ABSTRACT
High salinity and waterlogging are two stress factors that often occur simultaneously in nature, particularly during the rainy season in the Yellow River Delta (YRD) of China. An attractive approach to improve the saline-alkali soil produced by waterlogging and high salt is to use plants for wetland ecosystem restoration. In this work, we examined the ecological adaptability of Elaeagnus angustifolia L. under combined waterlogging and salt stress, to evaluate the potential of this species for introduction to the YRD. We monitored the effects of salt plus waterlogging co-stress on the anatomy, physiology, and enzymatic systems in E. angustifolia seedlings. Salt alone and waterlogging alone inhibited the growth of the seedlings, while salt plus waterlogging co-stress reduced this growth inhibition. Furthermore, E. angustifolia seedlings resisted the salt plus waterlogging co-stress by increasing porosity, accumulating more inorganic ions and organic solutes, and increasing antioxidant enzyme activities to maintain high photosynthetic rates and membrane stability and thus avoid damage. These findings support the inclusion of E. angustifolia in the ecological restoration of the YRD.
Introduction
The process of salinization involves the destruction of soil due to the accumulation of water-soluble salts that can reach such a high level that they hinder not only agricultural production but also economic welfare and environmental health.Citation1,Citation2 In recent years, salinization has become a serious problem, and greater than 950 million hectares of soil throughout the world have excessive levels of salt.Citation3,Citation4 A total of 10.5% of the land damaged by salt occurs north of the Yangtze River in the coastal areas, northwest interior, and northeast of China.Citation4 Salt stress impairs plant development and growth, restricts afforestation, and results in losses in agricultural production.Citation5,Citation6 The problem is compounded by irrigation that is poorly managed, resulting in the additional salinization of productive farmland and the additional loss of the remaining non-saline soil that can be used to grow crops.Citation7 Simultaneously, much of the saline soil in the world is also prone to becoming waterlogged, i.e., saturated, due to shallow water-tables and concentrated rainfall during the rainy seasons.Citation8,Citation9 Waterlogging leads to the secondary salinization of land and results in low oxygen concentrations (hypoxia) in soils due to the poor oxygen solubility in the water and the low diffusion rate of oxygen in pores filled with water. Oxygen diffuses through such soil pores at a rate approximately 10,000-fold less than through soil pores filled with gas.Citation10 Notably, the responses of plants to water and salt stress have features in common. As the uptake of water by plants is reduced during high salinity, the suite of metabolic changes induced by salinity stress shares similarities with those due to water stress alone, including a reduction in their growth rate.Citation11
Compared to other regions in the world, the Yellow River Delta (YRD) has a high level of interaction between the land and ocean. This region is often referred to as the “Golden Triangle” because enormous quantities of sediment, including soil from central China’s Loess Plateau that is highly fertile, are transferred to the river mouth with the result that the YRD expands at a rate of 1000–2000 hectares each year.Citation12,Citation13 This transfer has led to the degradation of the freshwater wetlands of the YRD, and both the soil quality and biodiversity of this area have been threatened by the increase in stress from salt.Citation14,Citation15 The plants that grow here are often subjected to double stress from salinity and waterlogging due to the high concentrations of salt in the soil and the concentrated rainfall. The general effects that salinity and waterlogging have on many plant species have been clearly documented.Citation16–Citation20 However, the combination of high salt and waterlogging has not been fully elucidated. Identifying plants that are resistant to salt and waterlogging and then introducing them to the increasing saline regions of the YRD could be one way to improve the ecology, which is necessary to reclaim this area for agriculture.
Elaeagnus angustifolia L. (oleaster or Russian olive) is a small tree or deciduous shrubCitation12 that can tolerate high salt and also grow well in environments in which the salt content is less than 1.0%.Citation21 It can successfully complete its life cycle in an environment with a salt content of 0.4–1.2%.Citation22 The species is grown in the arid regions of northwest China, which also demonstrates its drought resistance. In addition to its resilience to adverse environmental conditions, the species has abundant roots that are infected with hyphae, resulting in its ability to fix nitrogen from the atmosphere.Citation23 Previous research by Asia,Citation23 has indicated that E. angustifolia can increase the levels of carbon (19%), organic nitrogen (21%) and phosphorus available to plants (74%) in the soil. Improving the soil benefits plant growth and aids in the ecological restoration of the saline soil of the YRD, which is too damaged to sustain plant growth. These qualities of the species have resulted in its status as the first choice for planting in arid and saline regions.Citation12 E. angustifolia is also a candidate plant for improving saline-alkali land because of its resistance to salinity, drought, and barren conditions. However, the ability of the species to tolerate waterlogging or the combination of waterlogging and salinity stress has not been evaluated.
In this study, E. angustifolia seedlings were treated with high salt, waterlogging, and the combination of salt plus waterlogging. Its physiological characteristics were measured, and E. angustifolia seedlings treated with a combination of salt and waterlogging grew at higher rates when compared with plants subjected solely to waterlogging. These findings suggest that E. angustifolia may be an appropriate primary species for ecological restoration and reclamation of the YRD.
Materials and methods
Plant material and growth conditions
Seeds of E. angustifolia that were collected from Xinjiang Turpan, China, were soaked for 3–5 days in water and then transplanted into plastic pots containing rinsed river sand. The seedlings were cultured at 24 ± 3°C under a light intensity of 600 μmol m–2 s–1 (16 h light, 8 h dark) and 70% relative humidity from germination as they developed to the first true leaf stage. The seedlings were watered with 1/2 Hoagland solution after germination.
Salinity and waterlogging treatments
The four-leaf stages of uniform seedlings were subjected to the stress treatments. The seedlings were treated with solely NaCl, solely waterlogging, and a combination of NaCl and waterlogging. For both treatments that involved waterlogging, the liquid level was higher than the sand surface by 1–1.5 cm. For the NaCl treatments, the seedlings were treated with 0 and 0.6% NaCl by watering with 1/2 Hoagland nutrient solution containing the varying amounts of NaCl. The concentration of NaCl was increased 0.2% each day until the indicated concentration was reached to prevent osmotic shock. A nutrient solution containing NaCl was supplied to the sand daily to prevent the accumulation of salt due to evaporation. The treatments were terminated two weeks after the final salinity concentration was reached. Five replicates (Five replicates mean five pots and there are around 7 seedlings per pot) were performed for each treatment.
Morphological characterization
All the indicators of growth were measured at the termination of treatment for each seedling. These included measurements from the surface of the sand to the top of the primary stem for the plant height along with the dry and fresh weights. One replicate was composed of the mean value of 7 plants per pot.Citation12,Citation24
The plant tissue (main root) was fixed with a solution of formalin-acetic-alcohol (FAA), followed by dehydration with increasing ethanol concentrations (70%, 95% and 100%) and embedded paraffin. Five-millimeter sections were prepared using a rotation microtome (Jung, Heidelberg, Germany) as described by Hahn et al.Citation25 Sections (aerenchyma and vessels) were stained with toluidine blue and monitored using a Zeiss microscope (Carl Zeiss, Heideaham, Germany).Citation26
Photosynthetic parameters
Chlorophyll content
Fresh leaves from the third to the fifth expanded leaf from the bottom of the primary stem were compressed in 5 mL of 80% dimethyl sulfoxide (DMSO) and acetone. The mixtures were vortexed and incubated at 65°C until the leaf tissue became white. The sample was then centrifuged for 10 minutes at 1,000 rpm. The chlorophyll content in the supernatant was measured by monitoring the absorbance at 645 and 663 nm using a Shimadzu UV spectrophotometer (UV-120-02, Kyoto, Japan). The concentrations of chlorophyll a and chlorophyll b were calculated as described by Porra et al.:Citation27
Chl a = 12.7 A663 – 2.69 A645
Chl b = 22.9 A645 – 4.68 A663
The total chlorophyll content was calculated as shown below:
Total Chl = Chl a+ Chl b = 8.02 × A663 + 20.21 × A645
Photosynthetic parameters
The net photosynthetic rate (Pn), stomatal conductance (Gs), intercellular CO2 concentration (Ci), and transpiration rate (Tr) were measured using a Portable Photosynthesis System CIRAS-2 (CIRAS-COR Inc., PP-Systems, Hitchin, UK). All the parameters were measured between 10:00 AM and 11:00 AM under conditions of photosynthetically active irradiation of saturated light intensity (1,000 µmol·m–2·s–1), relative humidity of 60 ± 5%, a leaf temperature of 30 ± 2°C, and 390 µmol (CO2) mol–1 (Ca).Citation28
Chlorophyll fluorescence
The maximal quantum yield of the PSII photochemistry was determined using a portable fluorometer (FMS-2, Hansatech, King’s Lynn, UK). The maximal quantum yield of the PSII photochemistry was expressed as Fv/Fm = (Fm–F0)/Fm. The effective quantum yield of the PSII photochemistry (ФPSII) was calculated as (Fm’–Fs)/Fm’. The seedlings were adapted to darkness for at least 30 min before the F0 measurement. The seedlings were subjected to a 2.5 second pulse of 17,000 µmol·m–2·s–1 light to measure the Fm. The seedlings were then maintained in daylight (600 µmol·m–2 · s–1) at a minimum of 30 min before the Fs was determined. Finally, the Fm’ was calculated after a 2.5 s pulse of 17,000 µmol·m–2·s–1 light.Citation29,Citation30
Measurement of physiological and biochemical factors
Na+ and K+ contents
A total of 0.5 g of leaf tissue was extracted with boiling distilled water and the solution was filtered. The filtrate was brought to 15 mL using deionized water.Citation31 The contents of K+ and Na+ were determined using flame photometer (Model 2655–00 Digital Flame Analyzer, Cole-Parmer Instrument Company, Chicago, USA).)
Osmotic potential
The leaves were incubated at −80 ºC for one hour, and the tissue fluid was extruded with a syringe. The tissue fluid was centrifuged at 30,000 x g for one minute. The supernatant was filtered, and the leaf osmotic potential (Ψs) (50 µL supernatant) was immediately measured using a frozen Fiske 210 osmotic pressure meter (Advanced Instruments Inc., Norwood, MA, USA).Citation32
Organic solute determination
2 g of frozen leaves was ground using 10% and 5% acetic acid, and the concentrations of proline were determined using a Shimadzu spectrophotometer as described by Chen et al.Citation32
2 g of fresh leaves were extracted using boiling distilled water. The organic acids were assayed following filtration using the phenolphthalein-NaOH method described by Chen et al.Citation32
Dry leaves (equivalent to 2 g fresh leaves) were extracted at 80ºC using 80% ethanol. The concentration of total soluble sugars (SS) was measured following filtration using the anthrone colorimetric method.Citation33
The concentrations of protein were determined as described by Bradford,Citation34 using Bio-Rad reagents (Hercules, CA, USA) and BSA as the standard.
MDA content and membrane permeability
The content of malondialdehyde (MDA) in the leaves was determined as described by Buege and Aust.Citation35 The membrane permeability was determined using measurements of the electrolyte leakage rates (ELR).Citation36
Measurement of the activities of antioxidant enzymes
The activities of superoxide dismutase (SOD) and ascorbate peroxidase (APX) were determined as described by Liu et al.Citation37 The activity of APX was measured by monitoring the oxidation of ascorbate at 290 nm. The reaction mixture was composed of 0.5 mM ascorbate, 0.1 mM H2O2, and 10 μl enzyme in 50 mM potassium phosphate buffer (pH 7.0). The APX activity was expressed as 1 μM of ascorbate oxidized per min per g of fresh weight leaves. The reaction mixture for SOD was composed of 50 mM sodium phosphate buffer (pH 7.8), 0.1 mM EDTA, 77.12 μM NBT, 80.2 μM riboflavin, 130 mM L-methionine, and 10 μl enzyme. The components were incubated for 20 minutes, and the SOD activity was determined to be the amount of enzyme that inhibited the NBT reaction by 50%.
The activities of peroxidase (POD) were quantified as described by Abeles and Biles.Citation38 Briefly, the reaction mixture was composed of 4 mL of 0.2 mL of 20 mM benzidine and 0.4 mL of H2O2 (3%) combined with 30 μL enzyme in 0.2 M acetate buffer (pH 4.8), . The absorbance was monitored at 530 nm. The POD activity was defined as the oxidation of 1 μM of benzidine per min per gram fresh weight [Unit g−1 (FW)].
The glutathione reductase (GR) activity was assayed as described by Schaedle and Bassham.Citation39 The reaction mixture contained 475 ml of 3 mM MgCl2, 0.15 mM NADPH, and 0.5 mM glutathione in 50 mM Tris (pH 7.5) and 25 ml enzyme extract from the leaves. The GR activity was assayed by monitoring the oxidation of NADPH dependent on glutathione at 340 nm.
Statistical analysis
Five replicates were used, and all the values were the mean ± SD. The data were analyzed using SPSS 16.0 (SPSS Inc., Chicago, IL, USA). The significance of the treatment was determined using two-way analyzes of variance (ANOVA). The mean values that were denoted by the different lowercase letters were significantly different at P < .05 using Duncan′s multiple range test.
Results
The effects of the interaction of waterlogging and salinity on the growth of E. angustifolia seedlings
NaCl stress decreased the height of the E. angustifolia seedlings. Water stress also negatively affected the growth of the seedlings. However, the combination of moderate salinity (0.6% NaCl) and waterlogging reduced this inhibitory effect ().
Figure 1. Effects of NaCl, waterlogging and NaCl plus waterlogging co-stress on the growth of E. angustifolia seedlings. A photograph of E. angustifolia seedlings under NaCl, waterlogging and NaCl plus waterlogging co-stress (0, 0.6 NaCl) after two weeks of the indicated treatment.
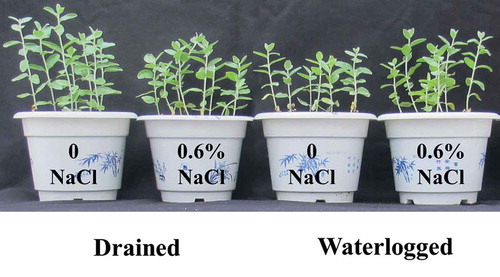
The height of the plants was reduced when treated with solely NaCl or waterlogging compared to the untreated controls ()). Plants subjected to a combination of 0.6% NaCl and waterlogging grew taller than plants that were solely subjected to waterlogging. The height of the plants treated solely with 0.6% NaCl and those treated with a combination of 0.6% NaCl and waterlogging did not differ significantly. Similarly, the fresh and dry weights of the plants treated with 0.6% NaCl alone and those treated with a combination of 0.6% NaCl and waterlogging did not differ significantly (,)).
Figure 2. Effects of NaCl, waterlogging, and NaCl plus waterlogging co-stress on plant growth. (a) plant height, (b) fresh weight, (c) dry weight. Values are means ± SD (n = 5). Values in a column followed by different lowercase letters are significantly different at P ≤ 0.05 according to Duncan′s multiple range test. A) plant height was measured from the sand surface to the top of the main stem. B) fresh weight is the fresh weight of whole seedlings. C) dry weight is the dry weight of whole seedlings.
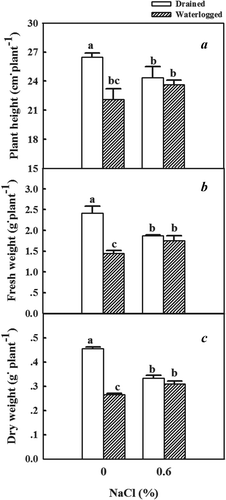
The effects of waterlogging and salinity co-stress on the aerenchyma of E. angustifolia seedlings
Anatomical responses
Root cross sections at the position of the first branch are shown in . Aerenchyma formation increased in plants subjected solely to waterlogging and those subjected to a combination of 0.6% NaCl and waterlogging compared to the untreated control plants. There was prominent aerenchyma formation in the plants subjected to solely waterlogging and those subjected to a combination of waterlogging and 0.6% NaCl, and the roots contained more metaxylem than those of the control. In addition, the roots developed an additional endodermal layer (). The number of vessels with a larger aperture in the plants subjected solely to waterlogging and those subjected to a combination of 0.6% NaCl plus waterlogging increased compared to those of the control plants (). This increase results in a stronger capacity for ventilation.
Table 1. The number of vessels of varying diameter of E. angustifolia main roots subjected to different treatments.
Figure 3. Effects of waterlogging and 0.6% NaCl plus waterlogging co-stress on root aerenchyma formation. Root cross sections were prepared at the position of the first branch of E. angustifolia. (a) A cross section of the primary root of an untreated control plant (Drained). (b) A cross section of the primary root of a plant kept under waterlogged conditions (Waterlogged) for two weeks. (c) A cross section of the primary root of a plant kept under 0.6% NaCl conditions (Drained with 0.6% NaCl) for two weeks. D) A cross section of the primary root of a plant kept under waterlogged conditions plus 0.6% NaCl stress (Waterlogged with 0.6% NaCl) for two weeks. Scale bar = 1.0 mm. Black arrows indicate the pith; Blue arrows indicate the vessels.
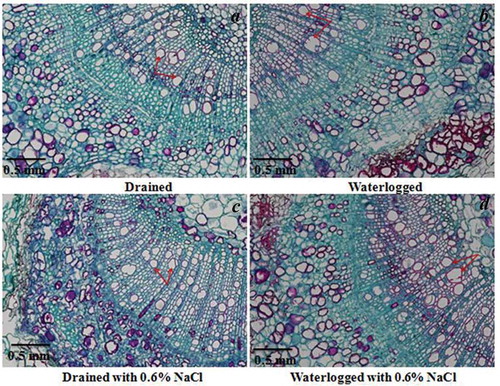
Porosity
There was no difference in the porosity of the leaves and stems in any of the treatments tested. However, the porosity of the root-stem junction and the roots of the E. angustifolia seedlings increased in the plants solely subjected to waterlogging and those subjected to waterlogging plus 0.6% NaCl compared to the plants solely treated with salt and the control untreated plants ()). This suggests that the treatment of solely waterlogging or the combination of 0.6% NaCl plus waterlogging can increase the porosity of the root-stem junction and the roots of the E. angustifolia seedlings.
Figure 4. Effects of NaCl, waterlogging, and NaCl plus waterlogging co-stress on the porosity of E. angustifolia seedlings. The sixth euthylla: the stem at the position of the sixth euthylla; The fourth euthylla: the stem at the position of the fourth euthylla; Cotyledon: the stem at the position of cotyledon; root-stem junction; The first root: the root at the position of the first branch. Values are means ± SD (n = 5). Values in a column followed by different lowercase letters are significantly different at P ≤ 0.05 according to Duncan′s multiple range test.
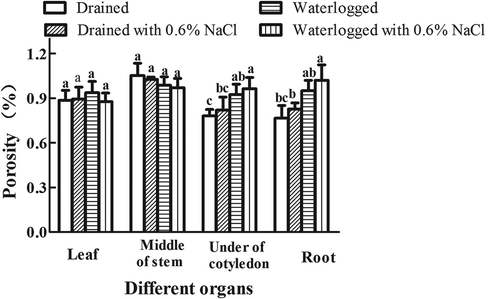
The effects of salinity plus waterlogging co-stress on photosynthesis of the E. angustifolia seedlings
Photosynthetic gas-exchange parameters
Pn, Tr, and Gs of the leaves of the E. angustifolia seedlings were reduced by treatment both solely with NaCl and solely with waterlogging (). Pn, Tr, and Gs of the E. angustifolia seedling leaves treated with the combination of 0.6% NaCl and waterlogging were higher than those treated solely with waterlogging ()). However, in these treatment conditions, the intercellular CO2 concentration (Ci) did not follow this trend ()). The Ci was higher in the leaves of the waterlogged plants compared to the controls that were untreated and drained. The Ci of both the drained and waterlogged plants treated with 0.6% NaCl did not differ compared with the untreated controls.
Figure 5. Effects of salinity, waterlogging, and NaCl plus waterlogging co-stress on photosynthesis. (a) net photosynthetic rate (Pn), (b) transpiration rate (Tr), (c) stomatal conductance (Gs), (d) intercellular CO2 concentration (Ci), (e) the maximal quantum yield of PSII photochemistry (Fv/Fm), (f) the effective quantum yield of PSII photochemistry (ФPSII), (g) Chlorophyll content and (h) photochemical quenching coefficient (qP) of expanded leaves of E. angustifolia seedlings treated for two weeks. Values are means ± SD (n = 5). For each variable, means with different lowercase letters are significantly different at P ≤ 0.05 according to Duncan’s multiple range test.
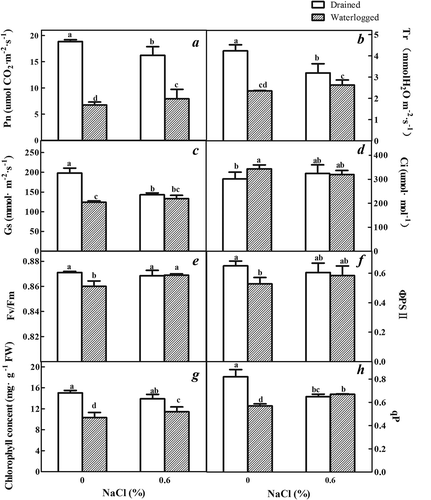
Photosynthetic pigments
The differences in the chlorophyll content ()) of the E. angustifolia seedling leaves subjected solely to NaCl or waterlogging, and a combination of NaCl and waterlogging were consistent with the difference observed for Pn.
Fv/Fm, ФPSII and qP
In the case of Fv/Fm ()), no difference was observed between the sole treatment with NaCl and the combination of NaCl plus waterlogging. However, the Fv/Fm was lower in the plants treated solely with waterlogging compared to all the other treatments. The changes in the ФPSII ()) of the E. angustifolia leaves of the plants subjected solely to NaCl or waterlogging, and a combination of NaCl and waterlogging were consistent with the Fv/Fm. The qP ()) decreased significantly in the plants treated solely with NaCl or waterlogging, and the combination of NaCl and waterlogging. However, the qP was lower in the plants treated solely with waterlogging compared to all of the other treatments. This suggests that a combination of moderate salinity (0.6% NaCl) plus waterlogging can increase the Fv/Fm, ФPSII and qP compared to treatment solely with waterlogging.
The effects of salinity plus waterlogging co-stress on inorganic ions in the E. angustifolia seedlings
Na+ is the primary toxic ion that is introduced by salt stress. The degree of salt injury to plants is often determined by the Na+/K+ ratio. A higher Na+/K+ ratio suggests a higher degree of stress induced by salt. The Na+ content of the roots and leaves increased under treatment solely with NaCl compared to that of the untreated plants. In the plants subjected to waterlogging, the content of Na+ in the leaves increased compared to the untreated controls, and the Na+ content of the roots decreased compared to that of the untreated controls (,)).
Figure 6. Effects of salinity, waterlogging, and NaCl plus waterlogging co-stress on inorganic ions. (a) Na+ content of expanded leaves, (b) K+ content of expanded leaves, (c) Root Na+ content, and (d) Root K+ content. All data are reflective of E. angustifolia seedlings treated for two weeks. Values are means ± SD (n = 5). Mean values with different lowercase letters are significantly different at P ≤ 0.05 according to Duncan′s multiple range test.
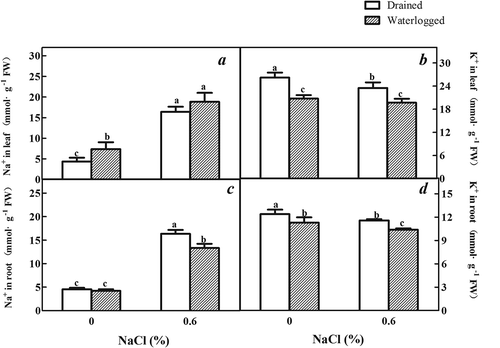
The content of K+ in the roots and leaves was lower than that in the plants treated solely with NaCl compared to the untreated controls. The K+ content in the roots was also lower in the waterlogged plants treated with NaCl compared to the plants solely treated by waterlogging (,)). In summary, the ratio of Na+/K+ in the leaves increased in the plants treated solely with NaCl compared to the untreated plants and those treated solely with waterlogging. This increase in the Na+/K+ ratio was lower in the plants subjected to 0.6% NaCl and waterlogging compared to those subjected solely to NaCl.
The effects of the combination of salinity and waterlogging treatments on organic material of the E. angustifolia seedling leaves
There was no difference in the soluble sugar, proline, protein, and organic acid contents of the plants maintained in waterlogged conditions compared to the drained control plants (). The soluble sugar, organic acids, protein, and proline contents of the leaves of the E. angustifolia seedlings increased in the plants subjected to 0.6% NaCl compared to the plants that remained untreated. There were significant increases in the proline, soluble sugar, organic acids, and protein contents in the leaves of the E. angustifolia seedlings exposed to the combination of waterlogging plus 0.6% NaCl compared to the drained plants subjected to the same NaCl concentration and the plants subjected solely to waterlogging.
Figure 7. Effects of salinity, waterlogging, and NaCl plus waterlogging co-stress on organic material. (a) Proline content, (b) Soluble sugar content, (c) Organic acids content (d) Protein content. All data are reflective of expanded leaves E. angustifolia seedlings treated for two weeks. Values are means ± SD (n = 5). Mean values with different lowercase letters are significantly different at P ≤ 0.05 according to Duncan′s multiple range test.
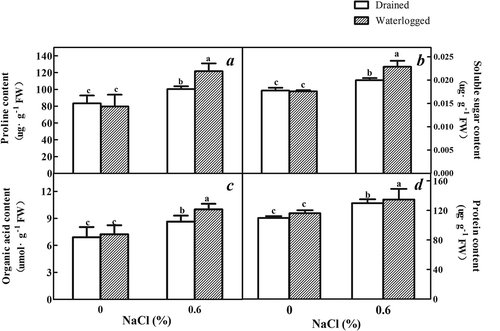
The effects of the combination of salinity and waterlogging stress on the osmotic potential of E. angustifolia seedlings
There was no difference in the osmotic potential between the drained control plants and those maintained in waterlogged conditions (). The osmotic potential was reduced in the plants treated solely with salt compared to that in the untreated control plants. The osmotic potential was lower in the plants subjected to 0.6% NaCl and waterlogging compared with the drained plants treated with the same concentration of NaCl.
Figure 8. Effects of salinity, waterlogging, and NaCl plus waterlogging co-stress on osmotic potential (Ψs). Expanded leaves of E. angustifolia seedlings treated for two weeks were used. Values are means ± SD (n = 5). Mean values with different lowercase letters are significantly different at P ≤ 0.05 according to Duncan′s multiple range test.
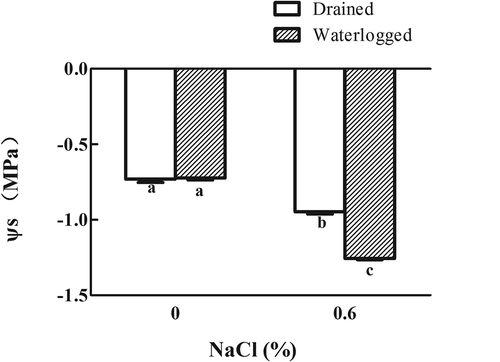
The effects of the combination of salinity and waterlogging stress on the antioxidant activities of E. angustifolia seedlings
The SOD, POD and GR activities of the E. angustifolia seedlings increased in plants treated with solely NaCl and solely waterlogging compared to the control (P ≤ 0.05). Treatment with a combination of NaCl and waterlogging induced an increase in the SOD, POD and GR activities that were higher than those of solely NaCl or waterlogging (,)).
Figure 9. Effects of salinity, waterlogging, and NaCl plus waterlogging co-stress on antioxidant enzyme activities. (a) SOD activity was determined by the nitrogen blue tetrazolium photoreduction method, (b) POD activity was determined by the guaiacol method, (c) APX activity was determined by ultraviolet absorption method, (d) GR activity was determined by monitoring the glutathione-dependent oxidation of NADPH at 340 nm. Values are means ± SD (n = 5). Expanded leaves of E. angustifolia seedlings were used. Mean values with different lowercase letters are significantly different at P ≤ 0.05 according to Duncan′s multiple range test.
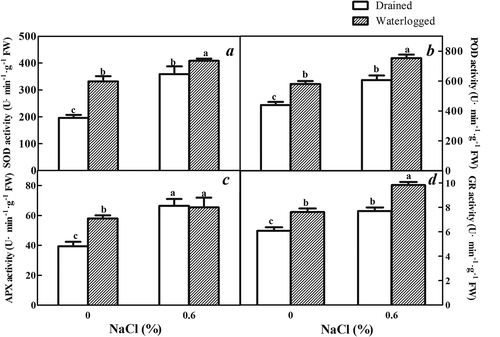
The APX activity of the E. angustifolia seedlings treated with solely waterlogging or NaCl or a combination of NaCl and waterlogging was higher than that of the untreated controls. However, unlike the activities of SOD, POD and GR, there was not a difference in the APX activity seedlings subjected solely to NaCl and those subjected to a combination of NaCl and waterlogging ()).
Discussion
High salinity and waterlogging severely affect plant growth in agricultural systems. These environmental factors initiate changes in signal transduction to produce biochemical and physiological responses. There are alterations in the expression of the genes responsible for these processes that have previously been documented.Citation40 The adaptation of plants to salt stress is complex, and both internal constitutive mechanisms for salt tolerance and external environmental factors affect their degree of adaptation.Citation41 Salinity can cause a reduction in plant growth due to its production of ion toxicity, osmotic stress, and nutritional stress.Citation7 E. angustifolia is a primary species for wetland restoration because it is tolerant of salinity-alkalinity and barren soil.Citation12 Therefore, it is critical to obtain an understanding of the physiological responses of E. angustifolia to the composite effects of salt and water table level stress.
In this study, treatment solely with salt and solely with waterlogging reduced the biomass and height of the E. angustifolia seedlings compared to the untreated plants. However, the combination of salinity and waterlogging stress treatments increased the biomass compared with the plants subjected solely to waterlogging. Similar results have been previously observed in Mentha pulegiumCitation20 and Mentha piperita.Citation19 The changes in biomass may be related to changes in the photosynthetic activity during stress responses.Citation7 The decrease in photosynthesis described in this study was caused by a decrease in stomatal conductance (Gs) and the transpiration rate (Tr) in the plants subjected solely to salt or waterlogging. Our results support the hypothesis that the changes in biomass are reflected by the changes in photosynthetic parameters in E. angustifolia seedlings ().
In general, abiotic stress has additional effects: it reduces the content of chlorophyll, damages the structure of chloroplasts, and decreases photosynthesis.Citation42 All of these results were corroborated in this study. However, the combination of 0.6% NaCl and waterlogging co-stress can reduce this damage (). These results suggest that in mild salt conditions, an appropriate water table level could improve plant photosynthesis more effectively than waterlogging treatment.
Kozlowski,Citation43showed that waterlogging causes premature leaf abscission and senescence, induces shoot dieback, suppresses leaf formation and the expansion of the leaves and internodes, and generally decreases cambial growth. However, waterlogging produced an increase in the growth parameters in the plants treated with salt. The cross sections of the roots in this study show an increase in the root vessel size and an increase in the diameter and porosity of the primary root when the plants were subjected to a combination of waterlogging and 0.6% NaCl compared to the untreated controls (). The development of the metaxylem in these treated roots may result in a more efficient flow of water and sap in the xylem.Citation43 The development of the metaxylem and the increase in the diameter of the stele and the vascular number are adaptive mechanisms to maintain higher levels of aeration and better water flow in E. angustifolia seedlings. According to Haddadi et al.,Citation41 the combination of subjecting the plants to salinity and waterlogging resulted in a greater induction of aerenchyma than that of plants subjected solely to waterlogging. The formation of aerenchyma in the plant organs is due to the degeneration of the cortex and subsequent cell death. The spaces that were formerly occupied by these cells serve to provide the voids filled with gas that facilitates the movement of oxygen.Citation44
Na+, the primary ion used for osmotic adjustment in plants, increased under treatment solely with salt and the combination of salt and waterlogging (). It was previously found that high concentrations of salt destroyed the selective permeability of the plasma membrane and the ionic balance in cells. This combination causes reduced development and growth, followed by death.Citation45 However, the accumulation of organic osmoregulation substances can decrease ion toxicity.Citation46 Proline, organic acid, soluble sugar, and protein contents were found at higher levels in the plants subjected to NaCl, and organic osmoregulation factors was observed at the highest concentration in the plants subjected to the combination of 0.6% salinity and waterlogging (). Proline may play a significant role during osmotic stress by maintaining the turgor and leaf osmotic potential during osmotic stress.Citation47 Proline could also serve to protect the stability of protein structures during dehydration. Previous research has indicated a positive relationship between the accumulation of proline and tolerance to osmotic stress.Citation48
In our study, antioxidant enzymes activity increased in the plants subjected to both NaCl and waterlogging. The highest enzyme activities were observed in the plants subjected to both 0.6% NaCl and waterlogging (). Abiotic stress can cause peroxides to form on the membrane lipids of higher plants, which increases the MDA content.Citation49 The findings of this study are consistent with this trend, since an increase in the MDA content was one of the observations of the plants subjected to stress (Fig. S1). Research on osmotic stress in M. pulegium identified similar results.Citation50,Citation51 Waterlogging plus treatment with 0.6% NaCl resulted in a decreased MDA content compared to salt treatment alone (Figure A1), which may be due to the increased oxidase activity. The lower MDA content indicates that fewer of the membrane lipids will be peroxidated and supports high membrane stability and low membrane relative conductivity (Figure A1) .Citation52
In summary, these results showed that for E. angustifolia plants, the combination of treatment with 0.6% NaCl and waterlogging increased the porosity, main root diameter, organic osmotic adjustment substances, and oxidase activities, while also resulting in a decreased MDA content, membrane relative conductivity, and osmotic potential. These responses allowed the E. angustifolia seedlings to avoid anoxia, reduce ion toxicity, and protected the function of biological macromolecules. Thus, treatment with a combination of 0.6% NaCl and waterlogging appears to promote photosynthesis and growth compared with waterlogging or salt treatments alone.
Authors’ Contribution Statement
Xiaojuan Liu and Chunxiao Chen wrote this manuscript; Chunxiao Chen and Xiaojuan Liu performed experiments; Yun liu, Yanlu Liu and Yang Zhao and collected data, carried out all analyses; Yanlu Liu and Yang Zhao were involved in preparing figures; Min Chen conceptualized the idea. All authors read and approved the final manuscript.
Disclosure statement
The authors declare that they have no competing interests.
Additional information
Funding
References
- Rengasamy P. World salinization with emphasis on Australia[J]. J Exp Bot. 2006;57(5):1–12. doi:10.1093/jxb/erj108.
- Shao Q, Han N, Ding T, Zhou F, Wang B. SsHKT1;1 is a potassium transporter of the C 3 halophyte Suaeda salsa that is involved in salt tolerance. Funct Plant Biol. 2014;41(8):790–802. doi:10.1071/FP13265.
- Feng ZT, Deng YQ, Zhang SC, Liang X, Yuan F, Hao JL, Zhang JC, Sun SF, Wang BS. K+ accumulation in the cytoplasm and nucleus of the salt gland cells of Limonium bicolor accompanies increased rates of salt secretion under NaCl treatment using NanoSIMS. Plant Sci. 2015;238:286–296. doi:10.1016/j.plantsci.2015.06.021.
- Han G, Wang M, Yuan F, Sui N, Song J, Wang B. The CCCH zinc finger protein gene AtZFP1 improves salt resistance in Arabidopsis thaliana. Plant Mol Biol. 2014;86(3):237–253. doi:10.1007/s11103-014-0226-5.
- Herrero J, Pérez-Coveta O. Soil salinity changes over 24 years in a Mediterranean irrigated district[J]. Geoderma. 2005;125(3–4):287–308. doi:10.1016/j.geoderma.2004.09.004.
- Rozema J, Flowers T. Ecology. Crops for a salinized world[J]. Science. 2008;322:1478–1480. doi:10.1126/science.1168572.
- Munns R, Tester M. Mechanisms of salinity tolerance[J]. Annu Rev Plant Biol. 2008;59(1):651–681. doi:10.1146/annurev.arplant.59.032607.092911.
- Ghassemi F, Jakeman AJ, Nix HA. Salinisation of land and water resources: human causes, extent, management and case studies. Sydney: University of New South Wales Press; 1995. 526 pp.
- Qureshi RH, Barrett-Lennard EG. Saline agriculture for irrigated land in Pakistan: a handbook. Canberra: Monograph No. 50, Australian Centre for International Agricultural Research; 1998. 142.
- Grable AR. Soil aeration and plant growth. Adv Agron. 1966;18:57.
- Munns R. Comparative physiology of salt and water stress[J]. Plant Cell Environ. 2002;25(2):239–250. doi:10.1046/j.0016-8025.2001.00808.x.
- Qi Y, Li J, Chen C, Li L, Zheng X, Liu J, Zhu T, Pang C, Wang B, Chen M. Adaptive growth response of exotic Elaeagnus angustifolia L. to indigenous saline soil and its beneficial effects on the soil system in the Yellow River Delta, China. Trees. 2018;32(6):1723–1735. doi:10.1007/s00468-018-1746-4.
- Wang ZY, Liang ZY. Dynamic characteristics of the Yellow River mouth.[J]. Earth Surf Processes Landforms. 2015;25(7):765–782. doi:10.1002/1096-9837(200007)25:7<765::AID-ESP98>3.0.CO;2-K.
- Shan K. Theory, methodology and practices of wetland ecological restoration in yellow river delta nature reserve[J]. Wetland Sci Manage. 2007;3(4):16–20.
- Yu J, Li Y, Han G, Zhou D, Fu Y, Guan B, Wang G, Ning K, Wu H, Wang J. The spatial distribution characteristics of soil salinity in coastal zone of the Yellow River Delta[J]. Environ Earth Sci. 2014;72(2):589–599. doi:10.1007/s12665-013-2980-0.
- Bansal R, Srivastava JP. Erratum to: antioxidative defense system in pigeonpea roots under waterlogging stress[J]. Acta Physiologiae Plant. 2012;34(4):1595. doi:10.1007/s11738-012-0977-z.
- Lenssen JPM, Menting FBJ, Van Der Putten WH, Blom CWPM. Vegetative reproduction by species with different adaptations to shallow-flooded habitats.[J]. New Phytol. 2010;145(1):61–70. doi:10.1046/j.1469-8137.2000.00557.x.
- Gibbs J, Greenway H. Mechanisms of anoxia tolerance in plants. I. Growth, survival and anaerobic catabolism[J]. Funct Plant Biol. 2003;30(3):1–47. doi:10.1071/PP98095.
- Khorasaninejad S, Mousavi A, Soltanloo H, Hemmati K, Khalighi A. The effect of salinity stress on growth parameters, essential oil yield and constituent of peppermint (Mentha piperita L.).[J]. J Med Plants Res. 2010;5:5360–5365.
- Merati MJ, Hassanpour H, Niknam V, Mirmasoumi M. Exogenous application of penconazole regulates plant growth and antioxidative responses in salt-stressed[J]. J Plant Interact. 2014;9(1):791–801. doi:10.1080/17429145.2014.948084.
- Wang BS, Yao DY. Effects of salt stress on membrane permeability, membrane lipid peroxidation and SOD activity of Elaeagnus angustifolia L. [J]. J Hebei Agric Univ. 1993;16:20–24.
- Zhang BY, Cao ZY, Zhao KF. Studies on some physiological characteristics of Elaeagnus angustifolia L. under salt stress [J]. Sci Silvae Sinicae. 1992;2:187–189.
- Asia K, John PA, Paul LG. Nitrogen fixation by Elaeagnus angustifolia L. the reclamation of degraded croplands of Central Asia. Tree Physiol. 2009;520:1–10.
- Wang D, Guo JR, Liu XJ, Song J, Chen M, Wang BS. Effects of cultivation strategies on hybrid pennisetum yield in saline soil. Crop Sci. 2014;54(6):1–10. doi:10.2135/cropsci2013.11.0741.
- Hahn M, Vogel M, Delling G. Virchows Arch. A Pathol Anat. 1991;418(1):1–7. doi:10.1007/BF01600238.
- Li S, Chen M, Yu D, Ren S, Sun S, Liu L, Ketelaar T, Emons AMC, Liu CM. EXO70A1-mediated vesicle trafficking is critical for tracheary element development in Arabidopsis. Plant Cell. 2013;25(5):1774–1786. doi:10.1105/tpc.113.112144.
- Porra RJ, Thompson WA, Kriedemann PE. Determination of accurate extinction coefficients and simultaneous equations for assaying chlorophylls a, and b, extracted with four different solvents: verification of the concentration of chlorophyll standards by atomic absorption spectroscopy[J]. Biochimica et Biophysica Acta (BBA) - Bioenergetics. 1989;975(3):384–394. doi:10.1016/S0005-2728(89)80347-0.
- Lin J, Li JP, Yuan F, Yang Z, Wang BS, Chen M. Transcriptome profiling of genes involved in photosynthesis in Elaeagnus angustifolia L. under salt stress. Photosynthetica. 2018;56(4):998–1009. doi:10.1007/s11099-018-0824-6.
- Dongsansuk A, Lütz C, Neuner G. Effects of temperature and irradiance on quantum yield of PSII photochemistry and xanthophyll cycle in a tropical and a temperate species[J]. Photosynthetica. 2013;51(1):13–21. doi:10.1007/s11099-012-0070-2.
- Qiu N, Lu Q, Lu C. Photosynthesis, photosystem II efficiency and the xanthophyll cycle in the salt-adapted halophyte Atriplex centralasiatica.[J]. New Phytol. 2003;159(2):479–486. doi:10.1046/j.1469-8137.2003.00825.x.
- Song J, Feng G, Tian CY, Zhang FS. Osmotic adjustment traits of Suaeda physophora, Haloxylon ammodendron and Haloxylon persicum in field or controlled conditions[J]. Plant Sci. 2006;170(1):113–119. doi:10.1016/j.plantsci.2005.08.004.
- Chen M, Song J, Wang BS. NaCl increases the activity of the plasma membrane H+-ATPase in C3 halophyte Suaeda salsa callus. Acta Physiol Plant. 2010;32(1):27–30. doi:10.1007/s11738-009-0371-7.
- Zhang ZQ. Determination of the soluble sugars in plant materials. In: shanghai Society of plant physiology experimental handbook for plant physiology. Shanghai: Shanghai Science and Technology Press; 1985. p. 134–138. (in Chinese).
- Bradford MM. A rapid and sensitive method for the quantitation of microgram quantities of protein utilizing the principle of protein-dye binding. Anal Biochem. 1976;72(1–2):248–254. doi:10.1016/0003-2697(76)90527-3.
- Buege JA, Aust SD. Microsomal lipid peroxidation.[J]. Hoppe-Seyler’s Zeitschrift für physiologische Chemie. 1978;52:1604–1605.
- Lutts S, Kinet JM, Bouharmont J. NaCl-induced senescence in leaves of rice (Oryza sativa L.) cultivars differing in salinity resistance[J]. Ann Bot. 1996;78(3):389–398. doi:10.1006/anbo.1996.0134.
- Liu S, Wang W, Li M, Wan S, Sui N. Antioxidants and unsaturated fatty acids are involved in salt tolerance in peanut. Acta Physiol Plant. 2017;39(9):207–234. doi:10.1007/s11738-017-2501-y.
- Abeles FB, Biles CL. Characterization of peroxidases in lignifying peach fruit endocarp. Plant Physiol. 1991;95(1):269–273. doi:10.1104/pp.95.1.269.
- Schaedle M, Bassham JA. Chloroplast glutathione reductase. Plant Physiol. 1977;59(5):1011–1012. doi:10.1104/pp.59.5.1011.
- Andjelkovic V, Thompson R. Changes in gene expression in maize kernel in response to water and salt stress. Plant Cell Rep. 2006;25(1):71–79. doi:10.1007/s00299-005-0037-x.
- Haddadi BS, Hassanpour H, Niknam V. Effect of salinity and waterlogging on growth, anatomical and antioxidative responses in Mentha aquatica, L[J]. Acta Physiologiae Plant. 2016;38:1–11.
- Ma Q, Yue LJ, Zhang JL, Wu GQ, Bao AK, Wang SM. Sodium chloride improves photosynthesis and water status in the succulent xerophyte Zygophyllum xanthoxylum[J]. Tree Physiol. 2012;32(1):4–13. doi:10.1093/treephys/tpr098.
- Kozlowski TT. Responses of woody plants to flooding and salinity[J]. Flooding Plant Growth. 1984;1:129–163.
- Taiz L, Zeiger E. Plant Physiol. 2010 ;5:163–198.
- Tavakkoli E, Fatehi F, Coventry S, Rengasamy P, McDonald GK. Additive effects of Na+ and Cl– ions on barley growth under salinity stress[J]. J Exp Bot. 2011;62(6):2189. doi:10.1093/jxb/erq422.
- Silva EN, Ribeiro RV, Ferreira-Silva SL, Viégas RA, Silveira JAG. Comparative effects of salinity and water stress on photosynthesis, water relations and growth of Jatropha curcas plants.[J]. J Arid Environ. 2010;74(10):1130–1137. doi:10.1016/j.jaridenv.2010.05.036.
- Sadiqov ST, Akbulut M, Ehmedov V. Role of Ca 2+, in drought stress signaling in wheat seedlings[J]. Biochem Biokhimiia. 2002;67(4):491–499. doi:10.1023/A:1015298309888.
- Aly AA, Latif HH. Differential effects of paclobutrazol on water stress alleviation through electrolyte leakage, phytohormones, reduced glutathione and lipid peroxidation in some wheat genotypes (Triticum aestivum L.) grown in-vitro[J]. Rom Biotechnol Letters. 2011;16:6710–6721.
- Demiral T, Türkan I. Does exogenous glycinebetaine affect antioxidative system of rice seedlings under NaCl treatment?[J]. J Plant Physiol. 2004;161(10):1089–1100. doi:10.1016/j.jplph.2004.03.009.
- Hassanpour H, Khavari-Nejad RA, Niknam V, Najafi F, Razavi K. Effects of penconazole and water deficit stress on physiological and antioxidative responses in pennyroyal (Mentha pulegium, L.). Acta Physiologiae Plant. 2012;34(4):1537–1549. doi:10.1007/s11738-012-0952-8.
- Karray-Bouraoui N, Hamrouni-Maâzoul H, Rabhi M, Harbaoui F, Attia H, Oueslati S, Ksouri R, Lachaâl M. Enzymatic and non-enzymatic antioxidant responses of two Mentha pulegium provenances to salt stress.[J]. J Med Plant Res. 2010;4(23):2518–2524. doi:10.5897/JMPR10.461.
- Simova-Stoilova L, Demirevska K, Kingston-Smith A, Feller U. Involvement of the leaf antioxidant system in the response to soil flooding in two Trifolium genotypes differing in their tolerance to waterlogging[J]. Plant Sci. 2012;183(9):43. doi:10.1016/j.plantsci.2011.11.006.