ABSTRACT
Salt stress, causing serious loss on crop productions, is one of the most important environmental stresses throughout the world. The aim of this study is to select salt-tolerant traditional rice resources collected from Lei-Qiong area of South China and investigate their physiological performances and biochemical regulations during salt stress response, together with two well-known international varieties, Nona Bokra (salt-tolerant sample) and IR29 (salt-sensitive sample). After comprehensive analyses, we discovered that two Lei-Qiong traditional salt-tolerant rice samples showed less growth inhibition by salt stress during both germination and seedling stage, in comparison with other rice samples. Moreover, there were less chlorosis symptoms in these two kinds of salt tolerant rice under salt stress, corresponding to their better water-holding capacity. We measured malondialdehyde and proline contents, and activities of CAT and POD of seedlings treated with 100 mM NaCl for 5 dand 10 d, respectively. Interestingly, less cellular membrane damage and stronger antioxidant enzyme system were found in the two Lei-Qiong rice samples. Our study suggests that traditional rice landrace growing onshore of Lei-Qiong area in China possesses good salt-tolerant capacity, which could be attributed to their efficient antioxidant enzyme system.
Introduction
Rice (Oryza sativa) is one of the most important crops on the Earth, as it feeds more than 50% of population. Previous reports indicated that the increasing human population is expected to reach nine billion in 2050. In this case, we need at least 70% increase in agricultural crop productivity to meet the demand of the population in the mid of twenty-first century.Citation1,Citation2 It has been widely accepted that salinity stress, limiting crop production of over 800 million hectares globally, is one of the major abiotic stresses to rice production.Citation3,Citation4 To reduce the loss in rice production due to salinity stress, different agricultural practices have been used. One of the strategies is that using better irrigation management to improve soil quality for enhancing plant production. However, this practice has been demonstrated to be mostly unfeasible for its high cost and difficulty to handle on a larger scale.Citation5 Therefore, generation of rice varieties with improved salt tolerance through transgenic technology or conventional breeding is essential for combating salt stress.Citation6,Citation7
Extensive salt in soils usually produces two kinds of stresses to plants. Firstly, osmotic stress due to excess solutes reducing water potential and consequently decreasing water uptake by the root system. Secondly, the ionic toxicity results from excessive influx of Na+ ions into the plant.Citation8,Citation9 Taken together, these stresses comprise the main reasons for metabolic chaos, growth inhibition, and even death of the plant under salinity environments.Citation10 It has been widely accepted that the early seedling stage is one of the most sensitive stages to salinity stress in rice.Citation5,Citation11 One effect induced by high salt accumulation in seedlings is chlorosis symptom, resulting from thylakoid damage in chloroplasts,Citation12,Citation13 followed by a gradual decrease in the activity of photosystems and growth inhibition.Citation11,Citation14 Reactive oxygen species (ROS) such as H2O2 and O2− is a product of the incomplete reduction of molecular oxygen, low content of which functions as signaling molecules controlling the stomatal aperture. However, because of their high chemical reactivity due to the presence of uncoupled electron within their molecules, extensive content of ROS under stresses such as salt stress and drought, results in damage to the large molecules and cell membrane in plants, which is considered a symptom of cellular dysfunction.Citation15,Citation16 High ROS accumulation would cause enhanced lipid peroxidation. And, the resulting lipid peroxides are subsequently metabolized into malondialdehyde (MDA) and 4-hydroxynonenal (4-HNE). Therefore, MDA concentration can be used as an indicator for the ROS-dependent cell damage.Citation17,Citation18
Salt tolerance of a plant is mainly dependent on its capability to control the amount of sodium reaching its sensitive cells in leaf and/or to reprogram metabolism for stress resistance when roots are subjected to high salt content.Citation19 When sensing salt stress, plants could induce a gene network regulation as a resistant response, followed by various biochemical and physiological reactions.Citation20,Citation21 Concentrations of protein and numerous metabolites, including proline and soluble sugars, functioning as compatible solutes, are usually increased when the plant cell sense salt stress.Citation21,Citation22 It is to be noted that the accumulation of proline is not always positively correlated to the salt-tolerant capacity of rice.Citation23 Increases in levels of antioxidants (e.g., ascorbate, glutathione, and carotenoids) and activities of detoxifying enzymes, such as POD, CAT, and enzymes in the ascorbate-glutathione cycle usually confer to plant tolerance to salinity, by avoiding excessive accumulation of ROS under salt stress.Citation24,Citation25 After all, variations in the intensity of metabolic reprogram may confer complicated and variable salt-tolerant capacities in different rice genotypes.
Plants in coastal areas are exposed to saline soils and saline water more frequently than those in inland. So coastal area could be home to many salt-tolerant plants. There is about 1 million ha of coastal soil suffering from salinity in Bangladesh, mostly due to sea-water intrusion.Citation26 Leizhou Peninsula – Hainan Island in South China, called as Lei-Qiong for short, possesses a long coastline. This area has a long history of rice cultivation.Citation27,Citation28 We predicted that traditional rice landraces growing in this area may possess genetic resource conferring themselves with good salt-tolerant capacity.
In this project, we collected rice seed stocks from Lei-Qiong area, followed by propagation and screen work. Two of the most tolerant traditional rice landraces and one of the less tolerant landraces were used for further inspection. By comparison, we found that the two salt-tolerant rice landraces collected from Lei-Qiong area are, to a certain extent, more resistant to salt stress when compared with Nona Bokra, a widely used salt-tolerant rice landrace globally,Citation29 as the two salt-tolerant rice landraces collected from Lei-Qiong area showed less growth inhibition and better water-holding capacity under salt stress, which could be attributed to less ROS-dependent cell membrane damage. Our results suggested that these traditional rice landraces in Lei-Qiong area could possess a more efficient antioxidant system, and would be good sources for salt-tolerant rice breeding.
Results
Rice seed collection and screen
With the prediction that the rice landrace around Lei-Qiong area may possess a good salt tolerance trait. We collected more than 30 rice samples from this area, and propagated them on our experimental fields. As it is well known that the First Green Revolution in rice happened around the 1960s which produced semi-dwarf rice varieties to get increased yields,Citation30,Citation31 we removed five rice samples from further observation as they are dwarf and looks like commercial varieties (data not shown), and were supposed to be the mixed seeds of non-traditional rice varieties.
The sampling points of rice landraces are distributed widely and at different heights, indicating the probability of seawater immersion is different around these points. In this case, the plants grew, generation after generation, on these places may possess variable salt tolerance and genotypes through adaptation and evolution. To select the most salt-tolerant samples for further tests, we preliminary compared salt tolerance between this subset of traditional rice samples. As shown in , the salt tolerance capacity between different rice samples varied. In detail, B11, C22, and C32 performed worst, not a single seedling from them survived in the test. A subgroup of traditional rice samples had some seedlings survived at end of the test, including C7, C16, HG1, B36, and so on. To our notice, C34, C24, and C6 performed best with the highest survival rates. This result indicated that there could be variations on the molecular level affecting salt tolerance between this subset of rice samples.
Figure 1. Comparison of salt tolerance between different Lei-Qiong traditional rice samples. Twenty-eight kinds of Lei-Qiong rice samples were grown on Hoagland solution for about 3 weeks, and then fresh Hoagland solution with 0.5% NaCl was added for three times in every second day (the old solution is running out at this time point). After that, solution without NaCl was added again when the solution running out again. Salt tolerance capacity was roughly estimated by the survival rate in 10 d after salt treatment.
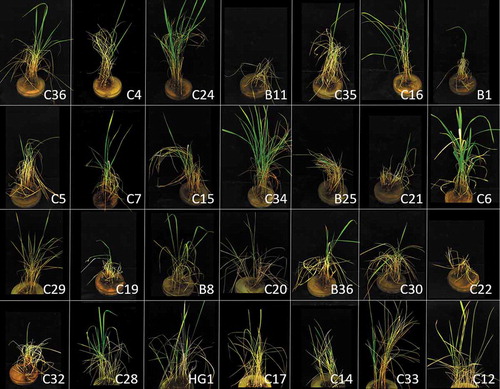
Lei-Qiong rice landrace showed salt tolerance during germination stage
To further study the salt tolerance of Lei-Qiong rice landraces, we used two of the salt-tolerant samples, C24 and C34, together with one less tolerant sample, HG1, from our Lei-Qiong rice landrace pool, and compared with the popular Indian salt-tolerant rice landrace Nona Bokra, and salt-sensitive rice species, IR29.Citation32 In our seed germination test, more than 98% of seeds from all samples germinated in control condition. Germination was affected little under 50 mM NaCl treatment. When the NaCl concentration increased to 100 mM, about 90% of seeds from all samples still germinated. However, the germination rate showed significant differences between different rice samples under higher salt concentrations. When the NaCl concentration reached 150 mM, seeds of Nona Bokra and IR29 showed about 60% of germination rates, whereas HG1 kept as high as 86% of germination rate, and C34 and C24 show 100% germination rate under the same salt stress. Under 200 mM NaCl treatment, the germination rates of Nona Bokra, HG1 and IR29, drop to 30%, 60%, and 14%, respectively. However, only less than 5% of seeds form C24 and C34 did not germinate under this treatment, indicating their strong salt-tolerant capacity during the germination stage. Under 300 mM NaCl treatment, C24 and C34 showed 40% and 54% of germination rate, but no seed from other rice samples used in this experiment showed germination. It is noteworthy that, the elongation rate of radicle and shoot of germinated seeds were inhibited considerably by high NaCl concentration ().
Figure 2. Germination rates of different rice landraces under salt treatment. Germination rate of C34, C24, HG1, Nona Bokra, and IR29 under 0 mM, 50 mM, 100 mM, 150 mM, 200 mM, 250 mM, and 300 mM NaCl treatments. (A) Pictures of seed germination under different conditions after 6 d of incubation. Names of rice samples were shown at left of each line, NaCl concentrations were indicated on top of each row. (B) Germination rates under different conditions. Data were mean of three replicates, no less than 50 seeds were used in each replicate.
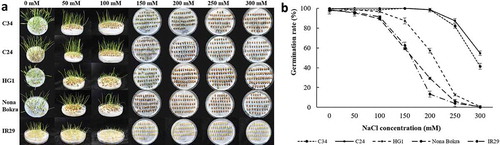
Lei-Qiong rice C24 and C34 seedlings showed less growth inhibition under salt stress
Seedling stage of rice is one of the two stages that most sensitive to salt stress in its whole life.Citation5,Citation33 In previous test, we found that all three Lei-Qiong rice landraces showed stronger salt tolerance than Nona Bokra and IR29 during the seed germination stage. However, it is unclear whether those rice samples perform the same during seedling stage. In our analysis, 4-weeks-old rice seedlings were transferred to new media containing 0 mM, 80 mM, 100 mM, 120 mM, and 150 mM NaCl for 10 d, followed by phenotyping. As shown in , relative growth inhibition was found in all rice samples after NaCl treatment. In detail, IR29 almost stopped growing and totally wilted in aerial part when incubated in nutrient medium containing as high as 100 mM NaCl for 10 d. HG1 seedlings performed better than that of IR29. Aerial part elongation of Nona Bokra was affected significantly by NaCl treatments when compared with that of C24 and C34 seedlings (). In the case of root elongation, again, IR29 performed worst, with nearly 50% of relative root growth inhibition rate under 80 mM NaCl treatment, and more than 90% of relative root growth inhibition rate by 100 mM NaCl treatment. Nona Bokra root was more sensitive to salt than that of HG1 in low concentration NaCl treatment in our experiment, showing more than 80% of relative root growth inhibition rate at 100 mM NaCl treatment. Roots of C34 and C24 seedlings appeared more tolerant to salt stress than that of other rice samples, especially roots of C24, which maintained considerable growth rates even under 150 mM NaCl treatment ().
Figure 3. Performances of different rice landraces under salt treatments. Three-weeks old C34, C24, HG1, Nona Bokra, and IR29 seedlings were treated with 0 mM, 80 mM, 100 mM, 120 mM, and 150 mM NaCl for 10 d. Relative inhibition rate was indicated by relative reduction of plant height or plant root compared to control condition (0 mM NaCl). Each treatment was having 3 replicates, 10 plants were calculated in each replicate. (A) Relative inhibition rates of aerial part. (B) Relative inhibition rates of roots. (C) Water contents of rice seedlings. The harvested materials were dried in an oven for 3 d. (D) Chlorophyll contents measurement. Leaves were harvested and processed as shown in methods. (E) Pictures of seedlings after treatment, NaCl concentrations were indicated on the top of each panel. Data key: Different letters stand for having significant difference (P < 0.05) within the same treatments. Ruler bars (shown in red), 20 cm.
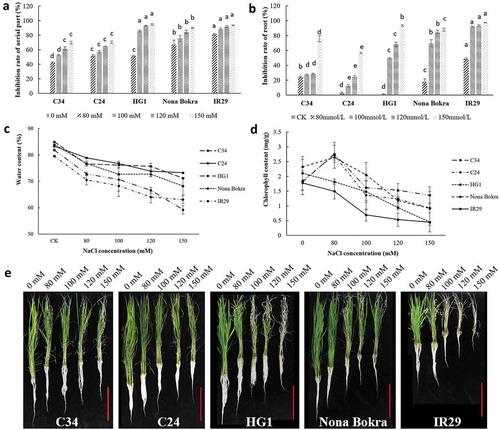
Seedlings with better water-holding capacity possessed stronger chlorosis resistance
Under salt stress, plants face challenges in absorbing water from the media solution as the water potential in the environment is decreased by the high concentration of salt ions.Citation34 We measured water-holding capacity of different rice samples after NaCl treatments. Results showed that salt treatments caused water loss in all rice seedlings in a concentration-depend manner. However, the rates of water loss were different between varieties. Water loss rate was higher in HG1 and IR29 than others under the same NaCl treatment. C24 seedlings to certain extent performed better than C34 and Nona Bokra in holding water under saline condition (). Plants growing in high salty environments may develop chlorosis and consequently, leaf photosynthesis capacity is affected. Therefore, the capacity to keep leaves green to certain extent can represent its salt tolerance. In our experiments, HG1 and IR29 showed decrease of chlorophyll at as high as 80 mM NaCl treatment, whereas C24, C34 and Nona Bokra showed increase in chlorophyll content in 80 mM NaCl treatment and followed by decrease at higher concentration of NaCl. Thus, leaves from these seedlings maintained more chlorophyll than the IR29 and HG1 in medium containing as high as 150 mM NaCl (,e). After all, there is a positive correlation between water-holding capacity and chlorosis resistance under salt stress.
Metabolic process in different rice landraces varied under the same salt treatment
Environmental stress could cause a rebalance of metabolites within plants to guide plants to adapt to the suboptimal conditions.Citation35 As we found different rice varieties performed differently under salt treatments, we would like to know that whether metabolic processes in these rice seedlings were also changed differently under the same salt treatment. MDA content could be used to measure the level of injury of plasma membrane by salt.Citation18 In our experiments, the MDA concentration increased gradually in all rice samples after salt treatment. MDA concentration in IR29 was higher than other rice seedlings in any condition, especially after salt treatment, indicating that IR29 possessed a more fragile cell membrane system with a higher degree of lipid peroxidation. MDA content is HG1 seedlings did not increase significantly when incubated in solution with 100 mM NaCl for 5 d. However, MDA content increased more intensively in this rice landrace when incubated with salt for another 5 d, suggesting that cell membrane of HG1 could be destroyed more obviously by long period of salt stress, when compared with other salt-tolerant rice seedlings. Interestingly, MDA contents in C24 and C34 were lower than that of Nona Bokra after 100 mM NaCl treatment for 10 d, which can be correlated with their performance better than Nona Bokra by maintaining growth under salt stress ().
Figure 4. MDA and proline contents of rice seedlings. Four-weeks old C34, C24, HG1, Nona Bokra, and IR29 seedlings were treated by 100 mM NaCl treatments for 5 d and 10 d, respectively. Seedlings were harvested after treatment, seedlings without NaCl treatment were used as control. (A) Measurement of MDA contents. (B) Measurement of proline contents. Each treatment was having 3 replicates, 12 plants were calculated in each replicate. Data key: Different letters stand for having significant difference (P < .05) within the same treatments. Asterisks (*) indicate significant differences between control and salt-stressed seedlings of the same genotype which were determined by Student’s t-test (*0.01 < P ≤ 0.05, **0.001 < P ≤ 0.01, ***P ≤ 0.001).
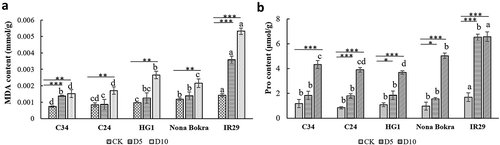
When plant cells suffered from osmotic stress, metabolic processes within these cells may rebalance and produce proteins, proline (Pro), and soluble sugars to keep low water potential of the cells, which may facilitate the cells water absorption.Citation22 However, the extent of accumulation of the metabolite Pro is not always positively correlated to the salt-tolerant capacity of plants.Citation23 In our experiment, Pro contents in all kinds of seedlings were induced by salt treatment, with a positive correlation with the treatment time in general. Pro concentration in Nona Bokra was similar with that of the three Lei-Qiong rice seedlings under control condition and 5 dafter 100 mM NaCl treatment, but increased quickly when growing in NaCl solution for 5 more days. In the case of IR29, Pro production increase quickly when this rice species was transferred to solution with 100 mM NaCl for only 5 d, corresponding with its salt-sensitive phenotype ().
Lei-Qiong rice C24 and C34 possessed stronger antioxidant system
When there is excessive accumulation of ROS under salt stress, detoxifying enzymes, such as POD, CAT, together with other antioxidants are usually employed in plant cells to combat oxidative stress.Citation25 In our experiments, the activity of POD generally increased more or less within 5 d after incubation with 100 mM NaCl and somewhat decreased when the incubation time prolonged. POD Activity in C24 seedlings was highest both before and after NaCl treatment, immediately followed by C34. However, the difference of POD activity between Nona Bokra, HG-1, and IR29 was not obvious before salt treatment and at the two time points we tested, namely, 5 dand 10 d after salt treatment (). The activity of another antioxidant enzyme, CAT, did show a clear decrease in all seedlings after 5 d and 10 dof salt treatment, compared to that of control condition. Again, C24 and C34 seedlings showed more CAT activities than other landraces used (). After all, seedlings of the two Lei-Qiong salt-tolerant rice samples, C24 and C34, had a stronger ability to remove ROS accumulation under salt stress in comparison with other seedlings tested in our experiments. This result correlated positively with their performance in previous phenotyping experiments.
Figure 5. POD and CAT activities of seedlings. Four-weeks old C34, C24, HG1, Nona Bokra, and IR29 seedlings were treated by 100 mM NaCl treatments for 5 d and 10 d, respectively. Seedlings were harvested after treatment, seedlings without NaCl treatment were used as control. (A) POD activity. (B) CAT activity. Each treatment was having 3 replicates, 12 plants were calculated in each replicate. Data key: Different letters stand for having significant difference (P < .05) within the same treatments. Asterisks (*) indicate significant differences between control and salt-stressed seedlings of the same genotype which were determined by Student’s t-test (*0.01 < P ≤ 0.05, **0.001 < P ≤ 0.01, ***P ≤ 0.001).
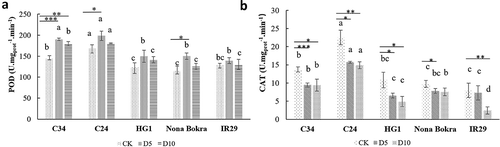
Discussion
Salt stress posing a great risk to crop production and increasing the human population make challenge to the sustainable development of human society. Genetic improvement of salt tolerance capacity of cereal crops like rice (Oryza sativa), maize (Zea mays), wheat (Triticum aestivum), and barley (Hordeum vulgare) is important for maintaining stable global food production. In rice, several salt-tolerant QTLs have been identified from different rice genotypes, such as Pokkali and Nona Bokra.Citation29,Citation36 In the present study, we predicted that traditional rice landraces growing on shore of Lei-Qiong area in South China, possess good salt tolerance, as plants in this environment are very often submerged by seawater when high tide during their long growing history. With the aim to collect traditional genotypes that are adapted to the saline soils around the shore, we firstly removed rice samples that are dwarf, which could be the products of the first green revolution.Citation30,Citation31 We have screened 28 traditional rice samples with different salt tolerance capacities, indicating the genotypes of the rice samples may possess variation on salt tolerance-related gene(s).
To further study their characteristics, two salt-tolerant samples, C34 and C24, together with one less tolerant sample, HG1, from Lei-Qiong area were used as experimental materials. The salt-tolerant rice genotype Nona BokraCitation29 and salt-sensitive genotype, IR29Citation11,Citation32 were also included as control samples in our experiment to evaluate the salt tolerance of Lei-Qiong rice materials. In seed germination experiment, NaCl treatment delayed and reduced the germination in almost all rice samples in a concentration-dependent manner, corroborating the conclusion in earlier reports that besides the toxic effects, high concentration of salt environment reduces the water absorption by germinating seeds during imbibition and therefore represses the germination.Citation37 The salt-sensitive genotype IR29 showed lowest germination rate under salt stress as expected. It is noteworthy that the three Lei-Qiong rice samples appeared to be more resistant to NaCl treatments during germination when compared to the popular salt-tolerant rice landrace, Nona Bokra, in our experiment.
It is well known that the seedling stage of rice is more sensitive to salt stress when compared with its germination stage,Citation5,Citation33 so salt-tolerant capacity during seedling stage would be a more useful trait for agricultural production. Salt stress may suspend plant growth by restraining cell elongation in the shoot and root, which result from ionic toxicity and osmotic stress of sodium ion.Citation3,Citation9 Results of our measurements demonstrated that C24 and C34 showed less growth inhibitions under NaCl treatments. These phenotyping results suggested that a subgroup of traditional rice samples growing in Lei-Qiong area of China could be a good resource for salt-tolerant rice breeding.
Water loss happens frequently when plant suffered from osmotic stress, such as salt stress and drought.Citation23 The results of water content measurement revealed that C34 and C24 possess relatively stronger water-holding capacity than other rice samples under salt stress. Nona Bokra performed better than HG1 and IR29 in holding water under high concentration of NaCl. It has been demonstrated that salt stress-mediated decrease in total chlorophyll concentration may be due to toxic ion accumulation which affects the membrane stability and causes damage.Citation38 Physiological analysis revealed that under 100 mM NaCl treatment, chlorophyll contents in C34, C24, and Nona Bokra were not reduced obviously, while chlorosis occurred considerably in HG1 and the salt-sensitive genotypes IR29. The reduction in chlorophyll content and together with decline in the fresh weights of plant tissues under saline conditions are observed in different crops.Citation10,Citation39 Significant reduction of growth rate in IR29 corresponds with its severe chlorosis symptom by NaCl treatment. In contrast, C24, C34 and Nona Bokra showed less inhibition rate of aerial part together with relatively better ability in protecting chloroplast from damage. Notably, although Nona Bokra showed less water loss and chloroplast damage, it performed worse than HG1, to certain extent, in the case of keeping growth under salt stress. These results indicated that different rice landraces may tolerate salt stress by different strategies; water holding and chloroplast protection may correlate positively with each other, but the growth rate may not have a definite connection with these two kinds of capacities under salt stress.
The cellular membrane is one of the key targets of many abiotic stresses, and it is noteworthy that the protection of membrane damage under salt stress correlated with better salinity tolerance.Citation40 Here, MDA amount increased in all landraces after treated by NaCl. The MDA content in IR29 increased quickly after treatment, which corresponds to its most salt-sensitive phenotype. C34 and C24 were found to restrict the amount of MDA a little more efficiently than Nona Bokra. These results indicated cellular membranes of C34 and C24 seedlings were less damaged, compared to Nona Bokra and the other two less salt-tolerant rice samples under the same salt condition. Our result corresponds with previous studies which demonstrated that the MDA amount increased mostly in salt-sensitive rice cultivars.Citation41
Several reports demonstrated that osmolytes like proline and soluble sugars contribute immensely toward protecting the membrane proteins, and other enzymes apart from its basic role of osmoprotection,Citation9,Citation22 and as a result, proline content was used frequently for indicating stress-tolerant capacity of plants. Interestingly, IR29, the one considered to be the most sensitive rice sample showing the most severe growth inhibition, chlorosis symptom, and obvious water loss when suffering salt stress in our phenotyping study, accumulated the highest levels of proline in its tissues. Whereas C24 and C34, which performed better than others in phenotyping experiments, together with another Lei-Qiong rice sample, HG1, showed relatively low content of proline. Furthermore, the proline content of Nona Bokra increased faster than the three Lei-Qiong landraces after NaCl treat for 10 d. Our results corresponded with the conclusion of Chen et al. That a strong correlation between proline accumulation and salt tolerance is not always obvious, and instead, proline accumulation could be a symptom of injury rather than an indicator of stress tolerance in rice.Citation42 After all, our results and some others suggest that the nature of proline accumulation under stress is still debated, Yang et al. also found that the transgenic rice with stronger salt tolerance did not produce more proline under certain conditions when compared with its wild type plant.Citation43 Maybe other factors such as the stress intensity, stress duration, genotype, and so on, need to be counted for achieving more information in detail when interpreting the relation between proline content and salt stress.
ROS as byproducts of photosynthesis, respiration, photorespiration have to be maintained in an optimal level to protect plants from oxidative stress and to function as signaling molecules mediating many physiological processes in plants.Citation44 The critical balance between production and scavenging of ROS gets disturbed by abiotic stresses like drought and salinity and this leads to a drastic increase in intracellular ROS levels which can cause significant damage to cellular structures. ROS scavenging mechanisms including enzymatic and non-enzymatic (antioxidants) are often activated in plants.Citation25 Recent studies reported that an increase in activity of antioxidative enzymes enhanced salt tolerance in rice, wheat, cotton, barley, alfalfa, and mustard.Citation3,Citation45 Moreover, higher stress level and low ROS scavenging capacity of the sensitive variety were found when compared to the tolerant one.Citation46 In the present study, we found that activities of CAT and POD in C24, C34 were greater than in Nona Bokra seedlings under different conditions. Stronger antioxidant enzymes of rice seedlings avoid ROS accumulation more efficiently, consequently confer those seedlings more tolerance to stress. Taken together, these findings highlight the effect of accumulation of antioxidants, such as CAT and POD to remove overextended ROS accumulation, which followed by less membrane system damage during salt stress and to maintain water potential underlie the greater tolerance of C34 and C24 to salt stress.
In conclusion, our results revealed that Lei-Qiong rice landraces, C24 and C34, could possess novel salt-tolerant trait-related genes, controlling some specific biological processes, such as antioxidant enzyme expression, by which they performed better in tolerating salt stress, when compared with Nona Bokra. Indeed, further inspection is necessary and worthy to deeply uncover the salt-tolerant related genomic information and their regulatory mechanism on these rice landraces.
Methods
Plant materials, growth conditions, stress treatments, and phenotyping
Three local rice landraces (C24, C34, and HG1) and Two rice genotypes, Oryza sativa L. ssp. Indica (Nona Bokra and IR29) were used in this study. Seeds were surface sterilized by incubation for 24 h in 3% H2O2, then washed thoroughly with distilled water. The sterilized seeds were germinated on moist tissue paper. For analysis of salt tolerance during germination stage, all rice species were put in distilled water with 50 mM,100 mM,150 mM,200 mM, 250 mM, and 300 mM NaCl, respectively, and cultured for 10 d and germination rates were recorded every 2-d interval. In other experiments, the germinated seedlings without treatment were transferred for further growth with 1/4 strength Hoagland solution. This hydroponic experiment was carried out in growth room with 26°C–-28°C and 16 h photoperiod. For seedling stage phenotyping, about 3-weekold seedlings were transferred to 1/4 strength Hoagland solution supplemented with 0 mM, 80 mM, 100 mM, 120 mM, and 150 mM NaCl, respectively. Plant height, root length, water content, and chlorophyll content were measured after culture for 10 d. For physiological experiments, seedlings were treated with 100 mM NaCl for 5 and 10 d, respectively. Method for each measurement will be described below.
Measurement of chlorophyll concentration
To determine chlorophyll concentration, seedling leaves were harvested, weighed, and extracted by incubating with acetone (80% v/v) at 4°C overnight. Absorbance (A) of the supernatant was recorded at wavelengths of 663 and 645 nm. The total chlorophyll concentration was calculated as chlorophyll content (mg/g) = (8.02A663 + 20.21A645) × v80% acetone/w leaves/1000.
Proline measurement
About 0.5 g of plant material was homogenized in 2 ml of 3% aqueous sulfosalicylic acid, and then centrifuged at 4000 rpm for 20 min. One milliliter of supernatant was reacted with 1 ml ninhydrin reagent and 1 ml of 6 M phosphoric acid in a test tube for 1 h at 100°C, and the reaction terminated in an ice bath. The reaction mixture was extracted with 3 ml toluene by mixing vigorously for 15–20 s. The chromophore containing toluene was aspirated from the aqueous phase, the absorbance read at 520 nm using toluene for a blank. The proline concentration was determined from a standard curve and calculated on a fresh weight basis following the formula: μg proline/ml × mltoluene × (Volume of extract/Volume of measure)/115.5 μg/μmol/gsample = μmolproline/g of fresh weight material.Citation43
MDA measurement
The method for measuring MDA content was modified from the method reported by Zhang et al.Citation47 Briefly, the leaves of rice seedlings were weighed and homogenized in 1.5 ml of 20% TCA solution. The homogenate was centrifuged, and the supernatant was added to 0.5% thiobarbituric acid. The mixture was incubated in boiling water for 15 min, and the reaction was stopped in an ice bath. Then, the samples were centrifuged and the absorbance of the supernatant was measured at 450, 532, and 600 nm. MDA contents (μmol.g−1 fresh weight) were calculated by the following formula: [6.45(A532 – A600) – 0.56A450] × Volume of extract /fresh weight.
Determination of peroxidase, superoxide dismutase, and catalase activity
A kit purchased from Jiancheng Company (Nanjing, China) was used in this experiment. Briefly, 0.5 g of plant material was homogenized in 4.5 ml of 0.1 M, pH 7.4 Phosphate Buffer Solution, centrifuged at 3500 rpm for 10 min. Supernatant was harvested for further usage following the protocol of the kit.
Statistical analysis
All data were analyzed by the analysis of variance using the SPSS statistical software and Excel. Significant differences were evaluated using single-factor variance analysis.
Disclosure of Potential Conflicts of Interest
No potential conflicts of interest were disclosed.
Additional information
Funding
References
- Godfray HC, Beddington JR, Crute IR, Haddad L, Lawrence D, Muir JF, Pretty J, Robinson S, Thomas SM, Toulmin C. Food security: the challenge of feeding 9 billion people. Science. 2010;327(5967):1–9. doi:10.1126/science.1185383.
- Tilman D, Balzer C, Hill J, Befort BL. Global food demand and the sustainable intensification of agriculture. Proc Natl Acad Sci U S A. 2011;108(50):20260–20264. doi:10.1073/pnas.1116437108.
- Li Q, Yang A, Zhang WH. Comparative studies on tolerance of rice genotypes differing in their tolerance to moderate salt stress. BMC Plant Biol. 2017;17(1):141. doi:10.1186/s12870-017-1089-0.
- Wang W, Vinocur B, Altman A. Plant responses to drought, salinity and extreme temperatures: towards genetic engineering for stress tolerance. Planta. 2003;218(1):1–14. doi:10.1007/s00425-003-1105-5.
- Walia H, Wilson C, Condamine P, Liu X, Ismail AM, Zeng L, Wanamaker SI, Mandal J, Xu J, Cui X, et al. Comparative transcriptional profiling of two contrasting rice genotypes under salinity stress during the vegetative growth stage. Plant Physiol. 2005;139(2):822–835. doi:10.1104/pp.105.065961.
- Chauhan PS, Lata C, Tiwari S, Chauhan AS, Mishra SK, Agrawal L, Chakrabarty D, Nautiyal CS. Transcriptional alterations reveal Bacillus amyloliquefaciens-rice cooperation under salt stress. Sci Rep. 2019;9(1):11912. doi:10.1038/s41598-019-48309-8.
- Cui LG, Shan JX, Shi M, Gao JP, Lin HX. DCA1 acts as a transcriptional co-activator of DST and contributes to drought and salt tolerance in rice. PLoS Genet. 2015;11(10):e1005617. doi:10.1371/journal.pgen.1005617.
- Hasegawa PM, Bressan RA, Zhu JK, Bohnert HJ. Plant cellular and molecular responses to high salinity. Annu Rev Plant Physiol Plant Mol Biol. 2000;51(1):463–499. doi:10.1146/annurev.arplant.51.1.463.
- Munns R, Tester M. Mechanisms of salinity tolerance. Annu Rev Plant Biol. 2008;59(1):651–681. doi:10.1146/annurev.arplant.59.032607.092911.
- Singh V, Singh AP, Bhadoria J, Giri J, Singh J, Vineeth TV, Sharma PC. Differential expression of salt-responsive genes to salinity stress in salt-tolerant and salt-sensitive rice (Oryza sativa L.) at seedling stage. Protoplasma. 2018;255(6):1667–1681. doi:10.1007/s00709-018-1257-6.
- Moradi F, Ismail AM. Responses of photosynthesis, chlorophyll fluorescence and ROS-scavenging systems to salt stress during seedling and reproductive stages in rice. Ann Bot. 2007;99(6):1161–1173. doi:10.1093/aob/mcm052.
- Aoki K, Ideguchi T, Kakuno T, Yamashita J, Horio T. Effects of NaCl and glycerol on photosynthetic oxygen-evolving activity with thylakoid membranes from halophilic green alga Dunaliella tertiolecta. J Biochem. 1986;100(5):1223–1230. doi:10.1093/oxfordjournals.jbchem.a121827.
- Zhao KF, Song J, Fan H, Zhou S, Zhao M. Growth response to ionic and osmotic stress of NaCl in salt-tolerant and salt-sensitive maize. J Integr Plant Biol. 2010;52(5):468–475. doi:10.1111/()1744-7909.
- Van Andel T, Veltman MA, Bertin A, Maat H, Polime T, Hille Ris Lambers D, Tjoe Awie J, De Boer H, Manzanilla V. Hidden rice diversity in the guianas. Front Plant Sci. 2019;10:1161. doi:10.3389/fpls.2019.01161.
- Pei ZM, Murata Y, Benning G, Thomine S, Klusener B, Allen GJ, Grill E, Schroeder JI. Calcium channels activated by hydrogen peroxide mediate abscisic acid signalling in guard cells. Nature. 2000;406(6797):731–734. doi:10.1038/35021067.
- Song Y, Miao Y, Song CP. Behind the scenes: the roles of reactive oxygen species in guard cells. New Phytol. 2014;201(4):1121–1140. doi:10.1111/nph.12565.
- Kwiecien S, Jasnos K, Magierowski M, Sliwowski Z, Pajdo R, Brzozowski B, Mach T, Wojcik D, Brzozowski T. Lipid peroxidation, reactive oxygen species and antioxidative factors in the pathogenesis of gastric mucosal lesions and mechanism of protection against oxidative stress - induced gastric injury. J Physiol Pharmacol. 2014;65:613–622.
- RoyChoudhury A, Roy C, Sengupta DN. Transgenic tobacco plants overexpressing the heterologous lea gene Rab16A from rice during high salt and water deficit display enhanced tolerance to salinity stress. Plant Cell Rep. 2007;26(10):1839–1859. doi:10.1007/s00299-007-0371-2.
- Deinlein U, Stephan AB, Horie T, Luo W, Xu G, Schroeder JI. Plant salt-tolerance mechanisms. Trends Plant Sci. 2014;19(6):371–379. doi:10.1016/j.tplants.2014.02.001.
- Singh J, Singh V, Sharma PC. Elucidating the role of osmotic, ionic and major salt responsive transcript components towards salinity tolerance in contrasting chickpea (Cicer arietinum L.) genotypes. Physiol Mol Biol Plants. 2018;24(3):441–453. doi:10.1007/s12298-018-0517-4.
- Wu D, Cai S, Chen M, Ye L, Chen Z, Zhang H, Dai F, Wu F, Zhang G. Tissue metabolic responses to salt stress in wild and cultivated barley. PLoS One. 2013;8(1):e55431. doi:10.1371/journal.pone.0055431.
- Malekzadeh P. Influence of exogenous application of glycinebetaine on antioxidative system and growth of salt-stressed soybean seedlings (Glycine max L.). Physiol Mol Biol Plants. 2015;21(2):225–232. doi:10.1007/s12298-015-0292-4.
- Nounjan N, Chansongkrow P, Charoensawan V, Siangliw JL, Toojinda T, Chadchawan S, Theerakulpisut P. High performance of photosynthesis and osmotic adjustment are associated with salt tolerance ability in rice carrying drought tolerance QTL: physiological and co-expression network analysis. Front Plant Sci. 2018;9:1135. doi:10.3389/fpls.2018.01135.
- Mishra P, Bhoomika K, Dubey RS. Differential responses of antioxidative defense system to prolonged salinity stress in salt-tolerant and salt-sensitive Indica rice (Oryza sativa L.) seedlings. Protoplasma. 2013;250(1):3–19. doi:10.1007/s00709-011-0365-3.
- Zhu JK. Salt and drought stress signal transduction in plants. Annu Rev Plant Biol. 2002;53(1):247–273. doi:10.1146/annurev.arplant.53.091401.143329.
- Razzaque S, Elias SM, Haque T, Biswas S, Jewel G, Rahman S, Weng X, Ismail AM, Walia H, Juenger TE, et al. Gene expression analysis associated with salt stress in a reciprocally crossed rice population. Sci Rep. 2019;9(1):8249. doi:10.1038/s41598-019-44757-4.
- Dong S, Wu L, Long F, Wu Q, Liu X, Pei H, Xu K, Lu Y, Wang Y, Lin Y, et al. The prevalence and distribution of Burkholderia pseudomallei in rice paddy within Hainan, China. Acta Trop. 2018;187:165–168. doi:10.1016/j.actatropica.2018.08.007.
- Du YD, Shen P, Wang H, Tang XR, Zhao H. Impacts of climate change on climatic division for double cropping rice in Guangdong Province, China. Ying Yong Sheng Tai Xue Bao. 2018;29(12):4013–4021. doi:10.13287/j.1001-9332.201812.041.
- Lin HX, Zhu MZ, Yano M, Gao JP, Liang ZW, Su WA, Hu XH, Ren ZH, Chao DY. QTLs for Na+ and K+ uptake of the shoots and roots controlling rice salt tolerance. Theor Appl Genet. 2004;108(2):253–260. doi:10.1007/s00122-003-1421-y.
- Conway G, Toenniessen G. Feeding the world in the twenty-first century. Nature. 1999;402(S6761):C55–58. doi:10.1038/35011545.
- Zhang F, Jiang YZ, Yu SB, Ali J, Paterson AH, Khush GS, Xu JL, Gao YM, Fu BY, Lafitte R, et al. Three genetic systems controlling growth, development and productivity of rice (Oryza sativa L.): a reevaluation of the ‘Green Revolution’. Theor Appl Genet. 2013;126(4):1011–1024. doi:10.1007/s00122-012-2033-1.
- Baillo EH, Kimotho RN, Zhang Z, Xu P. Transcription factors associated with abiotic and biotic stress tolerance and their potential for crops improvement. Genes (Basel). 2019;10(10):771. doi:10.3390/genes10100771.
- Zhang Y, Fang J, Wu X, Dong L. Na+/K+ balance and transport regulatory mechanisms in weedy and cultivated rice (Oryza sativa L.) under salt stress. BMC Plant Biol. 2018;18(1):375. doi:10.1186/s12870-018-1586-9.
- Takeuchi K, Gyohda A, Tominaga M, Kawakatsu M, Hatakeyama A, Ishii N, Shimaya K, Nishimura T, Riemann M, Nick P, et al. RSOsPR10 expression in response to environmental stresses is regulated antagonistically by jasmonate/ethylene and salicylic acid signaling pathways in rice roots. Plant Cell Physiol. 2011;52(9):1686–1696. doi:10.1093/pcp/pcr105.
- Serrano N, Ling Y, Bahieldin A, Mahfouz MM. Thermopriming reprograms metabolic homeostasis to confer heat tolerance. Sci Rep. 2019;9(1):181. doi:10.1038/s41598-018-36484-z.
- Jing W, Deng P, Cao C, Zhang W. Fine mapping of qSKC-1, a major quantitative trait locus for shoot K+ concentration, in rice seedlings grown under salt stress. Breed Sci. 2017;67(3):286–295. doi:10.1270/jsbbs.16190.
- Ren Y, Wang W, He J, Zhang L, Wei Y, Yang M. Nitric oxide alleviates salt stress in seed germination and early seedling growth of pakchoi (Brassica chinensis L.) by enhancing physiological and biochemical parameters. Ecotoxicol Environ Saf. 2019;187:109785. doi:10.1016/j.ecoenv.2019.109785.
- Flowers TJ, Munns R, Colmer TD. Sodium chloride toxicity and the cellular basis of salt tolerance in halophytes. Ann Bot. 2015;115(3):419–431. doi:10.1093/aob/mcu217.
- Vysotskaya L, Hedley PE, Sharipova G, Veselov D, Kudoyarova G, Morris J, Jones HG. Effect of salinity on water relations of wild barley plants differing in salt tolerance. AoB Plants. 2010;2010:plq006. doi:10.1093/aobpla/plq006.
- Djanaguiraman M, Prasad PV, Seppanen M. Selenium protects sorghum leaves from oxidative damage under high temperature stress by enhancing antioxidant defense system. Plant Physiol Biochem. 2010;48(12):999–1007. doi:10.1016/j.plaphy.2010.09.009.
- Kordrostami M, Rabiei B, Kumleh HH. Different physiobiochemical and transcriptomic reactions of rice (Oryza sativa L.) cultivars differing in terms of salt sensitivity under salinity stress. Environ Sci Pollut Res Int. 2017;24(8):7184–7196. doi:10.1007/s11356-017-8411-0.
- Chen Z, Cuin TA, Zhou M, Twomey A, Naidu BP, Shabala S. Compatible solute accumulation and stress-mitigating effects in barley genotypes contrasting in their salt tolerance. J Exp Bot. 2007;58(15–16):4245–4255. doi:10.1093/jxb/erm284.
- Yang A, Dai X, Zhang WH. A R2R3-type MYB gene, OsMYB2, is involved in salt, cold, and dehydration tolerance in rice. J Exp Bot. 2012;63(7):2541–2556. doi:10.1093/jxb/err431.
- Zhang M, Smith JA, Harberd NP, Jiang C. The regulatory roles of ethylene and reactive oxygen species (ROS) in plant salt stress responses. Plant Mol Biol. 2016;91(6):651–659. doi:10.1007/s11103-016-0488-1.
- Singh J, Singh V, Vineeth TV, Kumar P, Kumar N, Sharma PC. Differential response of Indian mustard (Brassica juncea L., Czern and Coss) under salinity: photosynthetic traits and gene expression. Physiol Mol Biol Plants. 2019;25(1):71–83. doi:10.1007/s12298-018-0631-3.
- Guan Q, Wang Z, Wang X, Takano T, Liu S. A peroxisomal APX from Puccinellia tenuiflora improves the abiotic stress tolerance of transgenic Arabidopsis thaliana through decreasing of H2O2 accumulation. J Plant Physiol. 2015;175:183–191. doi:10.1016/j.jplph.2014.10.020.
- Zhang L, Tian LH, Zhao JF, Song Y, Zhang CJ, Guo Y. Identification of an apoplastic protein involved in the initial phase of salt stress response in rice root by two-dimensional electrophoresis. Plant Physiol. 2009;149(2):916–928. doi:10.1104/pp.108.131144.