ABSTRACT
The winter squash (Cucurbita moschata, Cm) superoxide dismutase (SOD) CmSOD gene and Arabidopsis thaliana (At)SOD gene were transferred under a ubiquitin promoter into Arabidopsis via Agrobacterium tumefaciens. The expression and amount of SOD and the SOD activities in the AtSOD and CmSOD transgenic lines were significantly higher than those of non-transgenic (NT) plants exposed to 23 or 4°C treatment for 6 ~ 192-h periods. Furthermore, expressions of the cold-inducible gene (AtCBF2) and desiccation-responsible transcription factors (AtRD29A/B) were also activated in all transgenic lines compared to NT plants after chilling treatments. Compared to NT plants under chilling stress, superoxide (•O2−) accumulation was significantly lower, and chlorophyll (Chl) contents were significantly higher in all transgenic lines with higher SOD activity. Moreover, Arabidopsis seedlings overexpressing AtSOD and CmSOD also displayed greater resistance to chilling and less oxidative injury than NT plants under chilled conditions, indicating that the overexpression of AtSOD and CmSOD in Arabidopsis enhanced chilling tolerance by eliminating •O2−. The expression of AtRD29A was strongly up-regulated only in AtSOD transgenic plants treated with abscisic acid (ABA), while it was repressed in other transgenic plants, indicating ABA-sensitive AtCBF2 and AtRD29A/B transcriptional regulation signaling pathways in transgenic Arabidopsis under chilling conditions.
Introduction
Low temperatures are the primary physiological constraints to plant production in winter. Chilling sensitivity is considered an important issue in plant physiological and biophysical studies, particularly for tropical and subtropical species, which show characteristic damage symptoms when subjected to low but above zero temperatures.Citation1 Winter squash (Cucurbita moschata, Cm) is a creeping, climbing, herbaceous, monoecious and annual plant. It has been used successfully as a cold-hardy rootstock that is resistant to chilling stress for winter production of bitter melon (Momordia charanthia) because bitter melon is generally injured at temperatures below 10°C).Citation2 Low temperatures trigger a wide variety of plant responses, including regulation of gene expressions. Integrated transcriptional networks of stress and energy signaling share parts of their signaling pathways, indicating that the sensing of energy deprivation can widen stress responses.Citation3 Plants sense and cope with environmental stress by activating stress-related genes and metabolites related to signal transduction and stress perception (Huang et al. 2012).Citation4 Knowledge of enzymes responsive to a chilling condition is critical for a further understanding of the molecular mechanisms of stress tolerance.Citation5
The C-repeat-binding factor/dehydration responsive element-binding factor 1 (CBF/DREB1) transcription factor, regulates the promoter containing DRE/CRT (A/GCCGAC) for targeted gene expression under low temperatures.Citation6 Cell membranes of plants are injured under low temperatures, causing the cell membrane fluidity to drop or other changes to occur which induce calcium ions to transmit signals and activate mitogen-activated protein kinase (MAPK) required for cold acclimation.Citation7 Novillo et alCitation8 demonstrated that the CBF2 gene negatively regulates CBF1 and CBF3, and its expression in Arabidopsis promotes resistance to freezing and related stresses. RD29A, also known as low-temperature-responsive protein 78 (LTI78), participates in the cold stress signaling pathway by overexpressing CBF/DREB1, which leads to activation of RD29A expression.Citation9 ABA-responsive elements (ABREs) are cis-acting elements that function in mediating the expression of RD29B (also known as LTI65) of Arabidopsis in response to ABA treatment. Dehydration-responsive elements (DREs) function as coupling elements of ABREs in expressing RD29A.Citation10 TaAREB3 gene expression of Triticum aestivum was induced by ABA and low-temperature stress, and its protein has transcriptional activation activity and can bind to the ABRE cis-element in vitro.Citation11 Overexpression of TaAREB3 in Arabidopsis enhances ABA sensitivity, while also strengthening its drought and freeze tolerance. TaAREB3 also activates RD29A and RD29B by binding to their promoter regions in transgenic Arabidopsis.
Environmental stresses induce the production of reactive oxygen species (ROS).Citation12 These ROS are necessary for inter- and intracellular signaling, but under stress conditions, they seriously disrupt normal metabolism in plants through oxidation of membrane lipids, proteins, and nucleic acids in the absence of protective mechanisms.Citation13 Toxic radicals can be removed by the antioxidant system to protect plant cells against oxygen toxicity and counter the hazardous effects of ROS. The increased activity of some antioxidant enzymes are part of the defense mechanism against oxidative stress, in which activities of antioxidant enzymes are often used as a measure of ROS-mediated oxidative stress.Citation12 Antioxidant enzyme levels differentially change in response to environmental constraints, depending on the magnitude of the stress and species-specific sensitivity to the stress. Activities of antioxidant enzymes are correlated with a plant’s tolerance to abiotic stresses.Citation14
Stress tolerance is the result of a complex cascade of molecular events that include gene activation and/or upregulation of stress-induced genes that contribute to stress tolerance.Citation15 Genetic manipulation of antioxidant enzymes is one of the effective measures imparting stress tolerance in plants. The activity of superoxide dismutase (SOD) increases in plants subjected to cold stress and confers cold stress tolerance.Citation16 Increased expression of SOD genes in transgenic potatoes produced protection against methyl viologen-induced oxidative stress.Citation17 In addition, Xi et alCitation18 reported that overexpression of the SOD gene in Arabidopsis enhanced oxidation stress tolerance during germination and early seedling growth. Gill et alCitation19 also illustrated that overexpression of Potentilla atrosanguinea SOD improved salt stress tolerance in Arabidopsis thaliana. Several lines of evidence also demonstrated that SOD expression and activities are activated by salt, drought and osmotic stress in arabidopsis,Citation20 maize,Citation21 halophytes,Citation22 tomato,Citation23 and soybean.Citation24 Transgenic rice plants over-expressing Methanothermobacter thermautotrophicus protein disulfide isomerase increased SOD activity and exhibited stronger resistance to high temperatures with concomitant less oxidative injury.Citation25
Previously, we reported that increased SOD activity provided winter squash plants with increased chilling stress tolerance.Citation26 Increased expression of the SOD gene was accompanied by increased SOD activity which was involved in detoxifying ROS in response to chilling stress.Citation27 The full length of SOD complimentary (c)DNA was then cloned and found to consist of 703 base pairs (bp). Cloning the gene and studying its expression patterns in response to chilling stress provides a molecular basis for improving chilling tolerance in winter squash.Citation26 The objectives of this research were to study how expressions of the AtSOD and CmSOD genes in transgenic lines of Arabidopsis respond to chilling stress during seedling growth. In addition, in order to test whether ABA is involved in transcriptional regulation signaling pathways during chilling treatments, expressions patterns between and among the AtCBF2, AtRD29A, and AtRD29B genes in transgenic Arabidopsis plants in response to both ABA and chilling treatments are also discussed to facilitate our understanding of chilling response mechanisms.
Results
Transformation, regeneration, and identification of transgenic lines
Both transgenic and NT seedlings were selected on MS medium containing 50 mg/L hygromycin for 14 days. NT seedlings were pale and sterile after selection, but shoots of the transgenic seedlings still appeared healthy and green (photos not shown). Seedlings were transplanted into soil for subsequent growth. Transgenic seeds were then collected, oven-dried, and stored at 4°C until use. Transformation efficiencies of hygromycin-resistant AtSOD and CmSOD transgenic plants were 0.14% and 0.18%, respectively (Supplemental Table S1). Five AtSOD and 10 CmSOD healthy, robust, and independent transgenic Arabidopsis lines were generated and designated AtSOD1 ~ 5 and CmSOD1 ~ 10, respectively. These T3 transgenic lines were detected by genomic DNA PCR amplification and electrophoresis. All AtSOD and CmSOD lines respectively displayed the expected bands of the HA-AtSOD, CmSOD, and hygromycin genes, and the respective sizes of the fragments were 716, 600, and 987 bp (Supplemental Figure S2a,b).
Determination of AtSOD, CmSOD, AtCBF2, AtRD29A, and AtRD29B genes expression in transgenic lines
To investigate whether the AtSOD gene from Arabidopsis and the CmSOD gene from winter squash were expressed in transgenic Arabidopsis, a real-time qPCR analysis was performed with extracted RNA from 14-day-old NT, AtSOD1 ~ 5, and CmSOD1 ~ 10 transgenic plants which were subjected to chilling-stress treatment for 0 (control), 6, 72, and 192 h. Data were normalized with respect to the RNA level of AtActin-8, a housekeeping gene that is consistently expressed in plants. , show that different expressions were observed, and RNA abundances of AtSOD and CmSOD transgenic plants were upregulated. The AtSOD gene was constitutively expressed in all transgenic lines, and RNA levels of AtSOD1 ~ 5 (2.63 ~ 6.18 at 0 h, 3.39 ~ 13.31 at 6 h, 4.53 ~ 23.32 at 72 h, and 3.64 ~ 7.44 at 192 h) were significantly higher than those of NT plants (1 at 0 h, 2.30 at 6 h, 2.69 at 72 h, and 1.12 at 192 h) under 23°C (control) or chilling conditions (). Meanwhile, levels of the CmSOD gene of CmSOD1 ~ 10 (0.13 ~ 6.69 at 0 h, 0.23 ~ 4.58 at 6 h, 0.47 ~ 9.16 at 72 h, and 0.31 ~ 5.10 at 192 h) were significantly higher than those of NT (almost no expression) plants under 23°C (control) or chilling conditions ().
Figure 1. Relative RNA expressions of the Arabidopsis thaliana superoxide dismutase (AtSOD) and Cucurbita moschata SOD (CmSOD) genes in non-transgenic (NT) Arabidopsis plants and transgenic Arabidopsis lines under chilled conditions.
A. The AtSOD gene expression in NT plants and T3 homozygote AtSOD1 ~ 5 lines.
B. The CmSOD gene expression in NT plants and T3 homozygote CmSOD1 ~ 10 lines.
Total RNA in all tested plants was extracted from leaves of 2-week-old plants subjected to 4°C for 0 (control), 6, 72, and 192 h of chilling treatment. Relative amounts were calculated and normalized with respect to AtActin-8 RNA. Values are the means of three replicates with the corresponding standard deviation. The gene expression is compaired to NT plants and an asterisk indicates a significance level of p ≤ 0.05.
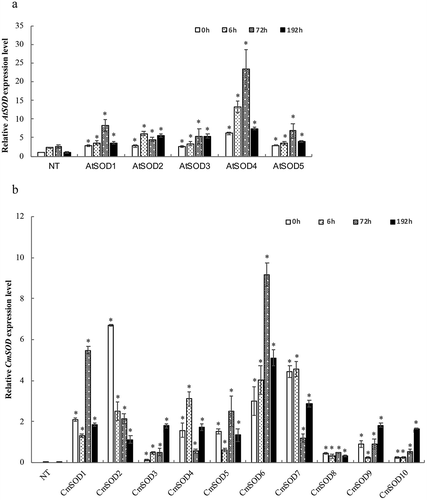
In addition to the AtSOD and CmSOD genes, expressions of transcription factors AtCBF2, AtRD22, AtDREB1A, AtRD29A, and AtRD29B involved in chilling stress response were analyzed. No significant expression differences of AtRD22, AtDREB1A were observed between transgenic lines and NT under chilling stress (data not shown). While AtCBF2, AtRD29A, and AtRD29B genes in the AtSOD and CmSOD transgenic lines with various chilling periods at 0 ~ 192 h are shown in . RNA levels of AtCBF2 (0.37 ~ 9.6, panel A), AtRD29A (0.11 ~ 5.65, panel C), and AtRD29B (0.37 ~ 9.36, panel E) in the AtSOD1 ~ 5 lines were significantly higher than those of NT plants (0.30 ~ 0.63 in panel A, 0.10 ~ 0.45 in pane; C, and 0.22 ~ 1.54 in panel E) after chilling exposure for 0 ~ 192 h. Moreover, all expressions of AtCBF2, AtRD29A, and AtRD29B in the AtSOD1 ~ 5 lines increased from 0 to 72 h but had decreased by 192 h of the chilling period. show that when plants were subjected to 0 ~ 192 h of chilling, expressions of AtCBF2 (0.48 ~ 13.28, panel B), AtRD29A (0.07 ~ 5.08, panel D), and AtRD29B (0.33 ~ 50.9, panel F) in the CmSOD1 ~ 10 lines were also significantly higher those of NT plants (0.09 ~ 1.32 in panel B, 0.11 ~ 0.45 in panel D, and 0.22 ~ 1.73 in panel F). In addition, expressions of AtCBF2 in the CmSOD1 and CmSOD4 ~ 10 lines (panel B), and AtRD29A in the CmSOD1 line (panel D) increased from 0 to 72 h but had decreased by 192 h of the chilling period. However, expressions of AtCBF2, AtRD29A, or AtRD29B in the other CmSOD lines peaked at 6 h then dropped thereafter.
Figure 2. Relative RNA expressions of Arabidopsis thaliana (At)CBF2, AtRD29A, and AtRD29B genes in non-transgenic (NT) Arabidopsis plants and transgenic Arabidopsis lines under chilled conditions.
A. The AtCBF2 gene expression in NT plants and T3 homozygote AtSOD1 ~ 5 lines,
B. The AtCBF2 gene expression in NT plants and T3 homozygote CmSOD1 ~ 10 lines,
C. The AtRD29A gene expression in NT plants and T3 homozygote AtSOD1 ~ 5 lines,
D. The AtRD29A gene expression in NT plants and T3 homozygote CmSOD1 ~ 10 lines,
E. The AtRD29B gene expression in NT plants and T3 homozygote AtSOD1 ~ 5 lines,
F. The AtRD29B gene expression in NT plants and T3 homozygote CmSOD1 ~ 10 lines,
Total RNA in all tested plants was extracted from 2-week-old plants subjected to 4°C for 0 (control), 6, 72, and 192 h of chilling treatment. Relative amounts were calculated and normalized with respect to AtActin-8 RNA. Values are the means of three replicates with the corresponding standard deviation. The gene expression is compaired to NT plants and an asterisk indicates a significance level of p ≤ 0.05.
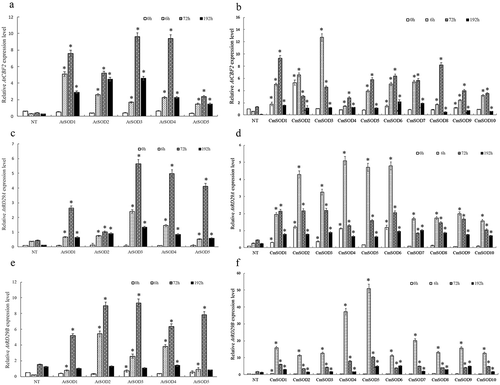
Western blot analysis, SOD activity analysis, and in vivo detection of superoxide in transgenic lines
A Western blot analysis was conducted to estimate relative amounts of the AtSOD and CmSOD proteins that had accumulated in the transgenic Arabidopsis lines, followed by use of antiserum anti-HA to identify AtSOD and CmSOD expressions. A clear band was detected in the AtSOD1 ~ 5 (29 kDa, ) and CmSOD1 ~ 10 lines (24 kDa, ), but such a protein was not detected in NT plants. In addition, the AtSOD3, AtSOD4, CmSOD3, CmSOD6, CmSOD7, and CmSOD8 lines had relatively higher accumulations of the SOD protein than did the other transgenic lines.
Figure 3. Western blot analysis of the Arabidopsis thaliana superoxide dismutase (AtSOD)1 ~ 5 (a) and Cucurbita moschata SOD (CmSOD)1 ~ 10 (b) transgenic lines and comparisons with non-transgenic (NT) plants. Forty micrograms of total proteins was used for each sample. The molecular weights of AtSOD at 29 kDa (a) and CmSOD at 24 kDa (b) are indicated by an arrowhead.
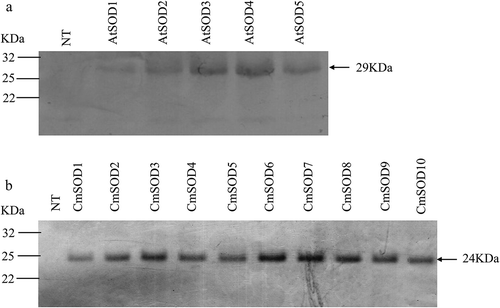
illustrate comparisons of SOD activities in 14-day-old NT and T3 homozygote transgenic plants under non-chilling (0 h of chilling at 23°C, control) treatment and 4°C chilling for 192 h in a cold room. SOD activities of the AtSOD1 ~ 5 lines (43 ~ 75 μmol/g protein) and CmSOD1 ~ 10 lines (37 ~ 81 μmol/g protein) were significantly higher than those of all NT plants (20 ~ 33 μmol/g protein) under control and chilling treatments. Furthermore, all transgenic lines exhibited lower accumulation of superoxide (•O2−) compared to NT plants under chilling treatment for 192 h by in situ ROS staining (), indicating that the transgenic lines had slightly lower ROS accumulation relative to NT plants. Chilling resulted in significantly higher levels of ROS accumulation in NT leaves, whereas the transgenic lines exhibited low accumulation levels as evidenced by the lower intensity of the blue color.
Figure 4. Superoxide dismutase (SOD) activity (a, b) and superoxide (•O2−) accumulation according to in situ reactive oxygen species (ROS) staining (c, d) in leaves of non-transgenic (NT) Arabidopsis plants and T3 homozygote transgenic lines. Arabidopsis thaliana (At)SOD1 ~ 5 (a, c) and Cucurbita moschata (Cm)SOD1 ~ 10 (b, d) transgenic lines were compared to NT plants after exposure to a chilling condition for 0 (control) and 192 h. Values are the means of three replicates with the corresponding standard deviation. The gene expression is compaired to NT plants and an asterisk indicates a significance level of p ≤ 0.05.
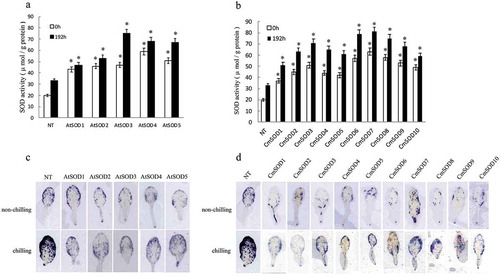
Expressions of the AtCBF2, AtRD29A, and AtRD29B genes in transgenic lines with chilling and ABA treatments
Since AtSOD3 ~ 5 (, panel A) and CmSOD6 ~ 8 (, panel B) showed relatively higher SOD activities than the other transgenic lines at 23 (control) or 4°C (chilling treatment) for 192 h, they were selected to analyze the possibility that ABA be involved in signaling pathways of the AtCBF2, AtRD29A, and AtRD29B genes. shows that RNA levels of AtRD29A (6.10 ~ 14.39, panel B) in the AtSOD3 ~ 5 lines were significantly higher than those of NT plants (0.39 ~ 0.64) after 1 μM ABA treatment and chilling exposure at 6 ~ 192 h. Nevertheless, RNA levels of AtCBF2 (0.42 ~ 0.62, panel A) and AtRD29B (0.38 ~ 0.52, panel C) in the AtSOD3 ~ 5 lines were similar to those of NT plants (0.39 ~ 0.55 in panel A and 0.38 ~ 0.54 in panel C) after 1 μM ABA treatment and chilling exposure for 6 ~ 192 h. The same pattern was also shown in the CmSOD6 ~ 8 lines, and expressions of AtCBF2 (0.42 ~ 0.63, panel A), AtRD29A (0.50 ~ 0.68, panel B), and AtRD29B (0.48 ~ 0.57, panel C) in those lines were close to levels of NT plants (0.38 ~ 0.64) after 1 μM ABA treatment and then exposure to 6 ~ 192 h of chilling.
Figure 5. Relative RNA expressions of the Arabidopsis thaliana (At)CBF2, AtRD29A, and AtRD29B genes in non-transgenic (NT) Arabidopsis plants and transgenic Arabidopsis lines after 1 μM abscisic acid (ABA) treatment followed by 0 to 192 h of chilling stress.
A. The AtCBF2 gene expression in NT plants and T3 homozygote AtSOD3 ~ 5 and Cucurbita moschata (Cm)SOD6 ~ 8 lines.
B. The AtRD29A gene expression in NT plants and T3 homozygote AtSOD3 ~ 5 and CmSOD6 ~ 8 lines.
C. The AtRD29B gene expression in NT plants and T3 homozygote AtSOD3 ~ 5 and CmSOD6 ~ 8 lines.
Total RNA in all tested plants was extracted from 2-week-old plants after 1 μm ABA treatment followed by 4°C for 0 (control), 6, 72, and 192 h of chilling treatment. Relative amounts were calculated and normalized with respect to AtActin-8 RNA. Values are the means of three replicates with the corresponding standard deviation. The gene expression is compaired to NT plants and an asterisk indicates a significance level of p ≤ 0.05.
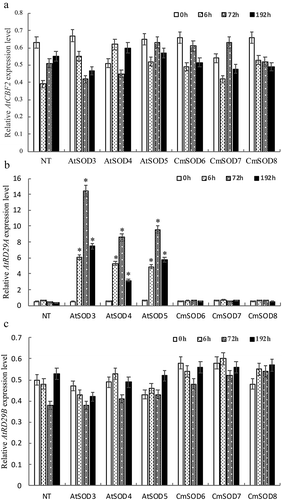
Germination rates, chilling tolerance test, and Chl contents in the transgenic lines
To determine whether T3 transgenic seeds showed tolerance to chilling stress, germination tests were conducted at 23 (non-chilling treatment) and 4°C (chilling treatment). When both transgenic and NT seeds were treated under a non-chilled or chilled condition for 192 h, no significant differences in germination rates were observed between transgenic and NT seeds or among transgenic seeds (data not shown). The effects of chilling on the phenotypes of those 4-week-old NT and transgenic plants are depicted in . Compared to NT plants, the AtSOD () and CmSOD () transgenic lines exhibited chilling tolerance. Leaves of chilled NT plants were epinastic and stunted after 192 h of chilling; however, most leaves of all of the chilled transgenic plants appeared large, green, and healthy.
Figure 6. Chlorophyll contents and seedling growth of non-transgenic (NT) and transgenic Arabidopsis thaliana superoxide dismutase (AtSOD)1 ~ 5 (a) and Cucurbita moschata (Cm)SOD1 ~ 10 (b) lines after exposure to chilling for 0 (control) or 192 h. Values are the means of three replicates with the corresponding standard deviation. An asterisk indicates a significance level of p ≤ 0.05.
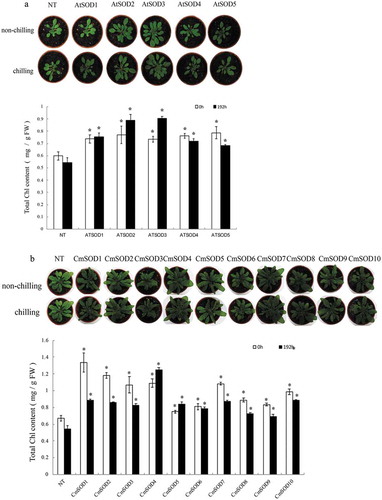
Chilling stress had a harmful effect on leaves of NT plants, and the total Chl content responded differently to chilling stress (, lower panels). Compared to NT plants at 23°C (0.60 mg/g FW) or exposed to chilling (0.55 mg/g FW) for 192 h, Chl contents were significantly higher in all transgenic lines at 23°C (0.73 ~ 1.33 mg/g FW) or when exposed to chilling (0.68 ~ 1.24 mg/g FW) for 192 h, indicating that overexpression of the AtSOD and CmSOD genes reduced the extent of Chl degradation in all of the transgenic lines.
Discussion
Monitoring expressions of plant genes at the transcriptional level is an essential step in their functional analysis. Expression patterns of CmSOD in response to chilling stress provide a molecular basis for improving chilling tolerance in winter squash.Citation2,Citation27 Thus, the increased AtSOD and CmSOD RNA levels were sustained for up to 192 h after 4°C treatment in AtSOD and CmSOD transgenic plants compared to NT plants, indicating that AtSOD and CmSOD gene products were involved in coping with low-temperature stress. The expression profile of SOD genes () was correlated with accumulation of the SOD protein (). SOD activities of all transgenic lines were significantly higher than those of NT plants under control or chilling treatment for 192 h, but various lines acted differently under control or chilling (). Observed variations in relative RNA and protein abundances may have resulted from changes in random T-DNA tagging by the Agrobacterium-mediated transformation,Citation28 and led to each line not necessarily being equally significant in protecting plants against chilling stress. Thus, chilling-induced transcriptional activation of the SOD gene corresponds to an increase in SOD activity for protecting cellular components against the effects of ROS accumulation as a consequence of chilling stress. The presence of SOD, AtCBF2, and AtRD29A/B transcripts in the AtSOD and CmSOD transgenic plants was examined, and its rapid response to chilling stress indicates the importance of this gene in the ROS defense system in plant cells. The capacity of a plant to upregulate the expression and synthesis of SOD defines that plant’s adaptation to chilling stress, with enhanced transcription of SOD and subsequently increased SOD activity leading to enhance chilling tolerance in plants.
The in situ ROS staining method is widely used to identify cellular superoxide (•O2−) accumulation. A high level of •O2− accumulation was observed as a fuscous precipitant (blue color) in NT plants under a chilled condition for 192 h (). All transgenic lines exhibited stronger resistance to chilling tolerance due to less •O2− accumulation. A high level of SOD activity resulted from overexpression of the AtSOD and CmSOD genes, which could eliminate chilling-induced •O2−. The AtSOD and CmSOD genes of transgenic plants are involved in •O2− detoxification and thus helped overcome chilling-induced stress. Presumably, ROS formation and SOD accumulation are favored in chilling tolerance, and the transgenic lines with higher levels of SOD resulted in an increased capacity of the ROS-scavenging system. The chilling-induced transcriptional activation of the AtSOD, CmSOD, AtCBF2, AtRD29A, and AtRD29B genes corresponded to an increase in SOD activity, which protected cellular components against the effects the ROS produced.
Effects of chilling on the germination rate and phenotypes of transgenic plants were also observed in the study. No significant difference in germination rates was detected in all transgenic lines compared to NT plants exposed to chilling stress. In addition, most leaves of NT plants visually exhibited impairment and a smaller size relative to all of the transgenic plants after 192 h of cold treatment. These results are the same as previous results which indicated that cold tolerance during seed germination.Citation29 The Chl content is susceptible to low temperatures and is usually reduced with chilling stress.Citation30 In this study, Chl contents in all NT plants had decreased after 192 h of chilling stress at 4°C, whereas all transgenic plants had higher chlorophyll contents than those of NT plants under both the control and chilled conditions (). Cold stress significantly reduced the measured photosynthetic traits, such as Chl a and b.Citation31 Thus, overexpression of the AtSOD or CmSOD genes in transgenic plants increased Chl accumulation for strengthening chilling tolerance of transgenic plants.
Chilling stress tolerance may have resulted from gene activation and/or upregulation of stress-induced genes. Chilling stress-responsive genes AtCBF2, AtRD22, AtDREB1A, AtRD29A, and AtRD29BCitation8,Citation9 were analyzed using an RT-qPCR under ABA and chilling stress. Compared to NT plants, there were no significant differences between the expressions of AtRD22 and AtDREB1A (data not show). Nevertheless, overexpression of the AtSOD and CmSOD genes activated AtCBF2, AtRD29A, and AtRD29B gene expressions in all transgenic Arabidopsis lines compared to NT plants under chilling stress treatments for 6 ~ 192 h (no ABA pretreatment, ). However, after ABA application and chilling treatments of those transgenic plants, only AtRD29A gene expression in the AtSOD3 ~ 5 lines had significantly strongly increased compared to NT plants (). Yet, expression of AtCBF2 and AtRD29B repressed in all transgenic plants showed no significant differences between NT plants and transgenic lines with ABA and chilling treatments. Overexpression of AtSOD and CmSOD activated AtCBF2, AtRD29A, and AtRD29B gene expressions and obviously enhanced SOD activity to increase chilling tolerance during plant development compared to NT plants under chilling stress. Both the AtSOD and CmSOD genes play important roles in chilling tolerance with different signaling pathways in these transgenic Arabidopsis plants. These findings are important for farming in low-temperature areas or other areas which encounter short and intense cold front events.
The plant hormone, ABA, was shown to increase in plants during cold acclimation.Citation32 Wang et alCitation33 reported that ABA, as an important signal, can mediate abiotic stress (drought, salt, and cold stresses) signal transduction and tolerance. ABA and abiotic stresses can induce both transcriptional and post-translational regulation of genes.Citation11 The AtCBF2 gene is a cold-induced gene and can be rapidly induced by nonfreezing cold stress such as 4 °C; its protein products activate downstream genes known as CBF regulons, leading to protection of plant cells from low-temperature injury.Citation34 Both the AtRD29A and AtRD29B genes are desiccation-responsive transcription factors, and were shown to be differentially responsive to cold, drought, and salt stresses.Citation35 Many systemic-responsive genes involved in hormone responses and metabolism, systemic carbohydrate-managing programs, and ubiquitin-dependent protein degradation were also affected.Citation36 In our study, shows the ABA-senstive AtRD29A, AtCBF2 and AtRD29B transcriptional regulation signaling pathways in the transgenic CmSOD and AtSOD Arabidopsis lines. In these pathways, the three chilling stress-responsive genes, AtCBF2, AtRD29A, and AtRD29B, were highly expressed in all AtSOD and CmSOD transgenic plants compared to NT plants under 4 °C chilling treatment. However, after application of ABA to AtSOD transgenic plants, AtRD29A expression was strongly upregulated, but those of AtCBF2 and AtRD29B were repressed in all AtSOD transgenic plants. While in CmSOD transgenic plants treated with ABA, AtCBF2, AtRD29A, and AtRD29B were all repressed. These results suggest that overexpression of AtSOD activate expressions of downstream target genes by ABA-enhanced AtRD29A or ABA-repressed AtCBF2 and AtRD29B transcriptional regulation signaling pathways in transgenic Arabidopsis under chilling stress. ABA acted as a signal to AtRD29A gene and activated gene products involved in cold acclimation and tolerance of ABA-upregulated pathway in AtSOD3 ~ 5 lines under chilling stress for 6 ~ 192 h. Nevertheless, both AtCBF2 and AtRD29B genes activated gene products involved in chilling response and tolerance of ABA-repressed pathway in those transgenic lines under chilling stress.
Figure 7. Abscisic acid (ABA)-sensitive AtRD29A, AtCBF2 and AtRD29B transcriptional regulation signaling pathways in transgenic Arabidopsis thaliana superoxide dismutase (AtSOD) and Cucurbita moschata (Cm)SOD Arabidopsis lines under chilling (4°C) treatment. The AtCBF2, AtRD29A, and AtRD29B genes were highly expressed in all transgenic lines compared to non-transgenic (NT) plants under chilling treatment. Overexpression of AtSOD activated expressions of downstream target genes by ABA-enhanced AtRD29A or ABA-repressed AtCBF2 and AtRD29B transcriptional regulation signaling pathways in transgenic Arabidopsis under chilling stress.
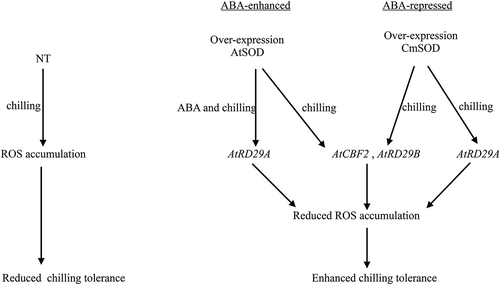
SOD has multiple isoforms in different plant species; these isoforms are located in separate subcellular compartments, often exhibit different properties, and respond in various ways to metabolic and environmental signals.Citation37 All members of the SOD gene family, irrespective of the isoforms they encode, are ultimately associated with general cellular metabolism, stress responses, signaling processes, or the development of chloroplasts.Citation38 For instance, three SOD isoforms, Fe SOD, Mn SOD, and Cu/Zn SOD, exhibit differential expressions (up- or down- regulation) in different subcellular locations in the sunflower seedling cotyledons in response to salt stress.Citation39 In our study, chilling treatments of test plants affecting SOD indicates a correlation with a cellular physiological phenomenon. Further characterization of SOD isoforms will be helpful in elucidating whether or not there are distinct effects among isoforms under chilling stress. A confirmation of the difference in specific SOD isoform expression is worth to be done by undertaking zymography in presence of inhibitors specific for various SOD isoforms.Citation39
In conclusion, the increased tolerance to chilling by Arabidopsis plants overexpressing the AtSOD, CmSOD, AtCBF2, AtRD29A, and AtRD29B genes suggests that these genes may be helpful in enhancing chilling tolerance of different kinds of transgenic plants. The characterization and functional analysis of SOD genes should facilitate our understanding of cold-response mechanisms in plants.
Materials and methods
Plant materials and chilling treatments
Seeds of Arabidopsis thaliana (At) L. ecotype Columbia and winter squash (Cucurbita moschata (Cm) cv. Gon-Zon, from KNOWN-YOU Seed Co., Kaohsiung, Taiwan) were sterilized with 1.5% sodium hypochlorite and rinsed with distilled-deionized (dd)H2O. They were then sown in a commercial potting soil mixture and grown in a growth chamber under 200 μmol m−2 s−1 light with a 16-h photoperiod (16 h of light/8 h of dark), at a temperature of 23°C and a relative humidity of 80% for 2 weeks. Plants were watered every other day, and an optimal amount of compound fertilizer solution (N-P2O5-K2O, 20-20-20) was applied once a week. The seedlings of At and Cm plants were then transferred to 3-inch (7.6-cm) plastic pots randomly placed in the above-mentioned growth chamber with the same growing condition for 2 weeks. Two weeks later, pots were transferred to a cold room for 6 ~ 192 h of acclimation under a 16-h photoperiod with an irradiance of 200 μmol m−2 s−1 at 23°C, followed by exposure to 23°C (control) or 4°C (chilling) for 6 ~ 192 h. Three replicates of each treatment were randomly placed in the growth chamber. Following each treatment, young, fully expanded leaves from each plant were clipped, frozen in liquid nitrogen, and stored at -80°C in an ultra-freezer until used.
Expression analysis of CmSOD and AtSOD genes and amplification of cDNA
Four-week-old plant leaves (0.1 g) with or without chilling treatment for 6 ~ 192 h were used for total RNA isolation using an Ambion Kit (San Francisco, CA, USA). First-strand cDNA synthesis was carried out according to Chiang et al.Citation40 Paired specific primers (CmSOD-F/CmSOD-R and AtSOD-F/AtSOD-R, ) were used for amplification. SOD gene amplification was described in our previous study.Citation40 Briefly, a polymerase chain reaction (PCR) was carried out in an Eppendorf Mastercycler Gradient Thermal Cycler (Hamburg, Germany) with the following thermal program: initial denaturation at 94°C for 2 min, followed by 35 cycles of 94°C for 15 s, 55°C for 30 s, and 72°C for 30 s, with a final extension at 72°C for 10 min. The products were electrophoretically separated on 1.5% agarose gels, and predicted sizes of 600 bp of the CmSOD gene (accession no. AB793712.1) and 696 bp of the AtSOD gene (accession no. NM_111929.3) were verified with a 100-bp DNA ladder marker.
Table 1. Paired primers used in the study.
Plasmid construction
Escherichia coli strain DH5α (Yi-Shan Biotech, Taipei, Taiwan), TOP 10 (Invitrogen, Carlsbad, CA, USA), and the pPZP200/Ubi-HA-ccdB-Nos-35S-hpt-tml destination vectorCitation40 were respectively used for gene construction. The TOPO® Gateway system (Invitrogen, Carlsbad, CA, USA) was used for CmSOD and AtSOD construction. This system was separated into two parts for pENTR® TOPO cloning and a Gateway LR Clonase enzymes mixes (LR) exchange reaction to the destination vector. The CmSOD and AtSOD genes were amplified with paired specific primers from the above-described reverse transcription (RT)-PCR in which ddH2O was used as a negative control. The PCR product was purified using the SNAP Gel Purification Kit (Invitrogen®), ligated to the pENTR TOPO vector, and transformed into TOP10 E. coli-competent cells. After kanamycin screening, plasmid DNA from the transformant E. coli was inserted into pENTR plasmids using paired primers (CmSOD-F/CmSOD-R and AtSOD-F/AtSOD-R, ). The constructed plasmids were named clones pENTR-CmSOD and pENTR-AtSOD. After pENTR cloning, an LR recombination reaction was performed with a Gateway® LR Clonase™ Enzyme Mix Kit (Invitrogen®). The pENTR-CmSOD and pENTR-AtSOD plasmids were exchanged and cloned into the ccdB region of the pPZP200/Ubi-HA-ccdb-Nos-35S-hpt-tml destination vector by LR site-specific recombination. After spectinomycin screening of the colony, plasmid insertion was confirmed by a PCR, and DNA sequences of the pPZP200/Ubi-HA-CmSOD-Nos-35S-hpt-tml (pPZP200-CmSOD) and pPZP200/Ubi-HA-AtSOD-Nos-35S-hpt-tml (pPZP200-AtSOD) clones were confirmed (Supplemental Fig. S1).
Agrobacterium-mediated transformation
A freeze-thaw methodCitation41 was used for plasmid transformation into Agrobacterium tumefaciens strain GV3101. Following plasmid extraction, PCR amplification of the CmSOD (600 bp), AtSOD (696 bp), and hygromycin phosphotransferase (HPT, 987 bp) genes was performed to confirm agrobacterium transformation. Thereafter, a floral dipping methodCitation42 was carried out to deliver the plasmids (pPZP200-CmSOD and pPZP200-AtSOD) into Arabidopsis. Transgenic seedlings were selected on Murashige and Skoog (MS) medium with 50 mg L−1 of hygromycin, and the efficiency of transformation was calculated.
Genomic PCR analysis
Genomic DNA and PCR preparations were carried out essentially as described by Chiang et al.Citation40 The PCR analysis was performed using the specific primers CmSOD5F/CmSOD3R, HA-AtSOD5F/AtSOD3R, and HPT5F/HPT3R () to respectively amplify the CmSOD, AtSOD, and HPT genes. The predicted sizes of 600 (CmSOD), 716 (HA-AtSOD), and 987 bp (HPT) were verified by PCR products separated on a 1.5% agarose gel.
Real-time quantitative (q)PCR and quantification of RNA levels
Relative changes in SOD gene expression with chilling induction were determined by a real-time qPCR. Two-week-old leaves of non-transgenic (NT) Arabidopsis plants and transgenic Arabidopsis lines were used for total RNA extraction. To test whether AtCBF2 (AT4G25470), AtRD22 (AT5G25610), AtDREB1A (AT4G25480), AtRD29A (AT5G52310), and AtRD29B (AT5G52300) gene expressions were induced by ABA and chilling stress, plants were grown on germination medium in agar plates containing 3% sucrose as previously described.Citation43 Two-week-old seedlings of NT plants and transgenic lines were then transferred to the same agar plates described above containing 1 μM of ABA (Sigma A1049, San Jose, CA, USA) and then subjected to chilling for 0 (control), 6, 72, and 192 h. First-strand complementary (c)DNA synthesis was described above, and equal volumes of each first-strand reaction were amplified with CmSOD-118-5F/3R, AtSOD-106-5F/3R, AtCBF2, AtRD29A, and AtRD29 primer pairs (). A real-time qPCR was performed based on our previous study.Citation40 The amplification program consisted of 1 cycle at 94°C for 1 min for pre-incubation, followed by 40 cycles of 94°C for 15 s, 58°C for 30 s, and 72°C for 30 s. Three replicates were performed for each cDNA sample, and template-free and negative controls were both used. To normalize the total amount of cDNA in each reaction, AtActin-8 from ArabidopsisCitation40 was used as an internal control (). Relative amounts of RNA were calculated by the ratio of the abundance of transgenic SOD lines to AtActin-8.Citation44
Western blot analysis
Total soluble proteins were preparedCitation45 from 300 mg of both transgenic and NT plant leaves at 23°C (control) and quantified by the Bradford method (BioRad, Hercules, CA, USA). Forty micrograms of total proteins was electrophoresed on a 5% stacking gel and 10% resolving gel (sodium dodecylsulfate polyacrylamide gel electrophoresis; SDS-PAGE) on Mini PROTEAN III equipment (BioRad). Following electrophoresis, proteins were transferred to an Immobilon-P transfer membrane (Millipore, Burlington, MA, USA). For the Western blot analysis of CmSOD and AtSOD expressions in transgenic Arabidopsis plants, the membrane was incubated at 4°C overnight with a mouse anti-hemoagglutinin (HA) antibody (Sigma, H9658) as described by Chiang et al.Citation27 Bands were detected with an anti-mouse immunoglobulin G (IgG) peroxidase-conjugated secondary antibody for 1 h. The label was detected with an immune-blot assay enhanced chemiluminescence (ECL) Kit (Pharmacia, Stockholm, Sweden) according to the manufacturer’s instructions.
SOD activity assay
Total soluble proteins extracted at 23°C (control) or chilled at 4°C for 192 h were also prepared for SOD (EC 1.15.11) activity analyzes. Enzyme activities were determined using a spectrophotometer. SOD activity was determined using a SOD Assay Kit – WST (Dojindo Molecular Technology, Gaithersburg, MD, USA). The SOD assay kit utilizes the WST working solution of 2-(4-iodophenyl)-3-(4-nitrophenyl)-5-(2,4-disulfophenyl)-tetrazolium, which produces a water-soluble formazan dye upon reduction with superoxide anions (•O2-). The rate of the reduction with O2- is linearly related to the xanthine oxidase activity and is inhibited by SOD. Therefore, 50% of the SOD inhibitory activity can be measured at an absorbance of 450 nm. The specific activity of SOD (inhibition rate) was calculated using the equation described in the protocol of the kit. One unit of enzyme was defined as the amount of enzyme required to decompose 1 μmol of substrate (min−1 g−1 protein).
In situ ROS staining
ROS were stained in situ utilizing the principle of nitroblue tetrazolium (NBT) reduction to blue formazan by •O2-. The intracellular concentration of ROS (•O2-) was directly proportional to the development of the intensity of blue color in the leaves. Briefly, leaves of 2-week old plants from NT and transgenic lines were cut, incubated in 10 mM potassium phosphate buffer (containing 10 mM sodium azide) and vacuum infiltrated for 10 min. The infiltrated leaf tissue was incubated in 0.1% NBT in 10 mM potassium phosphate buffer at pH 7.8 for 30 min. The stained leaf tissue was boiled in an acetic acid: glycerol: ethanol (1:1:3) solution to remove other pigments.Citation16
Seed germination, plant growth, and chlorophyll (Chl) content
Seeds from NT and transgenic lines were placed on 3M filter paper (St. Paul, MN, USA) wetted with ddH2O on a plate, and germinated at 23 and 4°C, respectively, under a 16/8-h light/dark photoperiod for a week, after which the germination rate was calculated.Citation26 Eight-day-old seedlings were then transplanted to 3.6-inch (9.1-cm) plastic pots containing commercial potting soil, and randomly placed in a growth chamber for 35 days under 200 μmol m−2 s−1 light with a 16-h photoperiod provided by fluorescent and incandescent lighting at 23/20°C (day/night). The pots were then subjected to 23°C (control) or a cold room at 4°C (chilling treatment) for 192 h. Chlorophyll was eluted from NT and transgenic leaves in 80% acetone and then centrifuged at 13,000 g for 5 min at 4°C. The supernatants were measured at 663 and 645 nm with a spectrophotometer (U-2000, Hitachi, Tokyo, Japan) according to Arnon.Citation46 The Chl content (mg/g fresh weight (FW)) = (6.1 × OD665 + 20.04 × OD649) × 5 (dilution multiple) ÷ 1000 ÷ sample fresh weight (g).
Statistical analysis
Each NT and transgenic line with three replications was examined to determine RNA levels, SOD activities, in situ ROS staining, germination rates, and chlorophyll contents. A paired t-test was calculated with significance set at p ≤ 0.05.
Highlight
The increased tolerance to chilling by Arabidopsis plants overexpressing the AtSOD and CmSOD genes via ABA-sensitive transcriptional regulation of AtCBF2, AtRD29A, and AtRD29B genes is helpful in enhancing chilling tolerance of different kinds of transgenic plants.
Abbreviations
ABA | = | Abscisic acid |
At | = | Arabidopsis |
CBF | = | C-repeat binding factor |
Chl | = | Chlorophyll |
NT | = | Non-transgenic |
RD | = | Desiccation-responsible |
SOD | = | Superoxide dismutase |
Disclosure of Potential Conflicts of Interest
No potential conflicts of interest were disclosed.
Supplemental Material
Download Zip (12.6 MB)Supplementary material
Supplemental data for this article can be accessed on the publisher’s website.
References
- Salveit ME. Chilling injury is reduced in cucumber and rice seedlings and in tomato pericarp discs by heat-shocks applied after chilling. Posthar. Biol. Tech. 2000;21:1–12. doi:10.1016/S0925-5214(00)00132-0.
- Lin KH, Kuo WS, Chiang CM, Hsiung TC, Chiang MC, Lo HF. Study of sponge gourd ascorbate peroxidase and winter squash superoxide dismutase under respective flooding and chilling stresses. Sci. Hort. 2013;162:333–340. doi:10.1016/j.scienta.2013.08.016.
- Hsu FC, Chou MY, Peng HP, Chou SJ, Shih MC. Insights into hypoxic systemic responses based on analyses of transcriptional regulation in Arabidopsis. PLos ONE. 2011;6:e28888. doi:10.1371/journal.pone.0028888.
- Huang GT, Ma SL, Bai LP, Zhang L, Ma H, Jia P, Liu J, Zhong M, Guo ZF. Signal transduction during cold, salt, and drought stresses in plants. Mol. Biol. Rep. 2012;39(2):969–987. doi:10.1007/s11033-011-0823-1.
- Cheongsamy V, Zhu JK, Sunkar R. Gene regulation during cold stress acclimation in plants. Meth. Mol. Biol. 2010;639:39–55.
- Lee SY, Boon NJ, Webb AA, Tanaka RJ. Synergistic activation of RD29A via integration of salinity stress and abscisic acid in Arabidopsis thaliana. Plant Cell Physi. 2016;57:2147–2160. doi:10.1093/pcp/pcw132.
- Almadanim MC, Alexandre BM, Rosa MTG, Sapeta H, Leitao AE, Ramalho JC, Lam TT, Negrao S, Abreu I, Oliveira MM. Rice calcium-dependent protein kinase OsCPK17 targets plasma membrane intrinsic protein and sucrose-phosphate synthase and is required for a proper cold stress response. Plant Cell Enviro. 2017;40:1197–1213. doi:10.1111/pce.12916.
- Novillo F, Medina J, Rodriguez-Franco M, Neuhaus G, Salinas J. Genetic analysis reveals a complex regulatory network modulating CBF gene expression and Arabidopsis response to abiotic stress. J. Exper. Bot. 2012;63:293–304. doi:10.1093/jxb/err279.
- Shinozaki K, Yamaguchi-Shinozaki K. Gene networks involved in drought stress response and tolerance. J. Exper. Bot. 2007;58:221–227. doi:10.1093/jxb/erl164.
- Nakashima K, Fujita Y, Katsura K, Maruyama K, Narusaka Y, Seki M, Yamaguchi-Shinozaki K. Transcriptional regulation of ABI3-and ABA-responsive genes including RD29B and RD29A in seeds, germinating embryos, and seedlings of Arabidopsis. Plant Mol. Biol. 2006;60(1):51–68. doi:10.1007/s11103-005-2418-5.
- Wang J, Li Q, Mao X, Li A. Wheat transcription factor TaAREB3 participates in drought and freezing tolerances in Arabidopsis. Int. J. Biol Sci. 2016;12:257–269. doi:10.7150/ijbs.13538.
- Nguyen HC, Lin KH, Ho SL, Chiang CM, Yang CM. Enhancing the abiotic stress tolerance of plants: from chemical treatment to biotechnological approaches. Physio. Plant. 2018;164:452–466. doi:10.1111/ppl.12812.
- Xu S, Li J, Zhang X, Cui L. Effects of heat acclimation pretreatment on changes of membrane lipid peroxidation, antioxidant metabolites, and ultrastructure of chloroplasts in two cool-season trufgrass species under heat stress. Enviro. Exper. Bot. 2006;56:274–285. doi:10.1016/j.envexpbot.2005.03.002.
- Kreslavski VD, Lyubimov VY, Balakhnina TI, Kosobryukhov AA. Heat-induced impairments and recovery of photosynthetic machinery in wheat seedlings. Role of light and prooxidant antioxidant balance. Physio.Mol. Biol. Plants. 2009;15:115–122. doi:10.1007/s12298-009-0013-y.
- Passaia G, Spagnolo FL, Caverzan A, Jardim-Messeder D, Christoff AP, Gaeta ML, de Araujo Mariath JE, Margis R, Margis-Pinheiro M. The mitochondrial glutathione peroxidase GPX3 is essential for H2O2 homeostasis and root and shoot development in rice. Plant Sci. 2013;208:93–101. doi:10.1016/j.plantsci.2013.03.017.
- Shafi A, Dogra V, Gill T, Ahuja PS, Sreenivasulu Y. Simultaneous over-expression of PaSOD and RaAPX in transgenic arabidopsis thaliana confers cold stress tolerance through increase in vascular lignifications. PLos ONE. 2014;9(10):e110302. doi:10.1371/journal.pone.0110302.
- Kim MD, Kim YH, Kwon SY, Yun DJ, Kwak SS, Lee HS. Enhanced tolerance to methyl viologen-induced oxidative stress and high temperature in transgenic potato plants overexpressing the CuZnSOD, APX and NDPK2 genes. Physio. Plant. 2010;140:153–162. doi:10.1111/j.1399-3054.2010.01392.x.
- Xi DM, Liu WS, Yang GD, Wu CA, Zheng CC. Seed-specific overexpression of antioxidant genes in Arabidopsis enhances oxidative stress tolerance during germination and early seedling growth. Plant Biotech. J. 2010;8:796. doi:10.1111/j.1467-7652.2010.00509.x.
- Gill T, Dogra V, Kumar S, Ahuja PS, Sreenivasulu Y. Protein dynamics during seed germination under copper stress in arabidopsis over-expressing potentilla superoxide dismutase. J. Plant Res. 2012;125:165–172. doi:10.1007/s10265-011-0421-2.
- Shafi A, Gill T, Zahoor I, Singh AP, Sreenivasulu Y, Komar S, Kumar SA. Ectopic expression of SOD and APX genes in Arabidopsis alters metabolic pools and genes related to secondary cell wall cellulose biosynthesis and improve salt tolerance. Mol. Biol. Rep. 2019;46:1985–2002. doi:10.1007/s11033-019-04648-3.
- Zhao Y, Liu M, He L, Li X, Wang F, Yan B, Wei J, Zhao C, Li Z, Xu J. A cytosolic NAD+-dependent GPDH from maize (ZmGPDH1) is involved in conferring salt and osmotic stress tolerance. BMC Plant Biol. 2019;19:16. doi:10.1186/s12870-018-1597-6.
- Mishra A, Tanna B. Halophytes: potential resources for salt stress tolerance genes and promoters. Fronti. Plant Sci. 2017;8:829. doi:10.3389/fpls.2017.00829.
- Ijaz R, Ejaz J, Gao S, Liu T, Imtiaz M, Ye Z, Wang T. Overexpression of annexin gene AnnSp2, enhances drought and salt tolerance through modulation of ABA synthesis and scavenging ROS in tomato. Sci. Rep. 2017;7(1):12087. doi:10.1038/s41598-017-11168-2.
- Wang N, Zhang W, Qin M, Li S, Qiao M, Liu Z, Xiang F. Drought tolerance conferred in soybean (Glycine max. L) by GmMYB84, a novel R2R3-MYB transcription factor. Plant Cell Physiol. 2017;58:1764–1776. doi:10.1093/pcp/pcx111.
- Wang X, Chen J, Liu C, Luo J, Yan X, Ai A, Cai Y, Xie H, Ding X, Peng X. Over-expression of a protein disulfide isomerase gene from Methanothermobacter thermautotrophicus, enhances heat stress tolerance in rice. Gene. 2019;684:124–130. doi:10.1016/j.gene.2018.10.064.
- Chiang CM, Kuo WS, Lin KH. Cloning and gene expression analysis of sponge gourd ascorbate peroxidase gene and winter squash superoxide dismutase gene under respective flooding and chilling stresses. Hort. Enviro. Biotech. 2014;55:129–137. doi:10.1007/s13580-014-0116-4.
- Chiang CM, Chien HL, Chen LFO, Hsiung TC, Chiang MC, Chen SP, Lin KH. Overexpression of the genes coding ascorbate peroxidase from Brassica campestris enhances heat tolerance in transgenic Arabidopsis thaliana. Biologia Plant. 2015;59(2):305–315. doi:10.1007/s10535-015-0489-y.
- Kim SI, Veena GSB. Genome‐wide analysis of Agrobacterium T‐DNA integration sites in the Arabidopsis genome generated under non‐selective conditions. The Plant J. 2007;51:779–791. doi:10.1111/j.1365-313X.2007.03183.x.
- Foolad MR, Lin GY. Relationship between cold tolerance during seed germination and vegetative growth in tomato: analysis of response and correlated response to selection. J.Amer. Soc. Hort. Sci. 2001;126:216–220. doi:10.21273/JASHS.126.2.216.
- Gajewska E, Sklodowska M, Slaba M, Mazu J. Effect of nickel on antioxidant enzyme activities, praline and chlorophyll contents in wheat shoots. Biolo. Plant. 2006;50:653–659. doi:10.1007/s10535-006-0102-5.
- Hajihashemi S, Noedoost F, Geuns JM, Djalovic I, Siddique KH. Effect of cold stress on photosynthetic traits, carbohydrates, morphology, and anatomy in nine cultivars of Stevia rebaudiana. Fronti. Plant Sci. 2018;9:2018.
- Lang V, Mantyla E, Welin B, Sundberg B, Palva ET. Alterations in water status, endogenous abscisic acid content, and expression of rab18 gene during the development of freezing tolerance in Arabidopsis thaliana. Plant Physiol. 1994;104:1341–1349. doi:10.1104/pp.104.4.1341.
- Wang X, Wang L, Wang Y, Liu H, Hu D, Zhang N, Tang S, Cao H, Cao Q, Zhang Z. Arabidopsis PCaP2 plays an important role in chilling tolerance and ABA response by activating CBF-and SnRK2-mediated transcriptional regulatory network. Front. Plant Sci. 2018;9:215. doi:10.3389/fpls.2018.00215.
- Rasmussen S, Barah P, Suarez-Rodriguez MC, Bressendorff S, Friis P, Costantino P, Bones AM, Nielsen HB, Mundy J. Transcriptome responses to combinations of stresses in Arabidopsis. Plant Physiol. 2013;161:1783–1794. doi:10.1104/pp.112.210773.
- Yamaguchi-Shinozaki K, Shinozaki K. A novel cis-acting element in an Arabidopsis gene is involved in responsiveness to drought, low-temperature, or high-salt stress. The Plant Cell. 1994;6:251–264. doi:10.1105/tpc.6.2.251.
- Gibbs DJ, Lee SC, Isa NM, Gramuglia S, Fukao T, Bassel GW, Correia CS, Corbineau F, Theodoulou FL, Bailey-Serres J, et al. Homeostatic response to hypoxia is regulated by the N-end rule pathway in plants. Nature. 2011;479:415–418. doi:10.1038/nature10534.
- Maecka A, Jarmuszkiewicz W, Tomazewska B. Antioxidative defense to lead stress in subcellular compartments of pea root cells. Acta Bioquimica Polonica. 2001;48:687–698.
- Kavitha K, Venkataraman G, Parida A. An oxidative and salinity stress induced peroxisomal ascorbate peroxidase from Avicennia marina: molecular and functional characterization. Plant Physiol. Biochem. 2008;46:794–804. doi:10.1016/j.plaphy.2008.05.008.
- Arora D, Bhalata SC. Melatonin and nitric oxide regulate sunflower seedling growth under salt stress accompanying differential expression of Cu/Zn SOD and Mn SOD. Free Radical Biol. Med. 2017;106:315–328. doi:10.1016/j.freeradbiomed.2017.02.042.
- Chiang CM, Chen CC, Chen SP, Lin KH, Chen LR, Su YH, Yen HC. Overexpression of the ascorbate peroxidase gene from eggplant and sponge gourd enhances flood tolerance in transgenic Arabidopsis. J. Plant Res. 2017;130:373–386. doi:10.1007/s10265-016-0902-4.
- Wise AA, Liu Z, Binns AN. Three methods for the introduction of foreign DNA into Agrobacterium. Meth. Mol. Biol. 2006;343:43–53.
- Clough SJ, Bent AF. Floral dip: a simplified method for Agrobacterium- mediated transformation of Arabidopsis thaliana. The Plant J. 1998;16:735–743. doi:10.1046/j.1365-313x.1998.00343.x.
- Hu Y, Yu D. Brassinosteroid insensitive2 interacts with abscisic acid insensitive to mediate the antagonism of brassinosteroids to abscisic acid during seed germination in Arabidopsis. The Plant Cell. 2014;26:4394–4408. doi:10.1105/tpc.114.130849.
- Livak KJ, Schmittgen TD. Analysis of relative gene expression data using real-time quantitative PCR and the 2(-Delta DeltaC(T)) method. Methods. 2001;25:402–408. doi:10.1006/meth.2001.1262.
- Wang W, Vignani R, Scali M, Cresti M. An universal and rapid protocol for protein extraction from recalcitrant plant tissues for proteomic analysis. Electroph. 2006;27:2782–2786. doi:10.1002/elps.200500722.
- Arnon DI. Copper enzymes in isolated chloroplasts, polyphenoxidase in Beta vulgaris. Plant Physio. 1949;24:1–15. doi:10.1104/pp.24.1.1.