ABSTRACT
Glycine betaine (GB), an osmolyte, is produced in chloroplasts by the action of betaine aldehyde dehydrogenase (BADH) on its precursor betaine aldehyde. The present work highlights the significance of nitric oxide (NO) in GB homeostasis as a long-distance salt (120 mM NaCl) stress-elicited response. In light-grown seedling cotyledons, both the activity and transcript levels of BADH are much higher than in dark-grown seedlings irrespective of salt stress. Significantly high accumulation of GB in dark-grown seedling cotyledons indicates its preferential mobilization from cotyledons to other plant parts in light-grown seedlings. NO donor application (diethylenetriamine) maintains high BADH activity in light, although in dark it is brought down marginally. BADH levels are maintained high in light than in dark in respective treatments. Reversal of the effect of NO donor on age-dependent GB content, BADH activity, and transcript levels by NO scavenger (diethyldithiocarbamate) further demonstrates the impact of NO on GB homeostasis in light- and dark-grown seedlings in an age-dependent manner, major modulation being observed in 4-d-old seedlings. The present work, thus, provides new information on co-regulation of GB homeostasis by NO and light. It also puts forward new information of GB–NO crosstalk in maneuvering salt stress sensing as a long-distance response in seedlings.
Introduction
Plants sense and modify their metabolism and activate a variety of defense mechanisms during stress conditions in order to get acclimatized.Citation1,Citation2 Adjustment of osmotic balance, accumulation of reactive oxygen species (ROS), and activation of antioxidant enzymes are the primary and major biochemical events taking place in plants during stress conditions.Citation3,Citation4 Accumulation of “compatible solutes” confers stress tolerance to plants through regulation of cellular osmotic balance, detoxification of ROS, and maintenance of plasma membrane integrity.Citation5 Glycine betaine (GB) is one of the most cosmopolitan osmolytes in plants, animals, and bacteria. GB (N, N, N-trimethylglycine) is zwitterionic in nature, and its nonpolar hydrocarbon moiety enables it to easily communicate with all the domains of biomolecules, such as proteins and enzymes.Citation6,Citation7 Enhanced accumulation of GB in plants directly signifies the degree of stress tolerance in plants.Citation8–Citation10 Predominantly, GB synthesis occurs in chloroplasts through the action of enzymes such as choline monooxygenase (CMO) and betaine aldehyde dehydrogenase (BADH) on choline and betaine aldehyde (BA), respectively.Citation11 In some plants such as barley, however, CMO and BADH have also been reported to be localized in peroxisomes in addition to chloroplasts.Citation12,Citation13 GB uses multifaceted defense mechanisms to protect plants from the deleterious effect of various stressesCitation14–Citation23 (). It reduces the loss of K+ ions and maintains high K+/Na+ ratio in cells during salinity stress. GB maintains the equilibrium of K+ ions by suppressing Na+ uptake through apoplastic route and thus, alleviating stress.Citation24–Citation26 Based on already standardized concentrations of NaCl (120 mM), diethylenetriamine (DETA; 250 μM), and diethyldithiocarbamate (DETC; 250 μM) in the author’s laboratory,Citation27 the present work focuses on examining photomodulation of GB homeostasis,Citation28,Citation29 as a long-distance signaling response to salt stress in sunflower seedling cotyledons. Modulation of BADH activityCitation30,Citation31 and transcriptCitation31 levels by nitric oxide (NO) has been proposed to be differentially regulated in light- and dark-grown seedlings, thereby altering GB homeostasis. Furthermore, GB's interaction with NO has been demonstrated to highlight its role as cross-talking molecule.
Results
Tissue sensitivity (age), growth conditions (light or dark), and NO modulate GB homeostasis in response to salt stress
Accumulation of GB in dark-grown seedling cotyledons of sunflower (Helianthus annuus L.) varied from 9.45 μg.mg−1 Fresh weight (FW) (in salt-stressed 6-d-old seedling) to 35.61 μg.mg−1 FW (in salt-stressed 4-d-old seedling). Maximum GB accumulation occurred in 4-d-old NaCl-treated (120 mM NaCl) seedling cotyledons. NaCl treatment resulted in enhanced GB content in cotyledons of 2- and 4-d-old seedlings by approximately 25% and 32%, respectively, with respect to control plants at their respective developmental ages. However, prolonged NaCl treatment (up to 6-d stage) decreased the accumulation of GB by ~23% compared to the respective content in plants raised without NaCl stress. This indicates that NaCl stress stimulates GB accumulation, and its content is further modulated by the developmental age of seedlings. In light-grown seedling cotyledons, GB content remained relatively moderate, though salt stress treatment did enhance the level in 4- and 6-d-old stage (). There was no significant difference in the level of GB content in seedling cotyledons raised in the presence of NO donor, DETA in 2- and 4-d-old stage. NO scavenger, DETC treatment, however, resulted in variable response in GB accumulation in seedling cotyledons depending on seedling age. Light downregulated the impact of NO donor treatment on GB content up to 4-d stage (). Thus, seedling age, in other words, tissue sensitivity to NO donor (DETA) and NO scavenger (DETC), was significant and variable with respect to GB accumulation ().
Figure 2. (a) Impact of nitric oxide (NO) on glycine betaine (GB) accumulation in dark-grown sunflower seedling cotyledons raised in the absence or presence of salt (120 mM), NO donor (DETA; 250 μM), and NO scavenger (DETC; 250 μM). (b) BADH activity in seedling cotyledons as affected by NO. (c) Zymographic analysis of BADH activity. (d) Abundance of BADH transcript. Data represent the mean and standard deviation from three replicates (n = 3). NaCl and treatment-induced changes in GB accumulation and BADH abundance and activity were analyzed by one-way ANOVA using SPSS 22.0 and were statistically significant (*p < .05, **p < .01, ***p < .001).
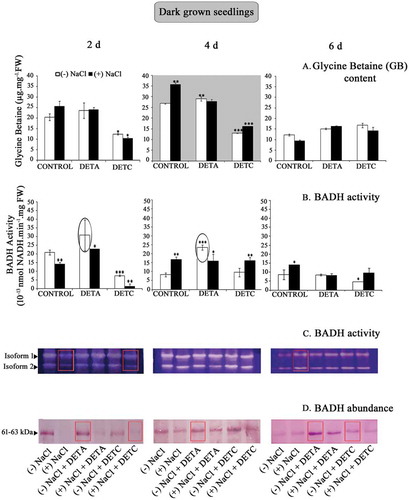
Betaine aldehyde dehydrogenase transcript levels and activity are differentially modulated by NO in light and dark growth conditions
BADH activity, analyzed by NAD+-dependent oxidation of BA, was high in 2- and 4-d-old control seedlings but declined at 6-d stage of seedling growth in sunflower, more so in NO-treated seedlings. Salt stress reduced BADH activity in 2-d-old seedlings by about 32% approximately but increased in 4- and 6-d-old seedling cotyledons to approximately 103% and 62%, respectively, in control conditions. Therefore, positive modulation of BADH activity by salt stress is presumably dependent on seedling age. The effect of NO was evident on BADH activity, and its exogenous application (as DETA) resulted in increased activity in 2- and 4-d-old seedlings. However, the enhancement in activity was higher in seedlings raised without NaCl stress compared to seedling raised in presence of NaCl stress. This indicates that NO might be involved in the regulation of GB accumulation in salt stress through modulation of its biosynthetic enzyme, i.e., BADH. BADH activity was highest in light-grown seedling cotyledons at 4-d stage, and it was not significantly affected by NO or salt stress ().
Figure 3. (a–d) Glycine betaine content and BADH analysis in light-grown seedlings. Other details as in . Data represent the mean and standard deviation from three replicates (n = 3). NaCl and treatment-induced changes in GB accumulation and BADH abundance and activity were analyzed by one-way ANOVA using SPSS 22.0 and were statistically significant (*p < .05, **p < .01, ***p < .001).
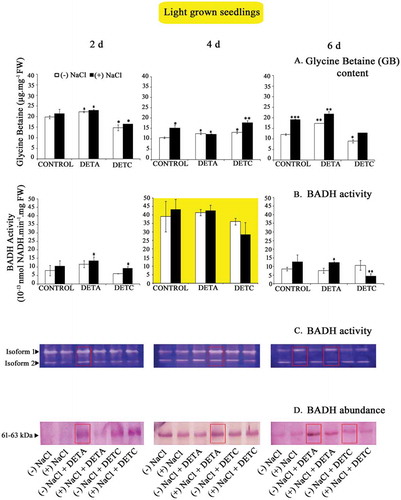
Zymographic analysis of BADH activity highlighted the presence of two BADH isoforms in sunflower seedling cotyledons. The level of expression of BADH transcripts decreased with advancing age and was highest and lowest in 4-d and 6-d-old seedlings, respectively, irrespective of light or dark growth conditions. The activity of both the isoforms was suppressed by salt stress. In 2-d-old, dark-grown seedlings, salt treatment resulted in decreased BADH activity. Zymographically detectable BADH activity was consistently low in 6-d-old seedlings irrespective of NO and salt stress. However, in 6-d-old salt-treated seedlings, BADH activity was enhanced relative to the 2-d-old seedlings grown under salt conditions. The protein extracts of seedling cotyledons showed the presence of single distinctive ~61–63 kDa isozyme of BADH when resolved through the electrophoretic method and detected using hybridized ALDH7A1 antibody (polyclonal ALDH7A1 raised in rabbit/IgG, ThermoFisher Scientific, USA). Salt stress apparently reduced the abundance of BADH enzyme. Treatment of NO donor (DETA) resulted in a marked improvement in BADH abundance at all growth stages in control conditions. The reverse response was evident with NO scavenger treatment (DETC), which reduced the BADH transcript level in the seedling cotyledons at each growth stage, more so under salt stress conditions. These results are concurrent with the high BADH activity observed spectrophotometrically in 4 day old light-grown seedling cotyledons. DETA leads to increase BADH abundance in 6 day old seedlings.
Discussion
The present work highlights that a reasonable amount of GB is being constitutively synthesized in sunflower seedling cotyledons. Sensing of NaCl stress, however, enhances the GB concentration by several folds. Analysis of GB accumulation at three different growth stages of seedling cotyledons (2, 4, and 6 days old) showed that accumulation is age dependent. This is in congruence with the fact that primary synthesis and accumulation of GB occur in young parts of plants, and it is then transported to the older regions/tissues through phloem.Citation32–Citation34 Significant modulation of GB accumulation by salt stress is evident in seedling cotyledons. Independent roles of NO and GB in abiotic stress are known.Citation35–Citation38 Earlier investigations have revealed that endogenous NO derived from nitric oxide synthase (NOS)-like activity in plants can have a possible role in maintaining osmolyte accumulation.Citation16,Citation39 Inhibition of NOS activity affects GB accumulation in maize.Citation35 The present work provides evidence for quenching of NO in solution by GB, indicating a possible direct interaction between the two molecules in plant tissues as well (). The necessary role of NO in maintaining endogenous levels of GB has also been confirmed in the present work by the reduction in GB content caused by NO scavenger (DETC) treatment. NaCl stress and availability of NO regulate BADH activity and, therefore, accumulation of GB. The role of NO in enhancing GB accumulation is maintained through the upsurge in abundance and activity of enzyme BADH. Therefore, it is likely that NO’s involvement in the biosynthetic pathway of GB production in plants takes place prior to the involvement of BADH in converting the toxic intermediate (BA) to GB. Modulation of BADH transcripts and its activity by NO could possibly be an outcome of transcriptional-level regulation of BADH transcripts or a consequence of post translational modification of BADH protein by NO. This aspect needs to be addressed to fully understand the amelioration effects of GB in plants under different abiotic stress. Sunflower seedling cotyledons respond differentially in accumulating GB in the presence and absence of light during the germination growth period of seedlings. Exposing seedling to 16-hr light cycle during their growth resulted in the accumulation of GB in reverse pattern as compared to the seedling grown in dark. This highlights the involvement of a mechanism of active transportation of GB to other plant parts that might be initiated by light. The extent of the impact of NO on GB content and BADH activity also varied with light exposure, but the pattern observed was similar to that observed in dark-grown seedlings. The activity and abundance of BADH were enhanced in response to NO availability, whereas NaCl stress resulted in decreased activity and abundance of BADH. This suggests that light is likely to be involved in initiating the transportation of GB across different parts of the seedling, thereby modulating the extent of accumulation under salt stress.
Figure 4. NO quenching ability of GB. NO released from NO donor (DETA) reacts with NO probe (MNIP-Cu) yielding an excitation peak at 417 nm (ex. 365 nm; em. 420 nm). The peak is, however, completely abolished upon co-addition of GB. DETA and GB were used at 100 μM, whereas MNIP-Cu was used at 2.5 μM as per established procedureCitation41 (MNIP-Cu: a copper derivative 4-mrthoxy-2-(1H-naptho (2, 3-d) imidazol-2-yl) phenol).
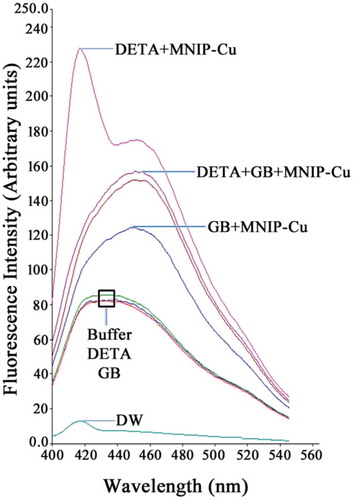
To sum up, sunflower seedling cotyledons constitutively accumulate a moderate amount of GB. Its level increases several folds in response to salt stress or NO availability. Levels of both BADH transcripts and enzyme activity seem to be differentially modulated by salt stress and age of seedlings. NO seems to be a prime regulator of GB accumulation through modulation of abundance and activity of its biosynthetic enzyme, BADH. It seems likely that GB complexed with NO could be the key molecule in eliciting a response. A model proposed in suggests three actions of NO in maintaining GB homeostasis: (1) Preferential mobilization of S-adenosylmethionine (SAM) in the biosynthesis of choline is due to inhibition of aminocyclopropanecarboxylate oxidase (ACC oxidase) activity by NO binding in the cytosolCitation;40 (2) NO further modulates BADH transcript levels and activity; and (3) GB interacts with NO and their complex (GB-NO) could be a key molecule in regulating plant growth under salt stress. These findings hold promise for further interesting work.
Abbreviations
ACC oxidase | = | Aminocyclopropanecarboxylate oxidase |
BA | = | Betaine aldehyde |
BADH | = | Betaine aldehyde dehydrogenase |
CMO | = | Choline monooxygenase |
DETA | = | Diethylenetriamine |
DETC | = | Diethyldithiocarbamate |
GB | = | Glycine betaine |
NAD+ | = | Nicotinamide adenine dinucleotide (oxidized form) |
NO | = | Nitric oxide |
NOS | = | Nitric oxide synthase |
ROS | = | Reactive oxygen species |
SAM | = | S-adenosylmethionine |
Acknowledgments
This work was supported by the Joint University Grants Commission–Israel Science Foundation Research Project (UGC-ISF); [F. No. 6-9/2017(IC)]. Financial assistance to the authors in the form of fellowship was provided by University Grants Commission (UGC), New Delhi, India (Ref No. 21/12/2014(ii)EU-V).
Disclosure of Conflict of Interest
No potential conflict of interest was disclosed.
Additional information
Funding
References
- Singh M, Kumar J, Singh S, Singh VP, Prasad SH. Roles of osmoprotectants in improving salinity and drought tolerance in plants: a review. Rev Environ Sci Biotechnol. 2015;14:1–7. doi:10.1007/s11157-015-9372-8.
- Gupta B, Huang B. Mechanism of salinity tolerance in plants: physiological, biochemical, and molecular characterization. Int J Genom. 2014;2014:Article ID 701596. doi:10.1155/2014/701596.
- Zhang L, Zhao Y, Zhai Y, Gao M, Zhang X, Wang K, Nan W, Liu J. Effects of exogenous nitric oxide on glycine betaine in maize (Zea mays) seedlings under drought stress. Park J Bot. 2012;44:1837–1844.
- Chen THH, Murata N. Glycine-betaine: an effective protectant against abiotic stress in plants. Trends Plant Sci. 2008;13(9):499–505. doi:10.1016/j.tplants.2008.06.007.
- Parihar P, Singh S, Singh R, Singh VP, Prasad SH. Effect of salinity stress on plants and its tolerance strategies: a review. Environ Sci Pollut Res. 2016;22:4056–4075. doi:10.1007/s11356-014-3739-1.
- Ahmad R, Lim CJ, Kwon SY. Glycine betaine: a versatile compound with great potential for gene pyramiding to improve crop plant performance against environmental stresses. Plant Biotechnol Rep. 2013;7:49–57. doi:10.1007/s11816-012-0266-8.
- Sakamoto A, Murata N. The role of glycine betaine in the protection of plants from stress: clues from transgenic plants. Plant Cell Environ. 2002;25(2):163–171. doi:10.1046/j.0016-8025.2001.00790.
- Yadu B, Chandrakar V, Meena RK, Keshavkant S. Glycine beaine reduces oxidative injury and enhances fluoride stress tolerance via improving antioxidant enzymes, proline and genomic template stability in Cajanus cajan L. South African J Bot. 2017;111(1):68–75. doi:10.1016/j.sajb.2017.03.023.
- Faridduddin Q, Varshney P, Yusuf M, Ali A, Ahmad A. Dissecting the role of glycine betaine in plants under abiotic stress. Plant Stress. 2013;7:8–18.
- Chen THH, Murata N. Enhancement of tolerance of abiotic stress by metabolic engineering of betaines and other compatible solutes. Curr Opinion Plant Biol. 2002;5:250–257. doi:10.1016/S1369-5266(02)00255-8.
- Mitsuya S, Kozaki K, Takabe T. Tissue localization of the glycine betaine biosynthetic enzymes in barley leaves. Plant Prod Sci. 2013;16(2):117–122. doi:doi:10.1626/pps.16.117.
- Holmstrom KO, Somersalo S, Mandal A, Palva TE, Welin B. Improved stress tolerance to salinity and low temperature in transgenic tobacco producing glycine betaine. J Exp Bot. 2000;51(343):177–185. doi:10.1093/jexbot/51.343.177.
- Nakamura T, Yokota S, Muramoto Y, Tsutsui K, Oguri Y, Fukui K, Takabe T. Expression of a betaine aldehyde dehydrogenase gene in rice, a glycine betaine non-accumulator, and possible localization of its protein in peroxisomes. The Plant J. 1997;11(5):1115–1120. doi:10.1046/j.1365-313X.1997.11051115.
- Munawar A, Akram NA, Ahmad A, Ashraf M. Nitric oxide regulates oxidative defense system, key metabolites and growth of broccoli (Brassica oleracea L.) plants under water limited conditions. Sci Hortic. 2019;254:7–13. doi:10.1016/j.scienta.2019.04.072.
- Tian F, Wanga W, Lianga C, Wanga X, Wanga G, Wanga W. Over-accumulation of glycine betaine makes the function of the thylakoid membrane better in wheat under salt stress. Crop J. 2016;5(1):73–82. doi:10.1016/j.cj.2016.05.008.
- Zhang M, Zhang H, Li H, Lai F, Li X, Tang Y, Min T, Wu H. Antioxidant mechanism of betaine without free radical scavenging ability. J Agric Food Chem. 2016;64(42):7921–7930. doi:10.1021/acs.jafc.6b03592.
- Kurepin LV, Ivanov AG, Zaman M, Pharis RP, Allakhverdiev SI, Hurry V, Huner NPA. Stress-related hormones and glycine betaine interplay in protection of photosynthesis under abiotic stress conditions. Photosynth Res. 2015;126(2–3):221–235. doi:10.1007/s11120-015-0125-x.
- Wani SH, Singh NB, Haribhushan A, Mir JI. Compatible solute engineering in plants for abiotic stress tolerance – role of glycine betaine. Curr Genom. 2013;14:157–165. doi:10.2174/1389202911314030001.
- Cruz FJR, Castro GLS, Silva-Junior DD, Festucci-Buselli RA, Pinheiro HA. Exogenous glycine betaine modulates ascorbate peroxidase and catalase activities and prevent lipid peroxidation in mild water-stressed Carapa guianensis plants. Photosynthetica. 2013;51(1):102–108. doi:10.1007/s11099-013-0004-7.
- Chen THH, Murata N. Glycine betaine protects plants against abiotic stress: mechanisms and biotechnological applications. Plant Cell Environ. 2011;34:1–20. doi:10.1111/j.1365-3040.2010.02232.x.
- Giri J. Glycine betaine and abiotic stress tolerance in plants. Plant Signal Behav. 2011;6(11):1746–1751. doi:10.4161/psb.6.11.17801.
- Ahmad R, Kim YH, Kim MD, Kwon SY, Cho K, Lee HS, Kwak SS. Simultaneous expression of choline oxidase, superoxide dismutase and ascorbate peroxidase in potato plant chloroplasts provides synergistically enhanced protection against various abiotic stresses. Physiol Plant. 2010;138(4):520–533. doi:10.1111/j.1399-3054.2010.01348.x.
- Sakamoto A, Murata A, Murata N. Metabolic engineering of rice leading to biosynthesis of glycine betaine and tolerance to salt and cold. Plant Mol Bio. 1998;38(6):1011–1019. Erratum in: Plant Mol Bio. 1999; 40(1):195. Murata A. doi:10.1023/A:1006095015717.
- Zhao XX, Ma QQ, Liang C, Fang Y, Wang YQ, Wang W. Effect of glycine betaine on function of thylakoid membranes in wheat flag leaves under drought stress. Biol Plantarum. 2007;51(3):584–588. doi:10.1007/s10535-007-0128-3.
- Cuin TA, Shabala S. Exogenously supplied compatible solutes rapidly ameliorate NaCl-induced potassium efflux from barley roots. Plant Cell Physiol. 2005;46(12):1924–1933. doi:10.1093/pcp/pci205.
- Kader MA, Lindberg S. Cytosolic calcium and pH signaling in plants under salinity stress. Plant Signal Behav. 2010;5(3):233–238. doi:10.4161/psb.5.3.10740.
- Singh N, Bhatla SC. Signaling through reactive oxygen and nitrogen species is differentially modulated in sunflower seedling root and cotyledon in response to various nitric oxide donors and scavengers. Plant Signal Behav. 2017;12(9):e1365214. doi:10.1080/15592324.2017.1365214.
- Valadez-Bustos MG, Aguado-Santacruz GA, Tiessen-Favier A, Robledo-Paz A, Mu~noz-Orozco A, Rasc_on-Cruz Q, Santacruz-Varela A. A reliable method for spectrophotometric determination of glycine betaine in cell suspension and other systems. Anal Biochem. 2016;498:47–52. doi:10.1016/j.ab.2015.12.015.
- Grieve CM, Grattan SR. Rapid assay for determination of water soluble quaternary ammonium compounds. Plant Soil. 1983;70:303–307. doi:10.1007/BF02374789.
- Arakawa K, Takabe T, Sugiyama T, Akazawa T. Purification of betaine-aldehyde dehydrogenase from spinach leaves and preparation of its antibody. J Biochem. 1987;101:1485–1488. doi:10.1093/oxfordjournals.jbchem.a122019.
- Weigel P, Weretilnyk EA, Hanson AD. Betaine aldehyde oxidation by spinach chloroplast. Plant Physiol. 1986;82:753–759. doi:10.1104/pp.82.3.753.
- Annunziata MG, Ciarmiello LF, Woodrow P, Aversana ED, Carillo P. Spatial and temporal profile of glycine betaine accumulation in plants under abiotic stresses. Front Plant Sci. 2019:10. doi:10.3389/fpls.2019.00230.
- Ashraf M, Foolad MR. Roles of glycine betaine and proline in improving plant abiotic stress resistance. Environ Exper Bot. 2005;59(2007):206–216. doi:10.1016/j.envexpbot.2005.12.006.
- Ishitani M, Nakamura M, Han SY, Takabe T. Expression of the betaine aldehyde dehydrogenase gene in barley in response to osmotic stress. Plant Mol Bio. 1995;27(2):307–315. doi:10.1007/BF00020185.
- Ullah S, Kolo Z, Egbichi I, Keyster M, Ludidi N. Nitric oxide influences glycine betaine content and ascorbate peroxidase activity in maize. South African J Bot. 2016;105:218–225. doi:10.1016/j.sajb.2016.04.003.
- Nazia K, Khalid N, Khalid H, Khizar H, Bhatti Ejaz HS, Aqsa T. Effect of exogenous applications of glycine betaine on growth and gaseous exchange attributes of two maize (Zea mays) cultivars under saline conditions. World Applied Sci J. 2014;29(12):1559–1565. doi:10.5829/idosi.wasj.2014.29.12.13971.
- Zhang LX, Li SX, Liang ZS. Differential plant growth and osmotic effects of two maize (Zea mays L.) cultivars to exogenous glycine betaine application under drought stress. Plant Growth Regul. 2009;58(3):297–307. doi:10.1007/s10725-009-9379-7.
- Zhang LX, Li SX, Zhang H, Liang ZS. Nitrogen rates and water stress effects on production, lipid peroxidation and antioxidative enzyme activities in two maize (Zea mays L.) genotypes. J Argon Crop Sci. 2007;193:387–397. doi:10.1111/j.1439-037X.2007.00276.x.
- Gupta N, Thind SK, Bains NS. Glycine betaine application modifies biochemical attributes of osmotic adjustment in drought stressed wheat. Plant Growth Regul. 2014;72(3):221–228. doi:10.1007/s10725-013-9853-0.
- Singh N, Bhatla SC. Nitric oxide regulates lateral root formation through modulation of ACC oxidase activity in sunflower seedlings under salt stress. Plant Signal Behav. 2018;13(5):e1473683. doi:10.1080/15592324.2018.1473683.
- Singh N, Bhatla SC. Hemoglobin as a probe for estimation of nitric oxide emission from plant tissues. Plant Methods. 2019;15:39. doi:10.1186/s13007-019-0425-9.