ABSTRACT
Sunflower plants are semi-tolerant to salt stress. Calcium modulates the expression of oubain-sensitive ATPases, responsible for sodium fluxes in cells. Salt stress delays degradation of oil body (OB) membrane proteins. Serotonin and melatonin contents are elevated in response to salt stress. Melatonin can detoxify the seedlings of elevated reactive oxygen species (ROS) levels. Enhanced nitric oxide (NO) expression correlates with NaCl-induced modulation of seedling growth. Salt stress enhances S-nitrosylation of cytosolic proteins in seedling cotyledons, while in roots, denitrosylation of proteins is observed. Lipid peroxide content and glutathione peroxidase (GPX4) activity are enhanced in response to salt stress. Salt stress downregulates the activity of superoxide dismutase (SOD) and upregulates the activity of GPX4 and glutathione reductase (GR). Heme oxygenase-1 (HO-1) abundance in cells surrounding the secretory canal in seedling cotyledons is enhanced in response to salt stress. NO negatively regulates the total glutathione homeostasis and regulates polyamine and glycine betaine homeostasis in response to salt stress. An intricate biochemical crosstalk is thus observed to control salt tolerance mechanisms in sunflower.
Stress tolerance in living organisms is a manifestation of several interlinked biochemical process.Citation1,Citation2 Salinity is one of the major factors that induces significant decrease in crop yield, especially in arid and semi-arid regions. The sensitivity and tolerance of halophytes and glycophytes to salt stress are quite differentCitation3 While halophytes (like sugarbeet) are not significantly affected by salt stress, glycophytes (like soybean) are highly sensitive to salt in soil/growth medium. Plants adapt to salinity stress using three main strategies: osmotic stress tolerance, toxic ion (Na+, Cl–) exclusion and tissue tolerance to toxic ions.Citation1,Citation4 In saline conditions, plants try to acquire essential elements like K+ and Ca2+ while avoiding the uptake of toxic ions, such as Na+ and Cl–5 Sunflower (Helianthus annuus L.) cultivars exhibit large variations in their tolerance to salt stress. They can be very sensitive, moderately sensitive or semi-tolerant to salt stress. Based on investigations using crop water-stress index, sunflower can be categorized as a salt semi-tolerant cropCitation6 Salinity-induced stress in sunflower leads to visible aberrations in plant growth right from seedling stage. It reduces hypocotyl elongation and root proliferation, causes browning of root tips, and suppresses cotyledon expansion in seedlings. In young plants, burning (necrosis) of leaves, reduced inflorescence and delayed seed set are the major morphological deviations in response to salt stress. Present review provides a critical analysis of the information available so far on the pattern and mechanisms of sodium ions accumulation and its impact on long distance sensing of salt stress by the way of oil body (OB) mobilization in seedling cotyledons. Recent findings on nitric oxide (NO) as a signaling molecule in salt stress induced impact of reactive oxygen species (ROS), ROS scavenging enzymes, modulation of their activity by tyrosine nitration and S-nitrosylation, polyamine biosynthesis and glycine betaine (GB) homeostasis provide new information on salt stress tolerance in sunflower.
Sodium ion toxicity is modulated by calcium
Accumulation of sodium and chloride ions is higher in the vegetative parts of sunflower plants as compared to reproductive partsCitation3 X-ray microanalysis has shown that root cortical cells act as a deposit site for sodium and chloride ions. High levels of sodium ions inhibit radial movement of calcium ions in the cell. The drop in calcium ion content is significantly higher in the cytoplasm than in intracellular spaces. Under salinity stress, calcium ions are redistributed toward the xylem and are transported to young leaves to counter its deficiency in the plant.Citation3,Citation7,Citation8
While sodium ions accumulate in the cortical cells, Cl– accumulation is largely in the epidermal cells of rootsCitation8 Toxic levels of Na+ accumulation can be countered by external application of CaSO4 as a source of Ca2+. Calcium ions tend to limit the radial movement of sodium ion in cells, thus facilitating salt tolerance in plants. Experimental studies on Arabidopsis thaliana and rice have demonstrated that Na+ is the principal lethal ion responsible for causing toxicity in the cell. However, in soybean and sunflower, salinity-induced damage is also related to chloride ions in addition to sodium. Chloride content in the cells of sunflower roots is higher than sodium, indicating differential chloride uptake by sunflower roots.Citation3,Citation9,Citation10 Restriction of toxic ions to older plant parts is an acclimation response to salt stressCitation11 Under high salinity, chloride ions accumulate in the leaves of sunflower and are responsible for necrosis at the leaf tip. Chloride ions taken up by the roots are transported to the leaves mainly along the transpiration streamCitation12 In sunflower, leaf injury in response to salt stress is caused primarily by Cl– toxicityCitation3 NaCl stress is also responsible for reduced photosynthetic efficiency of seedlings and plants. However, the individual effects of toxic ions (Na+ and Cl–) differ. Accumulation of Cl– in plant cells diminishes their photosynthetic efficiency and quantum yield due to degradation of chlorophyll. This may result from the ‘Cl–-induced structural disruption’ of PSII. High Na+ concentration disrupts the ionic balance of the cells by interfering with K+ and Ca2+ uptake. Consequently, photosynthesis and growth are visibly reducedCitation12 Active loading of Na+ into the xylem is also a key attribute in imparting salt tolerance to plantsCitation13 Na+/H+ antiporters are involved in the pumping of Na+ out of the cells into the xylemCitation14 Calcium content is also considerably altered in the presence of NaCl. It not only strengthens the cell structurally but also protects the cell from membrane-associated damage and ion leakageCitation15 Under salt stress, the cell wall associated calcium is displaced by sodium ions, which leads to an increase in cell permeability. This might be a strategy to enhance salt-tolerance by enhancing calcium-loading in xylemCitation8 Calcium is transported via apoplast to reduce calcium deficiency in young leavesCitation10
A negative correlation exists between the biomass of different plant parts and their respective Na+ and Cl– contents suggesting that reduced plant growth is mainly due to ion specific toxicity, nutrient deficiency and osmotic stress in the tissueCitation16 A positive correlation exists between salt tolerance and selective Na+ exclusion during early stages of growth and development of sunflower. Roots are efficient barriers for transport of sodium ions across the plant. Sodium gets accumulated in plant parts, like roots and lower shoot, to protect the aerial tissue from ion specific toxicity. This pattern of sodium ion movement and its selective accumulation in the roots of plants (generally, glycophytes) is an important salt tolerance mechanism to protect its aerial and reproductive tissues from ion-induced toxicity. Evolution of P-type ATPases, such as Na+, K+ ATPases in plants for sodium ion efflux, has also been investigated in author’s laboratory using techniques like fluorescence imaging and spectrofluorometry.Citation1,Citation8,Citation16,Citation17 Salinity seems to induce OU-sensitive ATPase activity in the meristematic cells of roots under salt stress (). These ATPases are localized in the nuclear and plasma membrane of salt-stressed seedling roots, and have a role in maintenance of Na+/K+ gradient across the cytosol and nucleusCitation18 Calcium significantly inhibits the expression of oubain-sensitive ATPases. Treatment with calcium ion chelator, EGTA (5 mM) helps in recovery and expression of fluorescence due to enzyme activity in the cells of root tips.
Figure 1. (a) Spatial distribution of sodium ions in seedling roots in response to salt (120 mM NaCl) stress, observed using Sodium Green. Profuse accumulation of sodium ions is observed in the meristematically active and elongation zones as a response to NaCl stress (unpublished data). (b). Salt stress–induced expression of oubain-sensitive ATPase in the root tips of 2 day old, dark grown sunflower seedlings. Oubain-sensitive ATPase expression is observed post incubation with 50 µM AO (Anthroyl oubain). Calcium significantly inhibits the expression of oubain-sensitive ATPases. Treatment with calcium ion chelator, EGTA (5 mM) helps in recovery and expression of fluorescence due to enzyme activity in the cells of root tips (Adapted from Mukherjee and Bhatla, 2014).
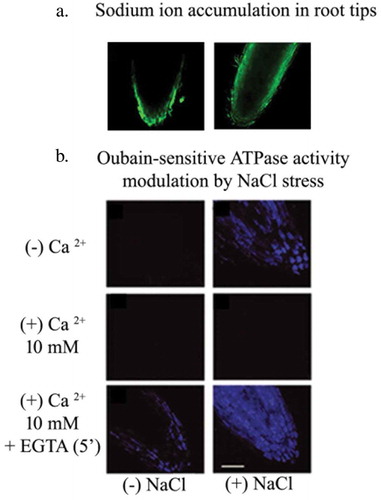
Salt stress delays oil body mobilization during seedling growth
OB mobilization is a major metabolic event that occurs in oilseeds, wherein TAGs compacted within the oil bodies are hydrolyzed by the action of cytosolic lipases. The phospholipid monolayer enveloping the storage lipids are studded with OB membrane proteins, such as oleosins, steroleosins and caleosins.Citation19,Citation20 Oleosins are known for maintaining structural integrity and sustaining the OBs in their uncastrated forms at low water potential. Oleosins are found exclusively in seeds and pollens, both of which face dehydration stress after maturationCitation21 Author’s laboratory has examined various physiological and biochemical signaling events involved in seed germination-associated OB mobilization pattern under salt stressCitation16 A correlation between the activity of a 65 kDa-cytosolic protease (responsible for degradation of OB membrane proteins) and extent of OB mobilization has been demonstratedCitation21 Differential expression pattern of OB membrane proteins and rate of TAG mobilization has also been observed in using light and dark-grown sunflower seedlings.Citation22,Citation23 Salt stress delays the degradation of OB membrane proteins in sunflower seedlingsCitation16
Hydrolysis of OB membrane proteins is followed by the breakdown of triacylglyerides (TAGs) by lipase action, the principal enzyme involved in mobilization of stored lipid reserves, during seedling growth. Enzymes like lipoxygenase (LOX), lipase and thiol protease play a significant role in mobilization of storage lipids during seed germinationCitation24 LOX catalyzes the oxygenation of polyunsaturated fatty acids (PUFA), followed by utilization of storage lipids. Under salt stress, LOX activity is significantly elevated in seedling cotyledons. Thiol protease and lipase are responsible for mobilization of proteins and subsequent release and hydrolysis of storage lipids (TAGs).Citation21,Citation25
Serotonin and melatonin (indoleamines)-modulate extension growth of salt-stressed seedlings
Serotonin and melatonin are antioxidants produced under stress and regulate abiotic stress tolerance in plantsCitation18 Tryptophan is the precursor molecule for the biosynthesis of serotonin and melatonin in plants. Root and shoot tips are the primary sites for the biosynthesis of plant hormone, auxin, antioxidant, and serotonin. The key enzymes involved in the biosynthesis of serotonin are 2-Tryptophan Decarboxylase (TDC), which mediates the formation of tryptamine, followed by its catalysis to serotonin by the action of Tryptamine 5-hydroxylase (T5H). Tryptamine is the branching point in the biosynthetic pathway of indoleamines. It can be catalyzed to either indole 3-acetic acid (IAA) or serotoninCitation26 Melatonin biosynthesis involves enzymes acting downstream to serotonin biosynthetic pathway. They are serotonin N-acetyltransferase (SNAT) and hydroxyindole-O-methyltranferase (HIOMT). HIOMT is the rate limiting enzyme that catalyzes the synthesis of melatonin from N-acetyl serotonin.Citation27-Citation29 Salt stress causes elevation in the endogenous levels of serotonin (5-hydroxytryptamine) and melatonin (N-acetyl 5-methoxytryptamine) and their differential spatial distribution in the roots and cotyledons of sunflower seedlings. It may be stipulated that these molecules have a role in salt-induced long distance signaling from roots to cotyledons. Accumulation of these morphoregulators in the cytosol of cells of cotyledons is supposedly correlated with the delayed mobilization of oil bodies under salt stress. Evidence suggests that these molecules might also be involved in regulating root growth of salt-stressed seedlings. Serotonin and melatonin are likely to impart abiotic stress tolerance to plants, either by altering hormone metabolism or by acting as antioxidant to scavenge free radicals or ROS and reactive nitrogen species (RNS).Citation27,Citation30,Citation31
Upregulation of serotonin biosynthesis accompanies NaCl-induced constraints in root growth, and limited availability of auxin to root cells. Abiotic stress induced accumulation of serotonin in plant tissue is likely to inhibit auxin biosynthesis. Stress-triggered deposition of serotonin mainly occurs in the vascular cells of primary root and oil bodies of cotyledons of young sunflower seedlingsCitation18 Similarly, melatonin levels are also elevated under conditions of stress. Salinity stress is known to affect the activity of HIOMT, the key enzyme involved in melatonin biosynthesis, responsible for catalyzing the formation of melatonin from N-acetylserotonin. Melatonin can detoxify the seedlings of ROS [hydroxyl radical, (OH–) and hydrogen peroxide (H2O2)] and RNS [peroxynitrite anion (ONOO–), NO and peroxyl radical (LOO–)] by direct scavengingCitation27
Exogenous application of these indoleamine-derivatives partially counters growth inhibition caused by salt stressCitation18 In the absence of salt stress, serotonin is primarily localized in symplast of the root cells. Salt stress triggers serotonin accumulation in the pericycle, endodermis and vascular bundle (protoxylem and protophloem) as well. Their concentration gradually increases with the age of seedlings. Application of suberin-specific fluorescent stain, berberine hemisulphate, revealed that serotonin is associated with the non-suberized, immature cells of endodermis. Thus, NaCl-induced serotonin biosynthesis is prominent in sunflower seedlings. Similarly, profuse accumulation of melatonin is observed in the cortex and vascular bundles of roots under unstressed (–NaCl) growth conditions. Salt treatment, however, triggers its accumulation in the cortical cells of primary roots. Melatonin biosynthetic enzyme, HIOMT, shows a five-fold increase in seedling cotyledons derived from seedling raised in presence of NaClCitation32
Accumulation of reactive oxygen species (ROS) in response to salt stress triggers differential expression of ROS scavenging enzymes
High salt concentrations in the growth medium often result in generation of free radicals and (ROS) in plant cells. These high energy molecules are produced as byproducts of metabolic pathways and includes O2–֗, H2O2, OH֗.Citation33,Citation34 A sudden rise in their concentration (oxidative burst) results in severe oxidative stressCitation35 leading to blemishing of the electron transport system within chloroplasts and mitochondria. Stress-induced imbalance in the levels of ROS induces lipid peroxidation in the membranes and denaturation of proteins and nucleic acids.Citation36-Citation38 Expression of molecules with ability to scavenge (ROS) is enhanced in response to salt stress. SOD is the main scavenger of superoxide anions (O2–֗) and forms primary line of defense against ROS in the cells by carrying out the dismutation of two superoxide anions (O2–֗) with H2O to form H2O2 and O2Citation39 It acts on superoxide anions as these ions are impermeable to phospholipid membrane, and thus can aggregate in the cell at toxic levels under stress. H2O2, the byproduct produced upon dismutation of superoxide anions, can freely move across the cell membrane and functions as a signaling molecule that spreads ‘alert signals’ to the plant tissue so that it can prepare its defense to combat stress induced ROS production in the cells. SOD is reported to have three isoforms, namely iron SOD (FeSOD), manganese SOD (MnSOD) and copper-zinc SOD (Cu-ZnSOD), which are differentially affected by salt stress ()Citation40 In sunflower seedlings raised in the presence of NaCl, SOD isoforms show differential spatial and temporal modulation in their responseCitation41 (). Activity of chloroplast-based FeSOD (40 kDa) is lowered under salt stress while Cu-ZnSOD (32 kDa) activity is enhanced as the seedling ages. Tissue-specific differential expression is observed in isoforms of SOD. Activity of FeSOD is higher in roots as compared to cotyledons. On the other hand, activity of cytoplasmic Cu-ZnSOD is greater in cotyledons than in roots. The differential spatial distribution of isoforms and sensitivity to salt stress indicates crucial compartmentalization of superoxide anions as stress induced rapid response H2O2 produced by the action of SOD is further reduced by action of peroxidases, which catalyze the reduction of H2O2 and its derivatives. Out of the five major peroxidases present in plant cells, author’s laboratory focused work on phospholipid hydroperoxidase/glutathione peroxidase (PHGPX or GPX4)Citation42,Citation43 GPX4 is the principal savior of phospholipid biomembranes against oxidative destruction. It catalyzes the reduction not only of hydrogen peroxides but also organic hydroperoxides and lipid peroxides to H2O and corresponding alcohol, using reduced glutathione (GSH). High glutathione hydroperoxide activity is evident in young seedlings under salt stress, indicating its early signaling role in response to NaCl stress. It is thus evident that under salinity stress, production and accumulation of lipid peroxides is followed by upregulation of GPX4 activity in etiolated sunflower seedlings cotyledonsCitation43 Stress perception by oilseeds is likely to alter the OB mobilization pattern by lipid peroxidation of the OB membrane proteins.
Sensitivity of different antioxidant enzymes to salt stress is variable. Salt stress downregulates the activity of antioxidants enzymes, such as superoxide dismutase (SOD), and upregulates the activity of enzymes like GPX4 and glutathione reductase (GR), in sunflower seedlings. Also, differential spatial distribution of antioxidant enzymes is evident in sunflower roots and cotyledons, indicating that long-distance signaling mechanisms are actively used to impart salt stress tolerance in oilseedsCitation41 With increasing ROS content in salt-stressed sunflower seedlings, an increase in GR activity is observed in cotyledons of etiolated seedling. A decline in GSH/GSSG content is evident under salt stress in seedling cotyledons. Elevation in total glutathione content is more significant in salt stressed cotyledons. Thus, there exists a correlation between GR activity and GSH concentration in sunflower seedlings. Modulation of GR activity is likely to affect the pool of GSH and GSSG. The abundance of GR in epidermal cells and secretory canal cells is notably higher under salt stress, highlighting the impact of salinity on spatial distribution of GR in seedling cotyledonsCitation32
Heme, an iron sensor in plants, is highly toxic to the cell in free form and catalyzes the formation of (ROS). HO or Heme oxygenase (EC 1.14.99.3) is the antioxidant enzyme responsible for the catabolic breakdown of toxic heme. HO-1 has been reported to provide protection to plant cells against several abiotic stress factors, such as salinity, low temperature and heavy metals.Citation44-Citation46 Immunolocalization of HO-1 enzyme has provided evidence pertaining to its distribution pattern in sunflower seedling cotyledons. HO-1 is abundantly present in cells surrounding the secretory canal in seedling cotyledons. Its abundance is further elevated in response to salt stress. Tyrosine nitrated proteins show a similar pattern of distribution in seedling cotyledons, with enhanced accumulation under salt stress conditions. The spatial distribution pattern of HO-1 and tyrosine nitrated proteins, in correlation with abundance of mitochondria in the specialized cells surrounding secretory canals of cotyledons, could be a possible long-distance mechanism of salt-stress acclimation in sunflower seedlings. NO, made available through exogenous application of NO donars (SNP, sodium nitroprusside and DETA, diethylenetriamine), also modulates HO-1 activity under salt stressCitation47
Nitric oxide (NO) signaling – the key molecule in molecular crosstalk regulating salt stress
NO is a gaseous signaling molecule in plants. It shows a biphasic mode of action. At high concentration, it acts like a prooxidant and reacts with superoxide anions to form highly unstable peroxynitrite anions (ONOO–). At low concentrations, NO inhibits reactions leading to lipid peroxidation and thus, functions like an antioxidantCitation48 In sunflower seedlings, NO expression correlates with NaCl-induced modulation of seedling growth, Na+/K+ ratio, ATPase activity and OB mobilization.
Salt stress enhances the activity of arginine-dependent putative nitric oxide synthase (NOS) activity and consequently, higher level of NO is produced. The NO content of roots increases in seedlings. In contrast to roots, cotyledons show a gradual drop in NO level with increasing ageCitation16 The levels of NO are exceptionally high on the surface of oil bodies containing in the cells of seedling cotyledons and it seem to have a role in delaying the degradation of oil bodies and subsequent TAG hydrolysis. Oil bodies obtained from cotyledons of sunflower seedlings raised under salt stress show increased accumulation of NOCitation49 NO mediates several modifications in the protein structure. These include tyrosine nitration, S-nitrosylation and metal nitrosylationCitation50 Protein tyrosine nitration is an established marker for nitrosative stress. Amplified gradient of tyrosine-nitrated proteins has further been observed in seedling roots grown in the presence of NaCl from tips toward the zone of differentiation (). Noteworthy enhancement in tyrosine nitration of both, cytosolic and OB membrane proteins, has been observed in sunflower seedling cotyledonsCitation49 Eight major cytosolic proteins exhibit intense tyrosine nitration in response to salt stress. Salt stress elevates S-nitrosylation of cytosolic proteins in seedling cotyledons, while in roots, denitrosylation of proteins is observed. S-nitroglutathione reductase (GSNOR) activity is inhibited under salt stressCitation51,Citation52 (). These attributes are likely to provide longevity to oil bodies (slow mobilization) for survival of seedlings. It can result in activation/deactivation of target proteinsCitation53
Figure 2. (a) Temporal distribution of nitric oxide (NO) in roots of 2 day old sunflower seedlings. (Adapted from David et al., 2010). (b). Tyrosine-nitrated protein distribution in 2 day old dark-grown seedlings roots in the absence or presence of NaCl stress enhances tyrosine-nitrated protein distribution in seedling roots in NaCl stress (120 mM) (Adapted from David et al., 2015). (c) S-Nitrosylation of proteins is a mechanism that revolves around the nitric oxide (NO) mediated effects in sunflower seedlings in response to salt (Adapted from Jain et al., 2018).
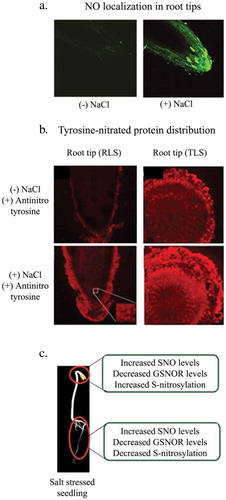
Nitric oxide crosstalks with ROS scavenging enzymes and indoleamines in regulating salt stress
Activity of antioxidant enzymes, such as SOD, GPX, GR and HO-1, is enhanced after exogenous application of NO, thus ameliorating their response to salt stressCitation32,Citation41,Citation43 (). Crosstalk of NO with various antioxidant enzymes has been analyzed in salt stressed sunflower seedlings raised in various concentrations and combinations of NO scavenger (cPTIO, DETC(O,O diethylthiophosphoryl chloride)), NO donar (SNP, DETA), NOS inhibitor (aminoguanidine) and peroxynitrite. Exogenous NO provided as SNP (1000 µM), elevates the activity of FeSOD and Zn-CuSOD in salt stressed roots of sunflower seedlings. NO acts both as a positive and negative regulator of SOD isoforms depending on the tissue (roots or cotyledons) or NaCl stressCitation41 However, cPTIO has an inhibitory effect on GR activity. Total glutathione content cannot be modulated by the application of NO donor although a significant rise has been noted in glutathione content in presence of NO quencher, indicating that NO negatively regulates the total glutathione homeostasis in plant cells. Interestingly, NO and melatonin have inverse effect on the accumulation of total glutathione content. While NO limits glutathione accumulation, melatonin promotes itCitation32 Salt stress induces melatonin accumulation in sunflower seedlings as a long distance signaling response. NO further enhances melatonin accumulation in salt stressed seedling cotyledons (). Differential spatial distribution and modulation of SOD isoforms – Cu-ZnSOD and MnSOD is observed in response to melatonin.Citation18,Citation53, Citation54
Figure 3. (a) Interactions among melatonin, nitric oxide and superoxide dismutase (SOD) as a consequence of NaCl (120 mM) stress (Adapted from Arora & Bhatla,2017). (b) Differential modulation of SOD isoforms by NO points toward separate intracellular pathways with their functional separation. Its concentration-dependent biphasic (pro- and antioxidant) nature of action is observed as SOD isoforms are affected variably by the presence of NO (Adapted from Arora and Bhatla, 2015).
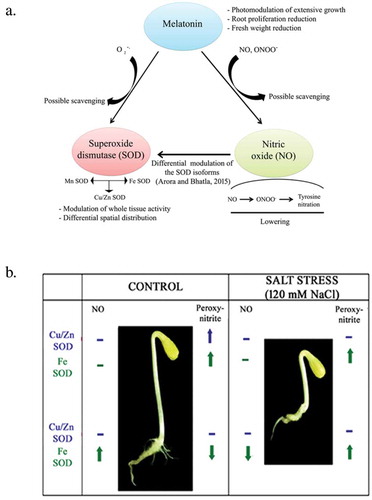
Nitric oxide regulates glycine betaine and polyamines homeostasis
Sunflower seedling cotyledons have been observed to constitutively accumulate some amount of GB, an osmolyteCitation55 Sensing of salt stress enhances tissue GB levels by several folds. NO appears to be a key regulator of GB accumulation in seedling cotyledons through modulation of the activity and abundance of betaine aldehyde dehydrogenase (BADH), the key biosynthetic enzyme for GB. NO also appears to facilitate the seedlings’ adaptability to salt stress by way of regulation of polyamine (PA) homeostasisCitation56 This has been observed to be brought about through regulation of PA distribution among free, conjugated, bound forms and enhanced accumulation of PA biosynthetic enzymes, namely arginine decarboxylase (ADC) and S-adenosine methionine decarboxylase (SAMDC), as well as by lowering the rate of PA catabolism by way of its impact on polyamine oxidase (PAO) activity.
To sum up, significant recent investigations highlight novel biochemical and molecular interactions governing salt tolerance in sunflower plants during early phase of growth. Deciphering the crucial role of oubain-sensitive ATPase further will provide evidence for mechanisms regulating sodium efflux. S-nitrosylation and tyrosine nitration of ROS scavenging enzymes and OB membrane proteins are likely to provide longetivity to oil bodies under stress conditions. Work on NO acting as a key molecule in GB and polyamine homoestasis needs to be taken up for further investigations. GB has been recently shown to interact with NOCitation55 GB-NO could be one of the key molecular interactions regulating plant growth under salt stress. NO and melatonin together seem to serve as key molecules through which plant cells are likely to facilitate salt tolerance at molecular level through their multifarious biochemical interactions. Levels of ascorbic acid can reach up to 20–50 mM in plant cells, and are escalated by stress. Recently, anion channels responsible for transport of ascorbate across plasma membrane have been found to be stimulated by NaClCitation57 Release of plant hemoglobin can hypothetically be correlated with enhanced ascorbate levels to combat salt stressCitation58
Abbreviations
Acknowledgments
Authors gratefully acknowledge support from UGC-ISF Joint research project [(F.No-6-9/2017 (IC)].
Additional information
Funding
References
- Keisham M, Mukherjee S, Bhatla SC. Mechanisms of sodium transport in plants-progresses and challenges. Int J Mol Sci. 2018;19(3):1. doi:10.3390/ijms19030647.
- Singh N, Bhatla SC. Nitric oxide regulates lateral root formation through modulation of ACC oxidase activity in sunflower seedlings under salt stress. Plant Signal Behav. 2018;13:e1473683. doi:10.1080/15592324.2018.1473683.
- Ebrahimi R, Bhatla SC. Effect of sodium chloride levels on growth, water status, uptake, transport and accumulation pattern of sodium and chloride ions in young sunflower plants. Commun Soil Sci Plan. 2011;42(7):815–8. doi:10.1080/00103624.2011.552657.
- Munns R, Tester M. Mechanisms of salinity tolerance. Annu Rev Plant Biol. 2008;59:631–681. doi:10.1146/annurev.arplant.59.032607.092911.
- Yeo A. Salinity in Plant solute transport. In: Yeo A, Flowers T, editors. Plant Solute Transport. London: Blackwell Publishing Ltd; 2007. p. 340–356.
- Katerji N, Hoorn VJW, Hamdy A, Mastrorilli M. Salt tolerance classification of crops according to soil salinity and to water stress index. Agr Water Manage. 2000;43:99–109. doi:10.1016/S0378-3774(99)00048-7.
- White PJ, Broadly MR. Chloride in soil and its uptake and movement within the plant: a review. Ann Bot. 2001;88:967–988. doi:10.1006/anbo.2001.1540.
- Ebrahimi R, Bhatla SC. Ion distribution measured by electron probe X-ray microanalysis in apoplast and symplastic pathways in root cells in sunflower plants grown in saline condition. J Biosci. 2012;37:713–721. doi:10.1007/s12038-012.9246-y.
- Luo Q, Yu B, Liu Y. Differential sensitivity to chloride and sodium ions in seedlings of Glycine max and S. soja under NaCl stress. J Plant Physiol. 2005;162:1003–1012. doi:10.1016/j.jplph.2004.11.008.
- Mengel K, Kirby EA. Principles of plant nutrition. Dordrecht (Netherlands): Kluwer Academic Publisher; 2001.
- Wahome PK. Mechanisms of salt tolerance (NaCl) stress tolerance in horticultural crops. A minireview. Acta Hortic. 2003;609:127–131. doi:10.17660/ActaHortic.2003.609.16.
- Tavakkoli E. High concentrations of Na+ and Cl− ions in soil solution have simultaneous detrimental effects on growth of faba bean under salinity stress. J Exp Bot. 2010;61:4449–4459. doi:10.1093/jxb/erq251.
- Tester M, Davenport R. Na+ tolerance and Na+ transport in higher plants. Ann Bot. 2003;91:503–527. doi:10.1093/aob/mcg058.
- Sairam RK, Tyagi A, Chinnusamy W. Salinity tolerance: cellular mechanisms and gene regulation. In: Huang B, editor. Plant-environment interactions. Boca Raton (Fl): CRC Press; 2006. p. 122–136.
- Fageria NK. The use of nutrients in crop plants. Boca Raton, Florida: CRC Press; 2009.
- David A, Yadav S, Bhatla SC. Sodium chloride stress induces nitric oxide accumulation in root tips and oil surface accompanying slower oleosin degradation in sunflower seedlings. Physiol Plant. 2010;140:342–354. doi:10.111/j.1399.3054.2010.01408.x.
- Mukherjee S, Bhatla SC. A novel fluorescence imaging approach to monitor salt stress- induced modulation of oubain- sensitive ATPase activity in sunflower seedling roots. Physiol Plant. 2014;150:540–549. doi:10.1111/ppl.12101.
- Mukherjee S, David A, Yadav S, Baluska F, Bhatla SC. Salt stress- induced seedling growth inhibition coincides with differential distribution of serotonin and melatonin in sunflower seedling roots and cotyledons. Physiol Plant. 2014;152:714–728. doi:10.1111/ppl.12218.
- Frandsen GI, Mundy J, Tzen JTC. Oil bodies and their associated proteins, oleosins and caleosins. Physiol Plant. 2003;112:301–307. doi:10.1034/j.1399-3054.2001.1120301.x.
- Bhatla SC, Vandana S, Kaushik V. Recent developments in the localization of oil body associated signaling molecules during lipolysis in oilseeds. Plant Signal Behav. 2009;4(3):176–182. PMCID: PMC2652523, PMID: 19721744. doi:10.4161/psb.4.3.7799.
- Sadeghipour HR, Bhatla SC. Differential sensitivity of oleosins to proteolysis during oil body mobilization in sunflower seedlings. Plant Cell Physiol. 2002;43:1117–1126. doi:10.1093/pcp/pcf142.
- Sadeghipour HR, Bhatla SC. Light-enhanced oil body mobilization in sunflower seedlings accompanies faster protease action on oleosins. Plant Physiol Biochem. 2003;41(4):309–316. doi:10.1016/S0981-9428(03)00024-X.
- Vandana S, Bhatla SC. Evidence for the probable oil body association of a thiol protease, leading to oleosin degradation in sunflower seedling cotyledons. Plant Physiol Biochem. 2006;44:714–723. doi:10.1016/j.plaphy.2006.09.022.
- Yadav MK, Bhatla SC. Localization of lipoxygenase activity on the oil bodies and protoplasts using a novel fluorescence imaging method. Plant Physiol Biochem. 2010:1–5. doi:10.1016/j.plaphy.2010.11.012.
- Pahoja VM, Sethar MA. Review of enzymatic properties of lipase in plants, animals and microorganisms. J Appl Sci. 2002;2(4):474–484. doi:10.3923/jas.2002.474.484.
- Bhatla SC, Lal MA. Plant physiology, development and metabolism. Springer Nature Singapore Pte. Ltd; 2018. ISBN 978-981-13-2022-4.
- Kaur H, Mukherjee S, Baluska F, Bhatla SC. Regulatory roles of serotonin and melatonin in abiotic stress tolerance in plants. Plant Signal Behav. 2015;10:1–8. PMID: 26633566, PMCID: PMC4883943. doi:10.1080/15592324.2014.1003752.
- Byeon Y, Back K. Melatonin synthesis in rice seedlings in vivo is enhanced at high temperature and under dark conditions due to increased serotonin N-acetyltransferase and N-acetylserotonin methyltransferase activities. J Pineal Res. 2014;56:189–195. doi:10.111/jpi.12111.
- Park S, Byeon Y, Back K. Functional analysis of three ASMT gene family members in rice plants. J Pineal Res. 2013;55:409–415. doi:10.1111/jpi.12088.
- Arnao MB, Hernandez-Ruiz J. Melatonin: plant growth regulator and/or bio stimulator during stress? Trends Plant Sci. 2014;19:789–797. doi:10.1016/j.tplants.2014.07.006.
- Shi H, Ye T, Chen Z. Comparative proteomic responses of two bermudagrass (Cynodon dactylon (L.) Pers.) varieties contrasting in drought stress resistance. J Exp Bot. 2014;82:218–228. doi:10.1093/jxb/eru373.
- Kaur H, Bhatla SC. Melatonin and nitric oxide modulate glutathione content and glutathione reductase activity in sunflower seedling cotyledons accompanying salt stress. Nitric Oxide. 2016;59:42–53. doi:10.1016/j.niox.2016.07.001.
- Foyer CH, Noctor G. Redox regulation in photosynthetic organisms: signaling, acclimation, and practical implications. Antioxid. Redox Signal. 2009;11(4):861–905. doi:10.1089/ars.2018.2177.
- Gill SS, Tuteja N. Reactive oxygen species and antioxidant machinery in abiotic stress tolerance in crop plants. Plant Physiol Biochem. 2010;48:909–930. doi:10.1016/j.plaphy.2010.08.016.
- Mittler R, Vanderauwera S, Gollery M, Breusegem FV. Reactive oxygen gene network of plants. Trends Plant Sci. 2004;9:490–498. doi:10.1016/j.tplants.2004.08.009.
- Hernandez JA, Jimenez A, Mullineaux P, Sevilla F. Tolerance of pea (Pisum sativum L.) to long- term salt stress is associated with the induction of antioxidant defences. Plant Cell Environ. 2000;23:853–862. doi:10.1046/j.1365-3040.2000.00602.x.
- Karray- Bouraoui N, Harbaoui F, Rabhi M, Jallali I, Ksouri R, Attia H, Msilini N, Lachaal M. Different antioxidant responses to salt stress in two different provenances of Carthamus tinctorius L. Acta Physiol Plant. 2011;33:1435–1444. doi:10.1007/S11738-010-0679-3.
- Tanou G, Molassiotis A, Diamantidis G. Induction of reactive oxygen species and necrotic death- like destruction in strawberry leaves by salinity. Environ Exp Bot. 2009;65:270–281. doi:10.1016/j.envexpbot.2008.09.005.
- Meloni DA, Olivia MA, Carlos AM, Cambraia J. Photosynthesis and activity of superoxide dismutase, peroxidase and glutathione reductase in cotton under salt stress. Environ Exp Bot. 2003;49:69–76. doi:10.1016/S0098-8472(02)00058-8.
- Wang B, Luttge U, Ratajezak R. Specific regulation of SOD isoforms by NaCl and osmotic stress in leaves of C3 halophyte Suaeda salsa L. J Plant Physiol. 2004;161:285–293. doi:10.1078/0176-1617-0123.
- Arora D, Bhatla SC. Nitric oxide triggers a concentration- dependent differential modulation of superoxide dismutase (FeSOD and Cu/ZnSOD) activity in sunflower seedling roots and cotyledons as an early and long distance signaling response to NaCl stress. Plant Signal Behav. 2015;10:E1071753 1–10. doi:10.1080/15592324.2015.1071753.
- Herbetter S, Roeckel- Drevet P, Dervet JR. Seleno- independent glutathione peroxidases more than simple antioxidant scavengers. Febs J. 2007;274:2163–2180. doi:10.111/j.1742-4658.2007.65774.x.
- Jain P, Bhatla SC. Signaling role of phospholipid hyderoperoxide glutathione peroxidase (PHGPX) accompanying sensing of NaCl stress in etiolated sunflower seedling cotyledons. Plant Signal Behav. 2014;9(12):e977746 1–7. doi:10.4161/15592324.2014.977746.
- Shen Z, Jiang M, Li H, Che LL, Yang ZM. Expression of Brassica napus heme oxygenase confers plant tolerance to mercury toxicity. Plant Cell Environ. 2011;34:752–763. doi:10.1111/j.1365-3040.2011.02279.x.
- Zhu K, Jin Q, Shamma MK, Lin G, Shen WB. Molecular cloning and characterization of a heme oxygenase1 gene from sunflower and its expression profiles in salinity acclimation. Mol Biol Rep. 2014;41:4109–4121. doi:10.1007/s11033-014-3281-8.
- Xie X, Cui W, Yuan X, Shen WB, Yang Q. Heme oxygenase- 1 is associated with wheat salinity acclimation by modulating reactive oxygen species homeostasis. J Integr Plant Biol. 2011;53:653–670. doi:10.1111/j.1744-7909.2011.01052.x.
- Singh N, Bhatla SC. Nitric oxide and iron modulate heme oxygenase activity as a long distance signaling response to salt stress in sunflower seedling cotyledons. Nitric Oxide. 2016;53:54–64. doi:10.1016/j.niox.2016.01.003.
- Hummel SG, Fischer AJ, Martin SM, Schafer FQ, Buettner GR. Nitric oxide as a cellular antioxidant: a little goes a long way. Free Radic Biol Med. 2006;40:501–506. doi:10.1016/j.freeradbiomed.2005.08.047.
- David A, Yadav S, Baluska F, Bhatla SC. Nitric oxide accumulation and protein tyrosine nitration as a rapid and long distance signalling response to salt stress in sunflower seedlings. Nitric Oxide. 2015;50:28–37. doi:10.1016/j.niox.2015.08.003.
- Astier J, Lindermayr C. Nitric oxide- dependent post- translational modification in plants: an update. Int J Mol Sci. 2012;13:15193–15208. doi:10.3390/ijms1311115193.
- Jain P, Toerne C, Lindermayr C, Bhatla SC. S-nitrosylation/denitrosylation as a regulatory mechanism of salt stress sensing in sunflower seedlings. Physiol Plant. 2018;162:49–72. doi:10.111/ppl.12641.
- Ischiropoulos H. Biological selectivity and functional aspects of protein tyrosine nitration. Biochem Biophys Res Commun. 2003;30:776–783. doi:10.1016/s0006-291x(03)00814-3.
- Singh N, Kaur H, Yadav S, Bhatla SC. Does N- nitrosylation compete with S- nitrosothiols as a long distance nitric oxide carrier in plants? Biochem Anal Biochem. 2016;5:1. doi:10.4172/2161-1009.1000262.
- Arora D, Bhatla SC. Melatonin and nitric oxide regulate sunflower seedling growth under salt stress accompanying differential expression of Cu/Zn SOD and Mn SOD. Free Radic Biol Med. 2017;106:315–328. doi:10.1016/j.freeradbiomed.2017.02.042.
- Kumari A, Kapoor R, Bhatla SC. Nitric oxide and light co-regulate Glycine betaine homoestasis in sunflower seedling cotyledons by modulating betaine aldehyde dehydrogenase transcript levels and activity. Plant Signal Behav. 2019; In Press;229:1641–1648. doi:10.1080/15592324.2019.1666656.
- Tailor A, Tandon R, Bhatla SC. Nitric oxide modulates polyamine biosynthesis in sunflower seedling cotyledons under salt stress. Plant Signal Behav. 2019; In Press;229:1641–1648. doi:10.1080/15592324.2019.1667730.
- Makavitskaya M, Svistunenko D, Navasesky I, Hryvusevich P, Mackievic V, Rabadanova C, Tyutereva E, Samokina V, Straltsova D, Sokolik A, et al. Novel roles of ascorbate in plants: induction of cytosolic Ca2+ signals and efflux from cells via anion channels. J Exp Bot. 2018;69(14):3477–3489. doi:10.1093/jxb/ery056.
- Singh N. Bhatla SC and Demidchik V. Plants and human beings engage similar molecular crosstalk with nitric oxide under stress conditions. Funct Plant Biol. 2019;46:695. doi:10.1071/FP1908.