ABSTRACT
In response to herbivory, poplar produces among other compounds the volatile alcohol 2-phenylethanol and its corresponding glucoside 2-phenylethyl-β-D-glucopyranoside. While the free alcohol is released only upon herbivory, the glucoside accumulates also in undamaged leaves, but increases after herbivore feeding. Recently we showed that 2-phenylethanol and its glucoside are biosynthesized via separate pathways in Populus trichocarpa. The phenylacetaldehyde synthase PtAAS1 plays a central role in the de novo formation of herbivory-induced volatile 2-phenylethanol, while the phenylalanine decarboxylase PtAADC1 initiates a pathway responsible for the herbivory-induced production of 2-phenylethyl-β-D-glucopyranoside. Besides PtAAS1, P. trichocarpa possesses another aromatic aldehyde synthase PtAAS2 with in vitro enzymatic activity comparable to that of PtAAS1. However, in contrast to PtAAS1, which is exclusively expressed in herbivory-damaged leaves, PtAAS2 was found to be expressed at constant levels in both damaged and undamaged leaves. Thus it has been hypothesized that PtAAS2 provides phenylacetaldehyde as substrate for the constitutive formation of 2-phenylethyl-β-D-glucopyranoside in undamaged P. trichocarpa trees. By generating RNAi-mediated AAS2 knockdown plants, we show here that despite the similar activities of PtAAS1 and PtAAS2 in vitro, the latter enzyme does not contribute to the biosynthesis of 2-phenylethyl-β-D-glucopyranoside in planta. Based on the recent finding that phenylpyruvic acid accumulates in undamaged poplar leaves, the constitutive formation of the glucoside may now be suggested to proceed via the Ehrlich pathway, which begins with the conversion of phenylalanine into phenylpyruvic acid.
To defend themselves against herbivores, plants produce a wide array of natural products, including nonvolatile compounds that accumulate in plant tissue and volatiles that are released upon herbivore attack. These compounds can act as toxins, feeding deterrents, and attractants for predators and parasitoids of the herbivores, and can also warn other plant parts or even neighboring plants of impending herbivory.Citation1 Often, plants rapidly biosynthesize such defense compounds after the plant has been damaged.Citation2 Poplar trees, for example, produce and emit phenylalanine-derived volatiles and accumulate phenylalanine-derived nonvolatile metabolites upon herbivory by gypsy moth caterpillars (Lymantria dispar).Citation3–Citation6 The volatile 2-phenylethanol and its corresponding glucoside 2-phenylethyl-β-D-glucopyranoside are major components of this defense reaction.Citation4
Recent research showed that the biosynthesis of volatile and glycosidically bound 2-phenylethanol in poplar proceeds via separate pathways that are either initiated by a cytochrome P450 from the CYP79 family, an aromatic aldehyde synthase (AAS), or an aromatic amino acid decarboxylase (AADC), respectively (;Citation3,Citation4). The AAS and AADC enzymes belong to the class of pyridoxal phosphate group II dependent enzymes, which are known to catalyze diverse enzymatic reactions in vivo.Citation7,Citation8 The phenylacetaldehyde synthase PtAAS1 and the phenylalanine decarboxylase PtAADC1 have been demonstrated to be involved in 2-phenylethanol formation in western balsam poplar (Populus trichocarpa).Citation4 PtAAS1 and PtAADC1 were found to be expressed in herbivore-damaged P. trichocarpa leaves and biochemical characterization of recombinant proteins and RNAi-mediated knockdown experiments revealed that PtAAS1 is involved in the formation of volatile 2-phenylethanol while PtAADC1 provides the substrate for the herbivory-induced production of 2-phenylethyl-β-D-glucopyranoside.Citation4
Figure 1. The biosynthesis of 2-phenylethanol and 2-phenylethyl-β-D-glucopyranoside in poplar. AADC, aromatic amino acid decarboxylase; AAS, aromatic aldehyde synthase; MAO, monoamine oxidase; PAR, phenylacetaldehyde reductase; CYP79, cytochrome P450 family 79 enzyme; AAAT, aromatic amino acid transaminase; TOX, transoximase; PPDC, phenylpyruvic acid decarboxylase; UGT, UDP-glucosyl transferase; β-Glu, β-glucosidase. Dashed lines indicate enzymes/reactions not yet characterized in planta. Solid lines indicate characterized poplar enzymes, and dotted lines indicate enzymes characterized in other plants.
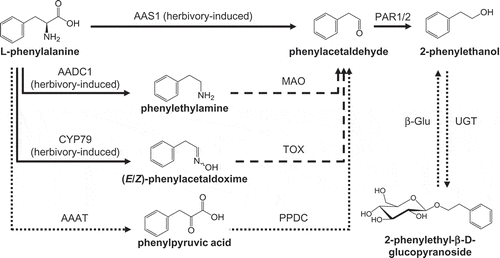
Besides PtAAS1, another aromatic aldehyde synthase, PtAAS2, was recently identified in P. trichocarpa. As PtAAS1, PtAAS2 catalyzes the conversion of L-phenylalanine to phenylacetaldehyde with reasonable kinetic parameters in vitro, suggesting that it also acts as a phenylacetaldehyde synthase in planta.Citation4 However, in contrast to PtAAS1, which is strongly expressed in herbivore-damaged leaves, PtAAS2 showed high expression in undamaged leaves. Thus, it was hypothesized that PtAAS2 produces phenylacetaldehyde as substrate for the constitutive formation of 2-phenylethyl-β-D-glucopyranoside in undamaged P. trichocarpa leaves.Citation4
To test this hypothesis, we studied the biological function of AAS2 via Agrobacterium tumefaciens-mediated RNAi of PcanAAS2 in gray poplar (P. x canescens) as described previously.Citation4 In brief, sterile stem internodes and leaf material of P. x canescens was infiltrated with A. tumefaciens carrying an antisense sequence complementary to PtAAS2. Transgenic saplings were generated and propagated via callus cultures derived from four independent transgenic events. For comparisons, we included wild type trees and trees transformed with an empty vector into the experiment. Plants were grown until a height of 1 meter and leaves (leaf plastochron index LPI3-10) were harvested for gene expression analysis and liquid chromatography-tandem mass spectrometry (LC-MS/MS) measurements as described in Günther et al.Citation4 Real time-quantitative PCR experiments revealed that the expression of PcanAAS2 was significantly decreased in all PcanAAS2 RNAi lines in comparison to wild type trees and the empty vector controls (). However, the downregulation of PcanAAS2 did not influence the accumulation of 2-phenylethyl-β-D-glucopyranoside (). These results suggest, contrary to our original hypothesis, that AAS2 does not contribute to the constitutive formation of glucosylated 2-phenylethanol in poplar.
Figure 2. Transcript accumulation of PcanAAS2 (a) and accumulation of 2-phenylethyl-β-D-glucopyranoside (b) in undamaged leaves of Populus x canescens wild type plants (WT), empty vector control plants (EV), and PcanAAS2 RNAi lines (AAS2-RNAi). (a) Gene expression was analyzed using real time-quantitative PCR and the relative normalized expression compared to the reference gene ubiquitin is shown. (b) 2-Phenylethyl-β-D-glucopyranoside was extracted with methanol from ground leaf material and analyzed via liquid chromatography-tandem mass spectrometry. Biological replicates (nb) and technical replicates (nt) of EV lines and RNAi lines were used to test for statistical differences. WT, nb = 5; EV, nb = 3, nt = 5; AAS2-RNAi, nb = 4, nt = 5 (AAS2-RNAi-2, nb = 4, nt = 4). Asterisks indicate statistical significance as assessed by Student’s t tests. PcanAAS2 expression (P < 0.001, t = 8.934); 2-phenylethyl-β-D-glucopyranoside accumulation (P = 0.792, t = −0.266). Medians ± quartiles and outliers are shown. Each data point is represented by a circle. ns, not significant.
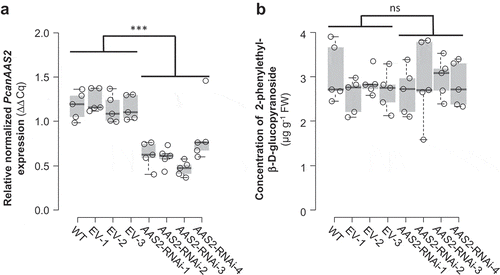
In general, plant aromatic aldehyde synthases can function in various biosynthetic pathways. Our study revealed that PtAAS2 does not play a role in the formation of 2-phenylethyl-β-D-glucopyranoside in poplar. However, the in vitro characterization of PtAAS2 showed that this enzyme is able to convert not only phenylalanine into the corresponding phenylacetaldehyde, but also tryptophan into indole-3-acetaldehyde.Citation4 Although the kinetic parameters for the conversion of tryptophan have not yet been determined, it is tempting to speculate that PtAAS2 produces mainly indole-3-acetaldehyde in planta as a precursor for other tryptophan-derived metabolites. The fact that the expression of PtAAS2, in contrast to PtAAS1, was not influenced by herbivory further suggests that PtAAS2 is not involved in the defense reaction against herbivores in poplar.
Because PtAAS2 can now be ruled out as candidate for the formation of the basal levels of 2-phenylethyl-β-D-glucopyranoside in undamaged poplar leaves, it is likely that another pathway provides the required phenylacetaldehyde substrate in these leaves. The stable accumulation of phenylpyruvic acid in undamaged and damaged P. trichocarpa leavesCitation4 indicates that the Ehrlich pathway might play a role in constitutive 2-phenylethyl-β-D-glucopyranoside accumulation. The Ehrlich pathway was originally elucidated in yeast and requires the action of an aromatic amino acid transaminase (AAAT) and a phenylpyruvic acid decarboxylase (PPDC) (; for review see Hazelwood et al.Citation9). Both enzymes have been identified in melonCitation10 and roseCitation11 and it would thus be worthwhile to investigate potential AAAT and PPDC candidates in future studies of 2-phenylethyl-β-D-glucopyranoside biosynthesis in poplar.
Disclosure of Potential Conflicts of Interest
No potential conflicts of interest were disclosed.
Author contributions
TGK, JG, and JGe designed the research, JG and AS carried out the experimental work, JG analyzed data, TGK and JG wrote the manuscript, all authors read and approved the final manuscript.
Acknowledgments
We thank Danny Kessler and all the MPI-CE gardeners for their help with rearing the poplar plants, and Marion Stäger for excellent technical assistance.
Additional information
Funding
References
- Unsicker SB, Kunert G, Gershenzon J. Protective perfumes: the role of vegetative volatiles in plant defense against herbivores. Curr Opin Plant Biol. 2009;12:1–3.
- Pichersky E, Noel J, Dudareva N. Biosynthesis of plant volatiles: nature diversity and ingenuity. Science. 2006;311:808–811. doi:10.1126/science.1118510.
- Irmisch S, Mccormick C, Boeckler GA, Schmidt A, Reichelt M, Schneider B, Block K, Schnitzler J, Gershenzon J, Unsicker SB, et al. Two herbivore-induced cytochrome P450 enzymes CYP79D6 and CYP79D7 catalyze the formation of volatile aldoximes involved in poplar defense. Plant Cell. 2013;25:4737–4754. doi:10.1105/tpc.113.118265.
- Günther J, Lackus ND, Schmidt A, Huber M, Stödler H-J, Reichelt M, Gershenzon J, Köllner TG. Separate pathways contribute to the herbivore-induced formation of 2-phenylethanol in poplar. Plant Physiol. 2019;180:767–782. doi:10.1104/pp.19.00059.
- McCormick AC, Boeckler GA, Köllner TG, Gershenzon J, Unsicker SB. The timing of herbivore-induced volatile emission in black poplar (Populus nigra) and the influence of herbivore age and identity affect the value of individual volatiles as cues for herbivore enemies. BMC Plant Biol. 2014;14:304. doi:10.1186/s12870-014-0304-5.
- Danner H, Boeckler GA, Irmisch S, Yuan JS, Chen F, Gershenzon J, Unsicker SB, Köllner TG. Four terpene synthases produce major compounds of the gypsy moth feeding-induced volatile blend of Populus trichocarpa. Phytochemistry. 2011;72:897–908. doi:10.1016/j.phytochem.2011.03.014.
- Torrens-Spence MP, Pluskal T, Li FS, Carballo V, Weng JK. Complete pathway elucidation and heterologous reconstitution of Rhodiola salidroside biosynthesis. Mol Plant. 2017;11:205–217. doi:10.1016/j.molp.2017.12.007.
- Facchini PJ, Huber-Allanach KL, Tari LW. Plant aromatic L-amino acid decarboxylases: evolution, biochemistry, regulation, and metabolic engineering applications. Phytochemistry. 2000;54:121–138. doi:10.1016/s0031-9422(00)00050-9.
- Hazelwood LA, Daran JM, Van Maris AJA, Pronk JT, Dickinson JR. The Ehrlich pathway for fusel alcohol production: a century of research on Saccharomyces cerevisiae metabolism. Appl Environ Microbiol. 2008;74:2259–2266. doi:10.1128/AEM.02625-07.
- Gonda I, Bar E, Portnoy V, Lev S, Burger J, Schaffer AA, Tadmor Y, Gepstein S, Giovannoni JJ, Katzir N, et al. Branched-chain and aromatic amino acid catabolism into aroma volatiles in Cucumis melo L. fruit. J Exp Bot. 2010;61:1111–1123. doi:10.1093/jxb/erp390.
- Hirata H, Ohnishi T, Tomida K, Ishida H, Kanda M, Sakai M, Yoshimura J, Suzuki H, Ishikawa T, Dohra H, et al. Seasonal induction of alternative principal pathway for rose flower scent. Sci Rep. 2016;6:20234. doi:10.1038/srep20234.
- Pare PW, Tumlinson JH. Update on plant-insect interactions plant volatiles as a defense against insect herbivores. Plant Physiol. 1999;121:325–331.