianjin Agric Sci. 2016;22:5–7+22.
- Bradford MM. A rapid and sensitive method for the quantitation of microgram quantities of protein utilizing the principle of protein-dye binding. Anal Biochem. 1976:72:248–254. doi:10.1006/abio.1976.9999.
- Jones RL, Varner JE. The bioassay of gibberellins. Planta. 1967;72:155–161. doi:10.2307/23366568.
- Livak KJ, Schmittgen TD. Analysis of relative gene expression data using real-time quantitative PCR and the 2−ΔΔCT method. Methods. 2001;25:402–408. doi:10.1006/meth.2001.1262.
- Kołodziejczyk I, Posmyk MM. Melatonin - a new plant biostimulator. J Elementol. 2016;21:1187–1198.
- Janas KM, Posmyk MM. Melatonin, an underestimated natural substance with great potential for agricultural application. Acta Physiol Plant. 2013;35:3285–3292. doi:10.1007/s11738-013-1372-0.
- Hernández-Ruiz J,Arnao MB. Distribution of melatonin in different zones of lupin and barley plants at different ages in the presence and absence of light. J Agric Food Chem. 2008;56:10567–10573. doi:10.1021/jf8022063
- Posmyk MM, Janas KM, Kontek R. Red cabbage anthocyanin extract alleviates copper-induced cytological disturbances in plant meristematic tissue and human lymphocytes. Biometals. 2009b;22:479–490. doi:10.1007/s10534-009-9205-8.
- Zhang H 2016 Effects of melatonin treatment on cucumber seed germination under NaCl stress and its molecular mechanism [D]. China Agricultural University. doi:10.2116/analsci.32.1267.
- Misra N, Dwivedi UN. Genotypic difference in salinity tolerance of greengram cultivars. Plant Sci. 2004;166:1135–1142. doi:10.1016/j.plantsci.2003.11.028.
- Iqbal M, Ashraf M, Jamil A, Rehman S. Does seed priming induce changes in the levels of some endogenous plant hormones in hexaploid wheat plants under salt stress? JIPB. 2006;48:181–189.
- Khan MA, Rizvi Y. Effect of salinity, temperature and growth regulators on the germination and early seedling growth of Atriplex griffithii var, Stocksii. Can J Bot. 1994;72:475–479. doi:10.1139/b94-063.
- Ahmad J, Bano M. The effect of sodium chloride on physiology of cotyledons and mobilization of reserved food in Cicer arietinum. Pak J Bot. 1992;24:40–48.
- Zhang N, Zhao B, Zhang HJ, Weeda S, Yang C, Yang ZC, Ren S, Guo YD. Melatonin promotes water-stress tolerance, lateral root formation, and seed germination in cucumber (Cucumis sativus L.). J Pineal Res. 2013;54:15–23. doi:10.1111/j.1600-079X.2012.01015.x.
- Ogawa M, Hanada A, Yamauchi Y, Kuwahara A, Kamiya Y, Yamaguchi S. Gibberellin biosynthesis and response during Arabidopsis seed germination. Plant Cell. 2003;15:1591–1604. doi:10.1105/tpc.011650.
- Qin XQ, Zeevaart JAD. Overexpression of a 9-cis-epoxycarotenoid dioxygenase gene in Nicotiana plumbaginifolia increases abscisic acid and phaseic acid levels and enhances drought tolerance. Plant Physiol. 2002;128:544–551.
- Kushiro T, Okamoto M, Nakabayashi K, Yamagishi K, Kitamura S, Asami T, Hirai N, Koshiba T, Kamiya Y, Nambara E. The Arabidopsis cytochrome P450 CYP707A encodes ABA 8ʹ-hydroxylases: key enzymes in ABA catabolism. Embo J. 2004;23:1647–1656. doi:10.1038/sj.emboj.7600121.
- Hampson CR, Simpson GM. Effects of temperature, salt and osmotic pressure on early growth of wheat (Triticum aestivum). Can J Bot. 1990;68:524–528. doi:10.1139/b90-072.
- Ashraf M, McNeily T. Variability in salt tolerance of nine spring wheat cultivars. J Agron Crop Sci. 1988;160:14–21. doi:10.1111/j.1439-037X.1988.tb01160.x.
- Mayer AM, Poljakoff-Mayber A. The Germination of Seeds. fourth ed. Oxford: Pergamon Press; 1989.
- Bewley JD, Black M. Physiology and Biochemistry of Seeds in Relation to Germination. Vol. 2, Berlin: Springer-Verlag; 1982.
- Peng J, Harberd NP. The role of GA-mediated signalling in the control of seed germination. Curr Opin Plant Biol. 2002;5:376-381. 10.1016/S1369-5266(02)00279-0.
- Jaworek J. Ghrelin and melatonin in the regulation of pancreatic exocrine secretion and maintaining of integrity. J Physiol Pharmacol. 2006;57:83–96.