ABSTRACT
This study was conducted to investigate the involvement of antifreeze proteins (AFPs; type I and III) in the germination mechanism of tomato seeds under low temperature stress. Germination of the seeds grown at a room temperature (25°C) was observed on 5 days after sowing (DAS), while all seeds exposed to a low temperature started to germinate at 16 days after sowing (DAS). However, in comparison with control seeds (0 µg/l), seeds treated with AFP I (100, 300, or 500 µg/l) germinated earlier and at a higher percentage until 20 DAS, and seeds treated with 100 µg/l AFP I showed the highest percentage of germination. Surprisingly, AFP III did not significantly increase germination, and the rate was lower among 500 µg/l AFP III-treated seeds compared with control seeds (0 µg/l). The transcription levels of the plasma membrane-associated H+-ATPase gene and antioxidant-related superoxide dismutase (SOD) and catalase 1 (CAT1) genes were analyzed, and the transcription levels of the genes in the seeds grown at 25°C were relatively low. For low temperature-treated seeds, H+-ATPase in control seeds (0 µg/l) was higher compared with that in AFP I-treated seeds and was lower compared with that in AFP III-treated seeds. The expression levels of the antioxidant-related genes (SOD and CAT1) were lower in AFP I-treated seeds than in control seeds (0 µg/l); however, they were higher in AFP III-treated seeds than in control seeds (0 µg/l). Overall, compared with AFP III, AFP I may potentially function as a cold-protective agent by modulating the genes associated with seed germination.
Introduction
Temperature controls the seed germination of various plant species including Pittosporum, Salicornia rubra, and tomato.Citation1–Citation4 In Pittosporum, the exposure of seeds to low temperatures (4°C) promotes germination,Citation4 while seeds of purple cauliflower was well germinated at the low temperature 10°C.Citation5 On the other hand, lower temperatures (5–15°C) inhibit the seed germination of S. rubra.Citation2 As tomato is a cold-sensitive crop, the temperature for seed germination is around 21–27°C, and temperatures below 10°C would inhibit and slow germination. MobayenCitation3 reported that the minimum and optimum temperature for tomato seed germination is around 11°C and 25°C, respectively. Similarly, Foolad and LinCitation6 observed that cold stress could result in the inhibition of tomato seed germination.
Plasma membrane H+-ATPase, which transports H+ ions from the cytoplasm to cell walls, plays a key role in seed germination, particularly in the beginning of the elongation of seed embryo.Citation7,Citation8 In addition, H+-ATPase adjusts the ionic balance to protect the plant membrane from injury due to low temperatures.Citation9,Citation10 The generation of reactive oxygen species (ROS) occurs in various cellular compartments, particularly those in stressed cells,Citation11 and it affects various aspects of seed physiology.Citation12 However, the excess production of ROS due to abiotic stress may lead to oxidative stress and cellular damage, resulting in seed deterioration.Citation13 Major antioxidant enzymes such as superoxide dismutase (SOD) and catalase (CAT) minimize oxidative stress by scavenging ROS in cells.Citation14 SOD catalyzes the dismutation of O2 – to H2O2, and CAT reduces H2O2 to H2O. The positive roles of antioxidant enzymes in scavenging ROS under various stress conditions have been reported in several plant species.Citation15–Citation17 The transcriptional variation of SOD and CAT under various abiotic stress conditions has also been reported.Citation18–Citation20
Recently, antifreeze proteins (AFPs) have been used as a cold-resistant agent in some plant species.Citation21–Citation23 Jeon et al.Citation21 and Seo et al.Citation22 found that AFP type III promoted the cryopreservation efficiency of chrysanthemum and potato, and Pe et al.Citation23 recently observed the transcriptional variation of cold-responsive genes in plants treated with AFPs (type I and type III). Tomato is a cold-sensitive crop, and a minimum temperature above 11°C is required for seed germination. Hence, it would be interesting to investigate the involvement of AFPs in the germination mechanism of tomato seeds under low temperature stress.
In this study, we investigated the effect of AFPs on the germination of tomato seeds exposed to a series of the temperature (4°C) for 5 days (d), followed by 10°C and 20°C for 7 d and 8 d respectively, by determining the percentage of germination and expression levels of the genes involved in the germination process.
Materials and methods
Materials
Seeds of the commercial tomato cultivar ‘Dotaerang Plus’ (Takii Korea Co., Ltd., Seoul, Korea) were used for this study.
AFP treatment
Before the seeds were sown into the soil, they were pre-treated with two different AFPs (type I and type III) derived from fish (A/F Protein Inc.,Waltham, MA, USA) by immersing in water containing different concentrations (100, 300, and 500 µg/l) of the AFPs for 24 h. The seeds immersed in the water without AFPs (0 µg/l) were used as the control. The difference in structure between AFP type I and type III was also described in .
Figure 1. Difference in structure between antifreeze proteins type I and type III. This figure is copyrighted by CapicciottiCitation24
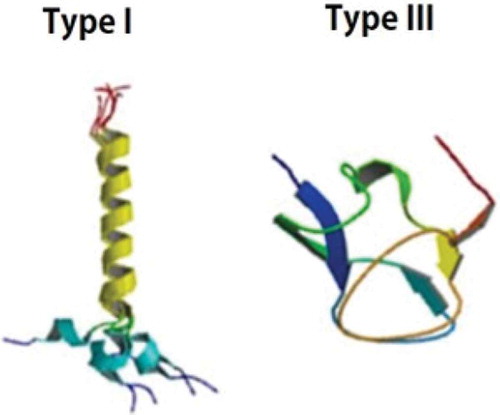
Low temperature stress and germination assessment
After immersion in water with or without AFPs for 24 h, the seeds were sown in seedling trays filled with the soil-less mixture BM7 (Berger Co., Quebec, Canada) in a growth chamber at 4°C with a 16 h photoperiod and 70% relative humidity for 5 d. Subsequently, the seeds were allowed to grow at 10°C for 7 d, followed by 20°C for 8 d. For positive control, the seeds soaked only in water were allowed to grow at a room temperature (25°C).There were 30 seeds per tray with three replications.
The germination percentage was recorded for all treatments from the first day the seedling emerged, and the percentage was recorded three times with two-day intervals.
Transcriptional analysis of genes associated with seed germination by qrt-pcr
RNA was extracted from 16-day-old seedlings using the RNAqueous kit (Ambion Inc., Austin, TX, USA). ReverTra Ace-α (Toyobo Co., Ltd., Osaka, Japan) was used to synthesize cDNA from 1 µg of total RNA. The transcription level of tomato plasma membrane-associated H+-ATPase gene and tomato antioxidant-related SOD and CAT1 genes was quantified using the StepOnePlus Real-Time PCR System (Thermo Fisher Scientific, Inc., Waltham, MA, USA). Tomato actin gene was used as a reference gene for normalization of the gene expression level. The primers and PCR conditions used in this study are listed in the supplementary table 1. The transcriptional analysis was performed from three different biological samples.
Data analysis
Data were analyzed using SPSS v.11.09 (IBM Corporation, Armonk, NY, USA). The data represent the means of three replications, and they were statistically analyzed using Duncan’s multiple range test (P < .05).
Results
Effect of afps on seed germination
The germination of tomato seeds started on day 5 under normal growth conditions (25°C) (data not shown); however, germination was not observed for seeds that were soaked with different concentrations of AFPs for 24 h and exposed to a low temperature (4°C) for 5 d. The seeds did not germinate even after they were incubated at 10°C for 7 d. Their germination started at 4 d after incubation at 20°C. Therefore, the germination of seeds was not observed for all treatments at 4°C and 10°C, and seed germination started at 16 days after sowing (DAS) at 20°C, with the maximum percentage of seed germination at 20 DAS. During the germination period, the rate of germination of the control seeds (0 µg/l) was slower and lower compared with that of the AFP I-treated seeds, and size of the seedlings germinated from control (0 µg/l) was smaller than that of the AFP I-treated seeds ().
Figure 2. Comparison of the size of seedlings germinated from tomato seeds treated with AFP I (100, 300, and 500 µg/l) and control (0 µg/l).
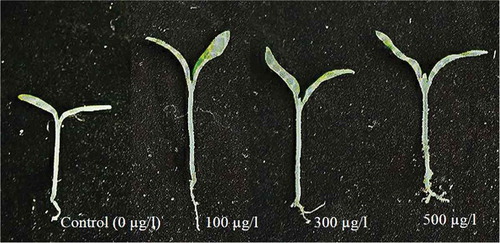
However, AFP III had a negative effect on seed germination. Although AFP I induced a higher percentage of germination (), the percentage was slightly different depending on the concentration used; specifically, the highest percentage was observed with 100 µg/l AFP I. In the case of AFP III, 100 and 300 µg/l were more likely to increase germination until 18 DAS; however, the percentage of germination was significantly higher among control seeds (0 µg/l) compared with AFP III-treated seeds at 20 DAS. Notably, AFP III (500 µg/l) negatively affected the germination of seeds throughout the period ().
Figure 3. Comparison of germination percentages of tomato seeds treated with or without antifreeze proteins type I (a) and type III (b) under the growing condition (at 4°C for 5 d, followed by 10°C for 7 d and 20°C for 8 d. Control indicates the seeds soaked only in water. Data represent means of the replications, and bars indicate errors of three replications.
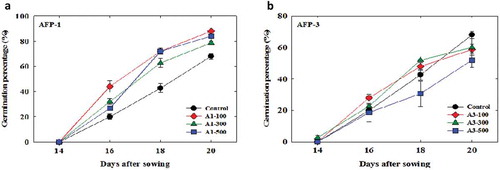
Transcriptional analysis of genes involved in seed germination
The seeds grown at 25°C germinated at 5 DAS. However, under low temperature condition, variation in the germination percentage was observed among tomato seeds treated with different concentrations and types of AFPs, as described in the previous section. To clarify the association between germination percentage and gene expression and to determine how AFPs affect the expression of the genes, we quantified the expression of the H+-ATPase, SOD, and CAT1 genes in the germinated seedlings by qRT-PCR. The transcription levels of the genes in the seedlings germinated from normal growing condition (25°C) were significantly lower than those of the seedlings derived from the seeds exposed to low temperature ( and ). In addition, the transcript levels of the genes in the seedlings derived from the seeds exposed to low temperature significantly varied depending on different concentrations and types of AFPs treated. Specifically, the transcript level of H+-ATPase in the seedlings germinated from control seeds (0 µg/l) was upregulated and was significantly higher compared with those germinated from100 and 300 µg/l AFP I-treated seeds and slightly higher compared with those germinated from 500 µg/l AFP I-treated seeds (). In contrast, the expression of H+-ATPase was lower in control (0 µg/l) than in AFP III treatments; however, it was not significantly different ().
Figure 4. Transcript levels of Plasma membrane H+-ATPase in seedlings germinated from the seeds grown in the normal growing condition (at 25°C) and those treated with or without antifreeze proteins type I (a) and type III (b) under low temperature condition (at 4°C for 5 d, followed by 10°C for 7 d and 20°C for 4 d. Control indicates the seeds soaked only in water and exposed to low temperature, and 25°C indicates the seeds soaked only in water and grown in the normal growing condition (25°C) for 16 days. Data represent means of three biological replicates, and bars indicate errors of three biological replicates.
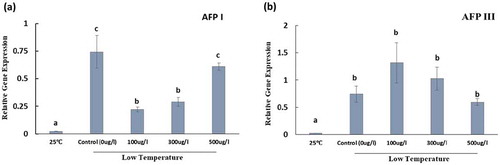
Figure 5. Transcript level of antioxidant-related genes SOD (a and b) and CAT1 (c and d) expressed in seedlings germinated from the seeds grown in the normal growing condition (at 25°C) and those treated with or without antifreeze proteins type I and III under the low temperature condition (at 4°C for 5 d, followed by 10°C for 7 d and 20°C for 4 d. Control indicates the seeds soaked only in water and exposed to low temperature, and 25°C indicates the seeds soaked only in water and grown in the normal growing condition (25°C) for 16 days. Data represent means of the biological replicates, and bars indicate errors of three biological replicates.
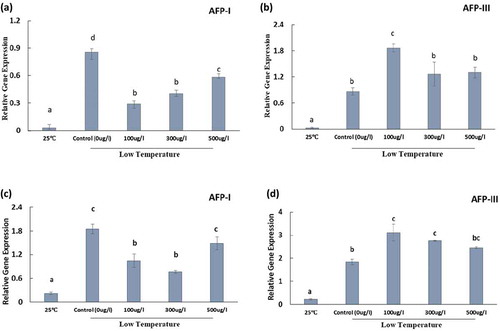
The expression patterns of SOD and CAT1 in control (0 µg/l) and AFP I treatments were similar to those of H+-ATPase, and their expression levels were higher in control (0 µg/l) than in AFP treatments (specifically 100 and 300 µg/l) ( a, c). Similar to H+-ATPase, the expression levels of SOD and CAT1 were higher in AFP III treatments than in control (0 µg/l), with significantly higher levels in 100 µg/l AFP III ( b, d).
Discussion
The optimum temperature conditions required for seed germination varies depending on the plant species. For example, the seeds of Pittosporum species are well germinated when exposed to low temperatures (4°C),Citation4 while 10°C was optimal for seed germination of purple cauliflower.Citation5 On the other hand, lower temperatures (5–15°C) significantly inhibited the seed germination of S. rubra.Citation2 Tomato seeds require higher temperatures (11–25°C) for germination due to cold sensitivity,Citation3 and the inhibition of seed germination has been observed during cold stress.Citation6 Recently, the cold-protective role of AFPs in some plant species has been reported.Citation21–Citation23 Therefore, we investigated the role of AFPs in the germination of tomato seeds exposed to a low temperature (4°C) for 5 d, followed by 10°C for 7 d and 20°C for 8 d.
In this study, although the tomato seeds were well germinated at 5 DAS under normal growth conditions, germination was not observed for seeds treated with or without AFPs at 4°C. Surprisingly, they failed to germinate even after transfer from 4°C to 10°C for 7 d. Mitosis may be inhibited by the low temperature.Citation25 The failure of germination may be attributed to the inactivation of mitotic cell division, which is vital for successful seed germination and early seedling growth.Citation26 Similarly, the severe effect of low temperatures on the germination of other Solanaceae crops such as eggplant, pepper, and tomato has been reported.Citation27 Seed germination started on day 4 (16 DAS) after transfer to 20°C and was continuously increased until 20 DAS. The percentage of germination was significantly lower in control seeds (0 µg/l) compared with all seeds treated with AFP (100, 300, or 500 µg/l). The reduced germination of control seeds (0 µg/l) may be attributed to cell injury during low temperature stress, and the higher germination percentage of AFP I-treated seeds may be attributed to the cold-protective role of AFP I, which could prevent cell injury under low temperature stress. The germination starting date of AFP III-treated seeds were also similar to those of AFP I-treated seeds; however, the germination percentage of AFP III-treated seeds was not significantly different from that of control seeds (0 µg/l) and was even lower than that of control seeds (0 µg/l) at 20 DAS. Unlike AFP I, AFP III may not protect cells from cold injury and may exert toxic effects on the seeds, resulting in lower germination percentages depending on the concentration in the following order: 500 < 100 < 300 < 0 µg/l. Based on the results, we found that the germination percentage was dependent on the types of AFPs used. Furthermore, although AFP III has been used as a cryoprotectant for the cryopreservation of some plant species, its adverse effect in this study may be explained by the different plant species used. The different effects of AFPs depending on the plant species and the adverse effects of AFP III have been reported in previous studies.Citation28 In addition, the more effective utilization of AFP I compared with AFP III in cryopreservation has been reported.Citation28
We found that the effect of AFPs on seed germination was associated with the transcription levels of the plasma membrane-associated H+-ATPase gene, which plays a key role in seed germination.Citation7,Citation8 Its transcription level was lower in the seedlings germinated from AFP I-treated seeds than in the seedlings germinated from untreated control seeds (0 µg/l). It is possible that the transcription of H+-ATPase was strongly induced in control (0 µg/l) to protect the cell membrane from damage under cold stress; however, transcription was moderately induced in AFP I treatments owing to the cold-protective role of AFP I. Although the results were not significant, transcription levels were higher in seedlings germinated from seeds treated with AFP III than in control (0 µg/l). It is likely that seeds treated with AFP III may have been more negatively affected during cold stress due to the potential toxic effect of AFP III, resulting in the high transcription levels of H+-ATPase in AFP III treatments. This may also explain the higher germination rate in control seeds (0 µg/l). Under normal growing condition (25°C), it seemed that the seeds did not need to trigger the expression of H+-ATPase for germination, thus, its expression was the lowest in the seedlings. Recently, the role of H+-ATPase in modulating the ionic balance to protect the plant membrane from injury caused by low temperatures has been identified.Citation9–Citation11
The upregulation of ROS in plant cells by various abiotic stresses has been reported,Citation11 and the role of major antioxidant enzymes such as SOD and CAT in minimizing oxidative stress by scavenging ROS in plant cells has also been reported.Citation14–Citation17 Moreover, the transcriptional variation of SOD and CAT under various abiotic stress conditions has been demonstrated.Citation18–Citation20 In this study, the transcription levels of SOD and CAT1 were found to be associated with the germination rate, and expression levels were higher in the seedlings germinated from control seeds than in AFP I treatments. Antioxidant-related enzymes can scavenge ROS generated under cold stress and minimize oxidative stress to promote seed germination.Citation12,Citation13 In this study, it is likely that AFP I reduced the injury of seeds caused by cold stress, whereby the seedlings derived from the AFP I-treated seeds did not strongly induce the transcription of the genes. In contrast to AFP I, AFP III may negatively affect the physiology of the seeds during cold stress because the transcription of the genes is strongly induced in the seedlings germinated from AFP III-treated seeds. The transcriptional variation of genes following AFP treatment under cold stress has also been reported recently.Citation23 The lowest expression of the genes in the seedling derived from normal growing condition could be explained that the seedlings did not need high antioxidant contents to scavenge ROS as they were not grown under low temperature condition. Taken together, the results revealed how cold stress may affect tomato seed germination and highlighted the potential of AFP I as a cold-protective agent in tomato seed germination under cold stress.
Conclusion
We demonstrated the sensitivity of tomato seed germination to cold stress. In addition, the potential of AFP I as a cold-protective agent in the seed germination of tomato was determined. However, AFP III may have an adverse effect considering the lower germination percentage and increased expression of H+-ATPase, SOD, and CAT1. This study demonstrated that AFP I may be used as a cold-protective agent in the seed germination of cold-sensitive crops under cold stress, and the results could contribute to a better understanding of how AFPs may reduce cold injury during tomato seed germination. To our knowledge, this is the first study to describe the positive role of AFP I in seed germination under cold stress.
Disclosure of Potential Conflicts of Interest
No potential conflicts of interest were disclosed.
Supplemental Material
Download MS Word (12.7 KB)Acknowledgments
This work was supported by the Korea Institute of Planning and Evaluation for Technology in Food, Agriculture, Forestry and Fisheries (IPET) through the Agri-Bio industry Technology Development Program, funded by the Ministry of Agriculture, Food and Rural Affairs (MAFRA) (Grant No. 315002-5).
Supplemental material
Supplemental data for this article can be accessed on the publisher’s website.
References
- Bewley JD, Black M. Physiology and biochemistry of seeds in relation to germination. Berlin, Germany: Springer-Verlag; 1982. Vol. 2. p. 1.
- Khan MA, Gul B, Weber DJ. Germination responses of Salicornia rubra to temperature and salinity. J Arid Environ. 2000;45:207–6. doi:10.1006/jare.2000.0640.
- Mobayen RG. Germination and emergence of citrus and tomato seeds in relation to temperature. J Hortic Sci. 1980;55:291–297. doi:10.1080/00221589.1980.11514937.
- Moore S, Bannister P, Jameson P. The effect of low temperatures on seed germination of some New Zealand species of Pittosporum. New Zeal J Bot. 1994;32:483–485. doi:10.1080/0028825X.1994.10412934.
- Wartidiningsih N, Geneve RL, Kester ST. Osmotic priming or chilling stratification improves seed germination of purple coneflower. HortScience. 1994;29:1445–1448. doi:10.21273/HORTSCI.29.12.1445.
- Foolad MR, Lin GY. Relationship between cold tolerance during seed germination and vegetative growth in tomato: germplasm evaluation. J Am Soc Hortic Sci1. 2000;25:679–683. doi:10.21273/JASHS.125.6.679.
- Hager A. Role of plasma membrane H+-ATPase in auxin-induced elongation growth: historical and new aspects. J Plant Res. 2003;116:483–505. doi:10.1007/s10265-003-0110-x.
- Buch-Pedersen MJ, Pedersen BP, Veierskov B, Nissen P, Palmgren MG. Protons and how they are transported by proton pumps. Eur J Physiol. 2009;457:573–579. doi:10.1007/s00424-008-0503-8.
- Muzi C, Camoni L, Visconti S, Aducci P. Cold stress affects Hþ-ATPase and phospholipase D activity in Arabidopsis. Plant Physiol Bioch. 2016;108:328–336. doi:10.1016/j.plaphy.2016.07.027.
- Małgorzata JR, Katarzyna K, Anna W, Grazyna K. Response of plasma membrane H+ -ATPase to low temperature in cucumber roots. J Plant Res. 2012;125:291–300. doi:10.1007/s10265-011-0438-6.
- Scandalios JG. Oxidative stress: molecular perception and transduction of signals triggering antioxidant gene defenses. Braz J Med Biol Res. 2005;38:995–1014. doi:10.1590/S0100-879X2005000700003.
- Bailly C. Active oxygen species and antioxidants in seed biology. Seed Sci Res. 2004;14:93–107. doi:10.1079/SSR2004159.
- Bailly C, El-Maarouf-Bouteau H, Corbineau F. From intracellular signaling networks to cell death: the dual role of reactive oxygen species in seed physiology. C R Biol. 2008;331:806–814. doi:10.1016/j.crvi.2008.07.022.
- Mittler R, Vanderauwera S, Gollery M, Van Breusegem F. Reactive oxygen gene network of plants. Trends Plant Sci. 2004;9:490–498. doi:10.1016/j.tplants.2004.08.009.
- Murshed R, Lopez-Lauri F, Sallanon H. Effect of salt stress on tomato fruit antioxidant systems depends on fruit development stage. Physiol Mol Biol Plants. 2014;20:15–29. doi:10.1007/s12298-013-0209-z.
- Wu CA, Yang GD, Meng QW, Zheng CC. The cotton GhNHX1 gene encoding a novel putative tonoplast Na+/H+ antiporter plays an important role in salt stress. Plant Cell Physiol. 2004;45:600–607. doi:10.1093/pcp/pch071.
- Xu SC, Li YP, Hu J, Guan YJ. Responses of antioxidant enzymes to chilling stress in tobacco seedlings. Agri Sci China. 2010;9:1594–1601. doi:10.1016/S1671-2927(09)60256-X.
- Badawi GH, Yamauchi Y, Shimada E, Sasaki R, Kawano N, Tanaka K, Tanaka K. Enhanced tolerance to salt stress and water deficit by overexpressing superoxide dismutase in tobacco (Nicotiana tabacum) chloroplasts. Plant Sci. 2004;166:919–928. doi:10.1016/j.plantsci.2003.12.007.
- Sen Gupta A, Webb RP, Holaday AS, Allen RD. Overexpression of superoxide dismutase protects plants oxidative stress. Plant Physiol. 1993;103:1067–1073. doi:10.1104/pp.103.4.1067.
- Polidoros AN, Mylone PV, Scandalios JG. Transgenic tobacco plants expressing the maize Cat2 gene have altered catalase levels that affect plant–pathogen interactions and resistance to oxidative stress. Transgenic Res. 2001;10:555–569. doi:10.1023/A:1013027920444.
- Jeon SM, Naing AH, Park KI, Kim CK. The effect of antifreeze protein on the cryopreservation of chrysanthemums. Plant Cell Tiss Org. 2015;123:665–671. doi:10.1007/s11240-015-0852-x.
- Seo JH, Naing AH, Jeon SM, Kim CK. Anti-freezing-protein type III strongly influences the expression of relevant genes in cryopreserved potato shoot tips. Plant Mol Biol. 2018;97:347–355. doi:10.1007/s11103-018-0743-8.
- Pe PPW, Naing AH, Chung MY, Park KI, Kim CK. The role of antifreeze proteins in the regulation of genes involved in the response of Hosta capitata to cold. Biotech. 2019;9:335.
- Capicciotti CJ, Doshi MBen RN. Ice recrystallization inhibitors: from biological antifreezes to small molecules. In: Wilson P, editor. Recent developments in the study of recrystallization. InTech, Rijeka-Croatia; 2013. p. 177–224
- Simon EW, Minchin A, McMenamin MM, Smith JM. The low temperature limit for seed germination. New Phytol. 1976;77:301–311. doi:10.1111/nph.1976.77.issue-2.
- Masubelele NH, Dewitte W, Menges M, Maughan S, Collins C, Huntley R, Nieuwland J, Scofield S, Murray JAH. D-type cyclins activate division in the root apex to promote seed germination in Arabidopsis. Proc Nat Acad Sci, USA. 2005;102:15694–15699. doi:10.1073/pnas.0507581102.
- Wilcox GE, Pfeiffer CL. Temperature effect on seed germination, seedling root development and growth of several vegetables. J Plant Nutr. 1990;13:1393–1403. doi:10.1080/01904169009364161.
- Naing AH, Kim CK. A brief review of applications of antifreeze proteins in cryopreservation and metabolic genetic engineering. Biotech. 2019;9:329.