ABSTRACT
ABA regulates abiotic stress tolerance in plants via activating/repressing gene expression. However, the functions of many ABA response genes remained unknown. C/VIFs are proteinaceous inhibitors of the CWI and VI invertases. We report here the involvement of C/VIF1 in regulating ABA response and salt tolerance in Arabidopsis. We found that the expression level of C/VIF1 was increased in response to ABA treatment. By using CRISPR/Cas9 gene editing, we generated transgene-free c/vif1 mutants. We also generated C/VIF1 overexpression plants by expressing C/VIF1 under the control of the 35S promoter. We examined ABA response of the 35S:C/VIF1 transgenic plants and the c/vif1 mutants by using seed germination and seedling greening assays, and found that the 35S:C/VIF1 transgenic plants showed an enhanced sensitivity to ABA treatment in both assays. On the other hand, the c/vif1 mutants showed slight enhanced tolerance to ABA only at the early stage of germination. We also found that salt tolerance was reduced in the 35S:C/VIF1 transgenic plants in seed germination assays, but slightly increased in the c/vif1 mutants. Taken together, our results suggest that C/VIF1 is an ABA response gene, and C/VIF1 is involved in the regulation of ABA response and salt tolerance in Arabidopsis.
Introduction
Abiotic stresses including drought, cold, salinity and extreme temperatures reduce the yield of crops and other plants.Citation1,Citation2 ABA (abscisic acid) is the key plant hormone that regulates plant responses to abiotic stresses.Citation3-Citation6 ABA signaling through the PYR1/PYL/RCAR (Pyrabactin resistance 1/PYR1-like/Regulatory component of ABA receptor) receptors,Citation7-Citation9 the PP2Cs (A-group PROTEIN PHOSPHATASE 2Cs) phosphatases,Citation10,Citation11 the SnRKs (NONFERMENTING 1 (SNF1)-RELATED PROTEIN KINASES) protein kinases,Citation12 and a few ABF/AREB/ABI5-type bZIP (basic region leucine zipper) transcription factorsCitation13-Citation15 regulate plant response to abiotic stresses via activating/repressing ABA response genes.Citation3,Citation5,Citation6,10-12 Consistent with this, mutation in the ABA singling genes affected plant tolerance to abiotic stresses. For example, overexpression of PYL genes enhanced drought tolerance in plants,Citation16,Citation17 whereas loss-of-function of SnRK genes or ABF/AREB/ABI5-type bZIP transcription factor genes led to reduced drought tolerance in plant.Citation18,Citation19 However, functions of a lot of ABA response genes in ABA signaling and/or plant abiotic stress responses remained unknown.
Cell wall invertase (CWI) and vacuolar invertase (VI) are acid invertases, they can irreversibly cleave sucrose, a primary form of carbon assimilates in the non-photosynthetic sink organs, into glucose and fructose.Citation20-Citation23 The glucose and fructose then serve as metabolites and nutrient sources of the plants, and as signaling molecules to modulate gene expression during plant growth and development as well as plant responding to environmental cues.Citation24,Citation25 As a result, up- or down-regulation of CWI and VI expression levels affected plant growth and development and/or plants response to stresses. For example, overexpression of CWI gene delayed leaf aging and enhanced drought tolerance in tobacco and tomato,Citation26,Citation27 suppression of VI gene decreased cold-induced sweetening in potato,Citation28,Citation29 and ectopic expression of a tea VI gene enhanced cold tolerance in Arabidopsis.Citation30
Activities of CWI and VI invertases are regulated by the low-molecular-weight proteinaceous inhibitors, cell wall/vacuolar inhibitor of fructosidases (C/VIFs), who can physically interact with CWI and VI invertases and inhibit their activities.Citation31-Citation33 Consistent with their biological functions, changes in the expression of C/VIF genes also affected plant growth and development and/or plants response to stresses. For example, silencing of CIF gene led to improved seed filling, prolonged leaf green, and enhanced cold tolerance in tomato,Citation34,Citation35 loss-of-function of CIF gene resulted in increased seed production and germination in Arabidopsis,Citation36 and ectopic expression of tobacco VIF enhanced drought tolerance in Arabidopsis.Citation37 However, the relationship between ABA and C/VIF inhibitors in regulating plant abiotic tolerance remained largely unclear.
We report here the roles of C/VIF1 in regulating ABA response and salt tolerance in Arabidopsis. We found that the expression level of C/VIF1 was upregulated by ABA, and transgenic plant overexpressing C/VIF1 showed enhanced sensitivity to ABA and reduced tolerance to salt.
Materials and methods
Plant materials and growth conditions
The Columbia-0 (Col) wild-type Arabidopsis (Arabidopsis thaliana) was used as a control for ABA sensitivity and salt tolerance assays, and for plant transformation to generate c/vif1 mutants and 35S:C/VIF1 transgenic plants.
For plant transformation, seeds of the Col wild type were germinated and grown in soil pots in a growth room. For total RNA isolation, ABA response and salt tolerance assays, seeds of the Col wild type, the 35S:C/VIF1 transgenic plants and the c/vif1 mutants were sterilized with 25% (v/v) bleach for 10 min, washed with sterilized water four times, and placed on vitamins (PlantMedia)-containing 1/2 MS (Murashige & Skoog) plates. The medium contains 1% (w/v) sucrose and was solidified with 0.6% (w/v) phytoagar (PlantMedia). The plates were kept in darkness at 4°C for 2 d and then transferred to a growth room.
The growth conditions in the growth room were set at 22°C, and a 16 h light/8 h dark cycle with photon density at ~125 μmol m−2 s−1.
ABA treatment, RNA isolation and RT-PCR
To examine the expression of C/VIF1 and C/VIF2 in response to ABA, 7-day-old Col wild-type seedlings were treated with 50 μM ABA or solvent methanol as a control for 4 h, frozen in liquid N2. Total RNA was then isolated from the seedlings, and cDNA was synthesized as previously described.Citation38 RT-PCR was used to examine the expression of C/VIF1 and C/VIF2 in response to ABA treatment, and qRT-PCR was used to quantitative the fold changes of the expression levels. The expression of ACT2 (ACTIN2) was used as a control. The primers used for PCR have been described previously.Citation36
Constructs
To generate the 35 S:C/VIF1 construct for plant transformation, the full-length ORF sequence of C/VIF1 was amplified by RT-PCR, double digested with Nde1 and Sac1 enzymes and cloned in frame with an N-terminal HA tag into the pUC19 vector. The pUC19-35S:C/VIF1 construct was then digested with Pst1 and Sac1 enzymes and cloned into the binary vector pPZP211,Citation39 to generate pPZP-35S:C/VIF1 construct. The primers used to amplify C/VIF1 were C/VIF1-Nde1F, 5ʹ-CAACATATGATGAAGATGATGAAGGTGATGATG-3ʹ, and C/VIF1-Sac1R, 5ʹ- CGAGCTCTTACCAACAAGTTCTTCCTCTATTGAA-3ʹ.
To generate CRISPR/Cas9 constructs for C/VIF1 gene editing, potential target sequences within the exons of C/VIF1 were selected by using CRISPRscan (http://www.crisprscan.org/?page=sequence). Target specificity was then evaluated on Cas-OFFinder (http://www.rgenome.net/cas-offinder/). The specific target sequences selected for editing C/VIF1 were 5ʹ-GGTTCCTCTGCCGACACTTC(TGG)-3ʹ, 5ʹ-GTCCCCAAATTCGGCGAAGA(CGG)-3ʹ and 5ʹ-GAAGACGGTGTGATCGACGC(CGG)-3ʹ. We also selected one target for C/VIF2, i.e., 5ʹ-AGTCCCACAGCCGACACAAA(AGG)-3ʹ. The target sequences were inserted into the pHEE-FT vector,Citation40 by using a method as described by Wang et al.Citation41 The primers used to generate pHEE-FT-C/VIF1 construct were DT1-BsF (C/VIF1sgR1), 5ʹ-ATATATGGTCTCGATTGGTTCCTCTGCCGACACTTCGTT-3ʹ, DT1-F0 (C/VIF1sgR1), 5ʹ-TGGTTCCTCTGCCGACACTTCGTTTTAGAGCTAGAAATAGC-3ʹ, DT2-R0 (C/VIF1sgR2), 5ʹ-AACGCGTCGATCACACCGTCTTCAATCTCTTAGTCGACTCTAC-3ʹ, and DT2-BsR (C/VIF1sgR2), 5ʹ-ATTATTGGTCTCGAAACGCGTCGATCACACCGTCTTC-3ʹ. The primers used to generate pHEE-FT-C/VIF1-C/VIF2 construct were DT1-BsF(C/VIF1sgR3), 5ʹ-ATATATGGTCTCGATTGTCCCCAAATTCGGCGAAGAGTT-3ʹ, DT1-F0 (C/VIF1sgR3), 5ʹ-TGTCCCCAAATTCGGCGAAGAGTTTTAGAGCTAGAAATAGC-3ʹ, DT2-R0 (C/VIF2sgR1), 5ʹ-AACTTTGTGTCGGCTGTGGGACCAATCTCTTAGTCGACTCTAC-3ʹ, and DT2-BsR (C/VIF2sgR1), 5ʹ-ATTATTGGTCTCGAAACTTTGTGTCGGCTGTGGGACC-3ʹ. The U626-IDF and U629-IDR primersCitation42 were used for colony PCR and sequencing of the CRISPR/Cas9 constructs generated.
Plant transformation, transgenic plant selection and transgene-free mutant isolation
The Col wild-type plants with several mature flowers (~5-week-old under our growth conditions) were transformed via GV3101 mediated transformation by using floral dip method.Citation43 The plants were transformed with the pPZP-35S:C/VIF1 construct to generate 35S:C/VIF1 overexpression plants, and the CRISPR/Cas9 constructs to generate c/vif1 mutants.
The 35S:C/VIF1 transgenic plants were selected by germinating the T1 seeds on 1/2 MS plates containing 50 μg/ml Kanamycin and 100 μg/ml Carbenicillin. The 35S:C/VIF1 transgenic plants with a single T-DNA insertion were selected by germinating T2 seeds, whereas homozygous 35S:C/VIF1 transgenic plants were selected by germinating T3 seeds on1/2 MS plates containing 30 μg/ml Kanamycin. Multiple homozygous lines were obtained and two with high expression levels of C/VIF1 were used for the experiments.
Transgenic plants generated with the CRISPR/Cas9 constructs were selected by germinating the T1 seeds on 1/2 MS plates containing 30 μg/ml Hygromycin and 100 μg/ml Carbenicillin, and transgene-free mutants were isolated as described previously.Citation40 In brief, early flowering T1 plants were selected and gene editing status was examined by amplifying and sequencing the genomic sequence of C/VIF1. T2 plants with normal flowering time were selected, and transgene-free status was confirmed by PCR amplification of Cas9 gene fragment.
DNA isolation and PCR
To examine C/VIF1 editing status, DNA was isolated from leaves of early flowering T1 or normal flowering T2 transgenic plants generated using the CRISPR/Cas9 constructs, genomic sequences of C/VIF1 were amplified by PCR and sequenced. To isolate transgene-free mutants, DNA was isolated from leaves of normal flowering T2 transgenic plants and used for PCR amplification of the Cas9 gene fragment. The primers for amplifying Cas9 fragment have been described previously.Citation42
In planta invertase activity analysis
Invertase activity was analyzed by NBT (nitroblue tetrazolium) staining as described previously.Citation36 Briefly, seeds of the Col wild type and the c/vif1 mutants were fixed with fixation buffer, rinsed with water to remove soluble sugar, and stained with staining solution. After staining, the seeds were rinsed with water and photographed under a dissection microscopy equipped with a digital camera.
ABA and salt sensitivity assays
ABA inhibited seed germination and cotyledon greening assays were performed as described previously.Citation44 Briefly, seeds of the Col wild type, the 35S:C/VIF1 transgenic plants and the c/vif1 mutants were sterilized and germinated on 1/2 MS plates in the presence or absence of 0.75 µM ABA. The plates were kept in darkness at 4°C for 2 d and then transferred to a growth room. Seeds germinated were counted every 12 h, photographs were taken and seedlings with green cotyledons were counted 8 d after the transfer. Percentage of germination and green seedlings was calculated.
For salt tolerance assays, seeds of the Col wild type, the 35S:C/VIF1 transgenic plants and the c/vif1 mutants were sterilized and germinated on 1/2 MS plates in the presence or absence of 200 mM NaCl. The plates were transferred to a growth room after kept for 2 d in darkness at 4°C. Seeds germinated were counted every 12 h, and the percentage of germination was calculated. All the experiments were repeated at least three times.
Results
Expression of C/VIF1 is upregulated by ABA treatment
ABA plays an important role in regulating plant response to abiotic stresses. In order to identify novel players in ABA signaling and/or abiotic stress tolerance, we attempted to characterize the ABA response genes identified in our previously transcriptome assays, and with unknown functions or functions in ABA signaling and/or abiotic stresses remained unknown.Citation45 By using this strategy, we have previously successfully identified bHLH129 transcription factor as an ABA response regulator,Citation6,Citation46 and AITRs as a novel family of transcription repressors that play a feed-forward regulating role in ABA signaling and function as negative regulators of plant response to abiotic stresses such as drought and salt.Citation6
In the previous transcriptome data set, we found that the expression level of the invertase inhibitor gene C/VIF1 was greatly increased in response to ABA treatment, with an RPKM of 22 in ABA treated seedlings compared with 2 in control samples. In another report, it has also been shown that the expression level of C/VIF1 was slightly increased in response to ABA treatment.Citation36
Therefore, we examined the expression of C/VIF1 in response to ABA in Arabidopsis seedlings. The Col wild-type seedlings were treated with 50 μM ABA and RT-PCR was used to examine the expression of C/VIF1. We found that the expression level of C/VIF1 was clearly increased in Arabidopsis seedlings treated with ABA (). Quantitative RT-PCR results showed that the expression level of C/VIF1 in Arabidopsis seedlings increased about 10 folds in response to ABA (). On the other hand, the expression level of another known invertase inhibitor gene, C/VIF2 remained largely unchanged ().
Figure 1. Expression of C/VIF1 in response to ABA treatment. (a) Expression of C/VIF1 was induced by ABA. Seven-day-old Col seedlings were treated with 50 µM ABA or mock-treated with solvent methanol for 4 h. Total RNA was isolated, cDNA was synthesized and used as a template for RT-PCR. The expression of ACT2 was used as a control. (b) Fold changes of C/VIF1 in response to ABA. Expression level of C/VIF1 was examined by qRT-PCR. The expression of ACT2 was used as an inner control. The fold change was calculated by comparing the expression levels of C/VIF1 in ABA treated and mock-treated seedlings. Data represent the mean ± SD of three replicates.
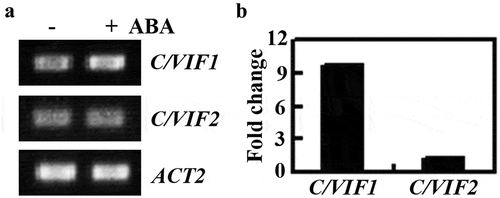
Functions of C/VIF1 is affected in the gene edited c/vif1 mutants
Having shown that expression of C/VIF1 was greatly upregulated by ABA treatment (), we wanted to explore the functions of C/VIF1 in ABA response and abiotic tolerance in Arabidopsis by generating and analyzing overexpression plants and loss-of-function mutants of the C/VIF1. Transgenic plants overexpressing C/VIF1 were generated by expressing C/VIF1 under the control of the 35S promoter (35S:C/VIF1) in the Col wild-type plants, and CRISPR/Cas9 gene editing was used to generate c/vif1 mutants.
Since we have used the FT expression cassette-containing CRISPR/Cas9 construct pHEE-FT to generated transgene-free Arabidopsis mutants in a much fast and easier way,Citation40 the pHEE-FT vector was used to generate CRISPR/Cas9 constructs for C/VIF1 gene editing. Two pHEE-FT vector constructs were generated. The pHEE-FT-C/VIF1 construct was made to target C/VIF1 gene at two different sites, aimed to generate fragment deletion mutants. The pHEE-FT-C/VIF1-C/VIF2 construct was made to target one target in C/VIF1gene, and the other in C/VIF2, intended to generate double mutants ().
Figure 2. Generation of pHEE-FT CRISPR/Cas9 constructs for C/VIF1 gene editing. (a) Diagram of the pHEE-FT vector with a GmFT2a expression cassette. (b) The sgRNA expression cassettes in the pHEE-FT-C/VIF1 and pHEE-FT-C/VIF1-C/VIF2 constructs. The target sequences of C/VIF1 and C/VIF2 were introduced into the sgRNA expression cassettes by PCR amplification, and the sgRNA expression cassettes were cloned into the pHEE-FT vector by using Golden gate cloning. (c) Target sequences in C/VIF1 and C/VIF2. Numbers indicate the positions of the nucleotides in the coding sequences of C/VIF1 or C/VIF2. PAM sites were indicated in the brackets.
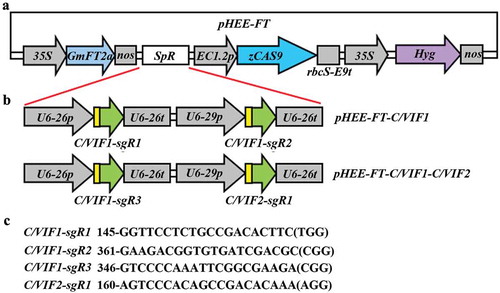
We obtained c/vif1 single mutants with both constructs. The mutant generated by using pHEE-FT-C/VIF1-C/VIF2 construct, c/vif1-c1, has a single nucleotide insertion occurred in C/VIF1 (), led to a few amino acid substitutions and premature stop in C/VIF1 (). The premature stop occurred after PKF (), a motif has been shown to be critical for invertase–inhibitor interaction.Citation32 We also obtained another c/vif1 mutant by using the pHEE-FT-C/VIF1 construct, c/vif1-c2, which has 216 nucleotides fragment deletion in the coding sequence of C/VIF1 (), resulted in 73 amino acids deletion spanning the PKF domain in C/VIF1 (). NBT (nitroblue tetrazolium) staining of the seeds showed that the invertase activities were increased in both c/vif1-c1 and c/vif1-c2 mutants (), suggest that the function of C/VIF1 was affected in these c/vif1 mutants.
Figure 3. Isolation of transgene-free c/vif1 mutants. (a) Editing status of C/VIF1 in the c/vif1 mutants. The c/vif1-c1 and c/vif1-c2 mutants were obtained by transforming Col wild-type plants with pHEE-FT-C/VIF1-C/VIF2 and pHEE-FT-C/VIF1 constructs, respectively. Editing status in T1 generation was examined, and transgene-free homozygous mutants were isolated in T2 generation. Star indicates the single T nucleotide insertion in the c/vif1-c1 mutant. Arrowhead indicates the 216 bp fragment deletion in the c/vif1-c2 mutant. Underlines indicate the PAM sites. (b) Alignment of C/VIF1 amino acid sequences in the Col wild type and the c/vif1 mutants. ORF of C/VIF1 in the c/vif1 mutants was identified by using ORFfinder (https://www.ncbi.nlm.nih.gov/orffinder/) and predicted amino acid sequences were used for alignment with the amino acid sequence of C/VIF1. Underline indicates the PKF motif. (c) NBT staining of the seeds. Seeds of the Col wild type and the c/vif1 mutants were fixed with fixation buffer for 1 h at 4°C, rinsed overnight with water to remove soluble sugar, and stained with staining buffer in a water bath at 30°C. Stained seeds were rinsed with water and photographed under a dissection microscopy equipped with a digital camera.
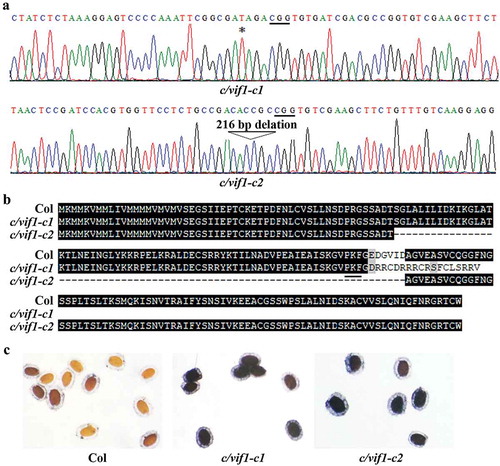
The 35S:CVIF1 transgenic plants are hypersensitive to ABA
After generated the C/VIF1 overexpression and the c/vif1 mutant plants, we examined their ABA response by using seed germination and cotyledon greening assays.
As shown in , reduced germination rate was observed for the 35S:C/VIF1 transgenic plant seeds at nearly all the time points examined, indicating that the 35S:C/VIF1 transgenic plants are more sensitive to ABA. On the other hand, a little difference on germination rate between the seeds of Col wild type and the c/vif1-c2 mutant plants was observed at the beginning, however, reduced germination rate was observed for the c/vif1 mutant plant seeds at the other time points examined (). The results suggest that disruption of the functions of C/VIF1 affected plant response to ABA.
Figure 4. Effects of ABA on seed germination and cotyledon greening of the Col wild type, the 35S:C/VIF1 transgenic plants and the c/vif1 mutants. (a) Effects of ABA on seed germination of the Col wild type, the 35S:C/VIF1 transgenic plants and the c/vif1 mutants. Sterilized seeds of the Col wild type, the 35S:C/VIF1 transgenic plants and the c/vif1 mutants were germinated on 1/2 MS plates in the presence or absence of 0.75 µM ABA. The plates were transferred to a growth room after kept in darkness for 2 d at 4°C. Seeds germinated were counted every 12 h and the percentage of germination was then calculated. All the seeds on the control plates were generated 24 h after the transfer. Data represent the mean ± SD of three replicates. (b) Effects of ABA on cotyledon greening of the Col wild type, the 35S:C/VIF1 transgenic plants and the c/vif1 mutants. Sterilized seeds of the Col wild type, the 35S:C/VIF1 transgenic plants and the c/vif1 mutants were germinated on 1/2 MS plates in the presence or absence of 0.75 µM ABA. The plates were transferred to a growth room after kept in darkness for 2 d at 4°C, and pictures were taken 8 d after the transfer. (c) Percentage of green seedlings of the Col wild type, the 35S:C/VIF1 transgenic plants and the c/vif1 mutants in response to ABA treatment. Seedlings with green cotyledons were counted 8 d after the transfer and used to calculate the percentage of green cotyledons. Data represent the mean ± SD of three replicates.
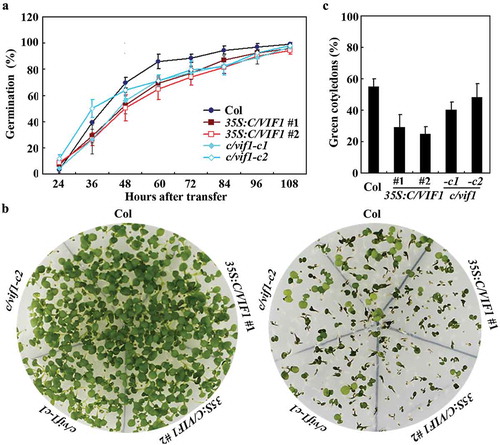
In the cotyledon greening assays, the 35S:C/VIF1 plants also showed enhanced sensitivity to ABA treatment, but a little, if any difference was observed for the c/vif1 mutants when compared with the Col wild-type plants (). Quantitative analysis showed that the green cotyledon rate of the 35S:C/VIF1 plants was only about half of the Col wild-type plants, whereas a slight decreased green cotyledon rate was observed for the c/vif1 mutants ().
Salt tolerance is decreased in the 35S:C/VIF1 transgenic plants
ABA is a key hormone that affects plant response to abiotic stresses, having shown that C/VIF1 regulates ABA response in Arabidopsis, we further examined if C/VIF1 may also regulate plant response to abiotic stresses. We found that reduced germination rate was observed for the 35S:C/VIF1 transgenic plant seeds at nearly all the time points examined, suggest that salt tolerance is reduced in the 35S:C/VIF1 transgenic plants in the seed germination assays, whereas germination rate was slightly increased in the c/vif1-c2 mutant seeds, but the c/vif1-c1 mutants showed a near wild-type response to salt treatments ().
Figure 5. Effects of NaCl on seed germination of the Col wild type, the 35S:C/VIF1 transgenic plants and the c/vif1 mutants. Sterilized seeds of the Col wild type, the 35S:C/VIF1 transgenic plants and the c/vif1 mutants were germinated on 1/2 MS plates in the presence or absence of 200 mM NaCl. The plates were transferred to a growth room after kept in darkness for 2 d at 4°C. Seeds germinated were counted every 12 h and the percentage of germination was then calculated. All the seeds on the control plates generated 24 h after the transfer. Data represent the mean ± SD of three replicates.
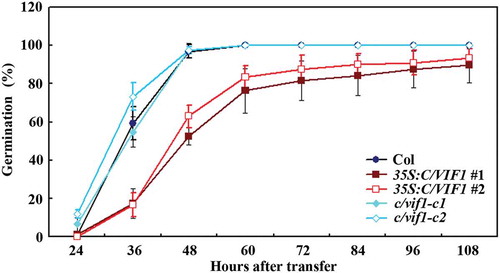
Discussion
C/VIFs are low-molecular-weight proteinaceous inhibitors that can inhibit the activities of CWI and VI,Citation31 both are acid invertases that can irreversibly cleave sucrose, into glucose and fructose.Citation20-Citation23 Consistent with their roles in sucrose metabolism, accumulated evidence suggests that changes in C/VIF gene expression affect plant growth and development, as well as plants response to stresses including biotic stresses and abiotic stresses in several different plants such as potato,Citation47-Citation50 tomato,Citation34,Citation35,Citation51 poplar,Citation33 and Arabidopsis.Citation36,Citation37 Evidences provided in this study suggest that C/VIF1 plays a role in the regulation of ABA response and salt tolerance in Arabidopsis. First, the expression of C/VIF1 was increased in response to ABA treatment (). Second, the 35S:C/VIF1 transgenic plants showed enhanced sensitivity to ABA in both seed germination and seedling greening assays (). Third, the 35S:C/VIF1 transgenic plants showed a reduced salt tolerance in seed germination assay ().
In addition to serve as metabolites and nutrient sources of the plants, glucose and fructose also serve as signaling molecules to modulate gene expression during plant growth and development, and plant response to environmental stimuli,Citation24,Citation25 whereas CWI and VI are able to cleave sucrose into glucose and fructose.Citation20-Citation23 Therefore it is reasonable to speculate that C/VIF1’s functions in regulating salt response is, at least partially, due to its ability in inhibiting CWI and VI activities, therefore affected the cleaving of sucrose into glucose and fructose. On the other hand, considering that ABA sensitivity in the 35S:C/VIF1 transgenic plants was affected (), it will be of great interest to examine if and how ABA signaling may also play a role in C/VIF1 mediated salt tolerance in Arabidopsis. Considering that salinity affects ion content in plants, it will be also of interest to examine if there is any difference in ion contents in the Col wild type, the 35S:C/VIF1 transgenic plants and the c/vif1 mutants in response to salt treatment.
We noted that slightly reduced sensitivity to ABA was observed only in the c/vif1-c2, but not the c/vif1-c1 mutants (), similar, slightly enhanced salt tolerance was also observed only in the c/vif1-c2 mutants (). It has been reported that CIF interacts with CWI via its PKF motif, suggesting that C/VIFs inhibit invertase activities via direct physical interactions.Citation32,Citation52 Considering that the single nucleotide insertion in the c/vif1-c1 mutant resulted in a few amino acid substitutions and premature stop for C/VIF1 after the PKF motif, whereas the 216 nucleotides fragment deletion in the c/vif1-c2 mutant resulted in 73 amino acids deletion of the PKF domain in C/VIF1 (), it is very likely that the c/vif1-c2 is a loss-of-function mutant, but the c/vif1-c1 is not. However, because the invertase activities were also increased in the c/vif1-c1 mutant (), it is possible that C/VIF1 in the c/vif1-c1 mutant may still able to interact with invertases, but its activities in inhibiting invertases were reduced. Even though additional evidence may be required to support that tolerance to ABA and salt was increased in loss-of-function mutants of C/VIF1, our evidence with the 35S:C/VIF1 transgenic plants indeed indicate that C/VIF1 plays a negative role in ABA response and salt tolerance.
It should be noted that C/VIF may have opposite effects in regulating abiotic stress responses in different plants. For example, silencing of CIF enhanced cold tolerance in tomato,Citation35 but ectopic expression of VIF enhanced drought tolerance in Arabidopsis.Citation37 However, silencing of C/VIF can improve agronomic traits in all plants studied so far. For example, silencing of CIF improved seed filling in tomato,Citation34 loss-of-function of CIF1 increased seed production in Arabidopsis,Citation36 and suppression of C/VIF genes by RNAi improved seed weight in soybean.Citation53 Considering that CRISPR/Cas9 gene editing is able to generate transgene-free mutants and has been used to improve important agronomic traits in crops,Citation54-Citation56 editing of C/VIF genes together with other agronomic trait controlling genes by CRISPR/Cas9 may enable to integrate desired agronomic traits in plants.
Disclosure of potential conflicts of interest
No potential conflicts of interest were disclosed.
Author contributions
SW conceived the study. SW, WY designed the experiments. WY, SC, YC, NZ, YM, WW, HT, YL and SH performed the experiments. WY and SW analyzed the data. SW drafted the manuscript. All the authors participated in the revision of the manuscript.
Additional information
Funding
References
- Wang W, Vinocur B, Altman A. Plant responses to drought, salinity and extreme temperatures: towards genetic engineering for stress tolerance. Planta. 2003;218:1–9. doi:10.1007/s00425-003-1105-5.
- Fujita M, Fujita Y, Noutoshi Y, Takahashi F, Narusaka Y, Yamaguchi-Shinozaki K, Shinozaki K. Crosstalk between abiotic and biotic stress responses: a current view from the points of convergence in the stress signaling networks. Curr Opin Plant Bio. 2006;9:436–442. doi:10.1016/j.pbi.2006.05.014.
- Umezawa T, Nakashima K, Miyakawa T, Kuromori T, Tanokura M, Shinozaki K, Yamaguchi-Shinozaki K. Molecular basis of the core regulatory network in ABA responses: sensing, signaling and transport. Plant Cell Physiol. 2010;51:1821–1839. doi:10.1093/pcp/pcq156.
- Yoshida T, Mogami J, Yamaguchi-Shinozaki K. ABA-dependent and ABA-independent signaling in response to osmotic stress in plants. Curr Opin Plant Biol. 2014;21:133–139. doi:10.1016/j.pbi.2014.07.009.
- Song L, Huang SC, Wise A, Castanon R, Nery JR, Chen H, Watanabe M, Thomas J, Bar-Joseph Z, Ecker JR. A transcription factor hierarchy defines an environmental stress response network. Science. 2016;354:aag1550. doi:10.1126/science.aag1550.
- Tian H, Chen S, Yang W, Wang T, Zheng K, Wang Y, Cheng Y, Zhang N, Liu S, Li D, et al. A novel family of transcription factors conserved in angiosperms is required for ABA signalling. Plant Cell Environ. 2017;40:2958–2971.
- Ma Y, Szostkiewicz I, Korte A, Moes D, Yang Y, Christmann A, Grill E. Regulators of PP2C phosphatase activity function as abscisic acid sensors. Science. 2009;324:1064–1068. doi:10.1126/science.1172408.
- Park SY, Fung P, Nishimura N, Jensen DR, Fujii H, Zhao Y, Lumba S, Santiago J, Rodrigues A, Chow TF, et al. Abscisic acid inhibits type 2C protein phosphatases via the PYR/PYL family of START proteins. Science. 2009;324:1068–1071. doi:10.1126/science.1173041.
- Santiago J, Rodrigues A, Saez A, Rubio S, Antoni R, Dupeux F, Park SY, Marquez JA, Cutler SR, Rodriguez PL. Modulation of drought resistance by the abscisic acid receptor PYL5 through inhibition of clade A PP2Cs. Plant J. 2009;60:575–588. doi:10.1111/j.1365-313X.2009.03981.x.
- Rodriguez PL, Leube MP, Grill E. Molecular cloning in Arabidopsis thaliana of a new protein phosphatase 2C (PP2C) with homology to ABI1 and ABI2. Plant Mol Biol. 1998;38:879–883. doi:10.1023/A:1006012218704.
- Gosti F, Beaudoin N, Serizet C, Webb AA, Vartanian N, Giraudat J. ABI1 protein phosphatase 2C is a negative regulator of abscisic acid signaling. Plant Cell. 1999;11:1897–1910. doi:10.1105/tpc.11.10.1897.
- Fujii H, Verslues PE, Zhu JK. Identification of two protein kinases required for abscisic acid regulation of seed germination, root growth, and gene expression in Arabidopsis. Plant Cell. 2007;19:485–494. doi:10.1105/tpc.106.048538.
- Stone SL, Williams LA, Farmer LM, Vierstra RD, Callis J. KEEP ON GOING, a RING E3 ligase essential for Arabidopsis growth and development, is involved in abscisic acid signaling. Plant Cell. 2006;18:3415–3428. doi:10.1105/tpc.106.046532.
- Liu H, Stone SL. Abscisic acid increases Arabidopsis ABI5 transcription factor levels by promoting KEG E3 ligase self-ubiquitination and proteasomal degradation. Plant Cell. 2010;22:2630–2641. doi:10.1105/tpc.110.076075.
- Chen YT, Liu HX, Stone S, Callis J. ABA and the ubiquitin E3 ligase KEEP ON GOING affect proteolysis of the Arabidopsis thaliana transcription factors ABF1 and ABF3. Plant J. 2013;75:965–976. doi:10.1111/tpj.12259.
- Park SY, Peterson FC, Mosquna A, Yao J, Volkman BF, Cutler SR. Agrochemical control of plant water use using engineered abscisic acid receptors. Nature. 2015;520:545–548. doi:10.1038/nature14123.
- Zhao Y, Chan Z, Gao J, Xing L, Cao M, Yu C, Hu Y, You J, Shi H, Zhu Y, et al. ABA receptor PYL9 promotes drought resistance and leaf senescence. Proc Natl Acad Sci USA. 2016;113:1949–1954. doi:10.1073/pnas.1522840113.
- Fujita Y, Nakashima K, Yoshida T, Katagiri T, Kidokoro S, Kanamori N, Umezawa T, Fujita M, Maruyama K, Ishiyama K, et al. Three SnRK2 protein kinases are the main positive regulators of abscisic acid signaling in response to water stress in Arabidopsis. Plant Cell Physiol. 2009;50:2123–2132. doi:10.1093/pcp/pcp147.
- Yoshida T, Fujita Y, Maruyama K, Mogami J, Todaka D, Shinozaki K, Yamaguchi-Shinozaki K. Four Arabidopsis AREB/ABF transcription factors function predominantly in gene expression downstream of SnRK2 kinases in abscisic acid signaling in response to osmotic stress. Plant Cell Environ. 2015;38:35–49. doi:10.1111/pce.12351.
- Koch K. Sucrose metabolism: regulatory mechanisms and pivotal roles in sugar sensing and plant development. Curr Opin Plant Biol. 2004;7:235–246. doi:10.1016/j.pbi.2004.03.014.
- Sturm A. Invertases. Primary structures, functions, and roles in plant development and sucrose partitioning. Plant Physiol. 2002;121:1–8. doi:10.1104/pp.121.1.1.
- Van den Ende W, Lammens W, Van Laere A, Schroeven L, Le Roy K. Donor and acceptor substrate selectivity among plant glycoside hydrolase family 32 enzymes. FEBS J. 2009;276:5788–5798. doi:10.1111/j.1742-4658.2009.07316.x.
- Wan H, Wu L, Yang Y, Zhou G, Ruan YL. Evolution of sucrose metabolism: the dichotomy of invertases and beyond. Trends Plant Sci. 2018;23:163–177. doi:10.1016/j.tplants.2017.11.001.
- Rolland F, Baena-Gonzalez E, Sheen J. Sugar sensing and signaling in plants: conserved and novel mechanisms. Annu Rev Plant Biol. 2006;57:675–709. doi:10.1146/annurev.arplant.57.032905.105441.
- Ruan Y-L. Sucrose metabolism: gateway to diverse carbon use and sugar signaling. Annu Rev Plant Biol. 2014;65:33–67. doi:10.1146/annurev-arplant-050213-040251.
- Balibrea Lara ME, Gonzalez Garcia M-C, Fatima T, Ehneß R, Lee TK, Proels R, Tanner W, Roitsch T. Extracellular invertase is an essential component of cytokinin-mediated delay of senescence. Plant Cell. 2004;16:1276–1287. doi:10.1105/tpc.018929.
- Albacete A, Cantero-Navarro E, Großkinsky DK, Arias CL, Balibrea ME, Bru R, Fragner L, Ghanem ME, González Mde L, Hernández JA, et al. Ectopic overexpression of the cell wall invertase gene CIN1 leads to dehydration avoidance in tomato. J Exp Bot. 2015;66:863–878. doi:10.1093/jxb/eru448.
- Bhaskar PB, Wu L, Busse JS, Whitty BR, Hamernik AJ, Jansky SH, Buell CR, Bethke PC, Jiang J. Suppression of the vacuolar invertase gene prevents cold-induced sweetening in potato. Plant Physiol. 2010;154:939–948. doi:10.1104/pp.110.162545.
- Zhu X, Gong H, He Q, Zeng Z, Busse JS, Jin W, Bethke PC, Jiang J. Silencing of vacuolar invertase and asparagine synthetase genes and its impact on acrylamide formation of fried potato products. Plant Biotechnol J. 2016;14:709–718. doi:10.1111/pbi.12421.
- Qian W, Xiao B, Wang L, Hao X, Yue C, Cao H, Wang Y, Li N, Yu Y, Zeng J, et al. CsINV5, a tea vacuolar invertase gene enhances cold tolerance in transgenic Arabidopsis. BMC Plant Biol. 2018;18:228. doi:10.1186/s12870-018-1456-5.
- Rausch T, Greiner S. Plant protein inhibitors of invertases. Biochim Biophys Acta-Proteins Proteomics. 2004;1696:253–261. doi:10.1016/j.bbapap.2003.09.017.
- Hothorn M, Van den Ende W, Lammens W, Rybin V, Scheffzek K. Structural insights into the pH-controlled targeting of plant cell-wall invertase by a specific inhibitor protein. Proc Natl Acad Sci. 2010;107:17427–17432. doi:10.1073/pnas.1004481107.
- Su T, Han M, Min J, Zhou H, Zhang Q, Zhao J, Fang Y. Functional characterization of invertase inhibitors PtC/VIF1 and 2 revealed their involvements in the defense response to fungal pathogen in. Populus Trichocarpa Front Plant Sci. 2020;10:1654. doi:10.3389/fpls.2019.01654.
- Jin Y, Ni D-A, Ruan Y-L. Posttranslational elevation of cell wall invertase activity by silencing its inhibitor in tomato delays leaf senescence and increases seed weight and fruit hexose level. Plant Cell. 2009;21:2072–2089. doi:10.1105/tpc.108.063719.
- Xu X, Hu Q, Yang W, Jin Y. The roles of cell wall invertase inhibitor in regulating chilling tolerance in tomato. BMC Plant Biol. 2017;17:195. doi:10.1186/s12870-017-1145-9.
- Su T, Wolf S, Han M, Zhao H, Wei H, Greiner S, Rausch T. Reassessment of an Arabidopsis cell wall invertase inhibitor AtCIF1 reveals its role in seed germination and early seedling growth. Plant Mol Biol. 2016;90:137–155. doi:10.1007/s11103-015-0402-2.
- Chen SF, Liang K, Yin DM, Ni DA, Zhang ZG, Ruan YL. Ectopic expression of a tobacco vacuolar invertase inhibitor in guard cells confers drought tolerance in Arabidopsis. J Enzyme Inhib Med Chem. 2016;31:1381–1385. doi:10.3109/14756366.2016.1142981.
- Wang X, Wang X, Hu Q, Dai X, Tian H, Zheng K, Wang X, Mao T, Chen JG, Wang S. Characterization of an activation-tagged mutant uncovers a role of GLABRA2 in anthocyanin biosynthesis in Arabidopsis. Plant J. 2015;83:300–311. doi:10.1111/tpj.12887.
- Hajdukiewicz P, Svab Z, Maliga P. The small, versatile pPZP family of Agrobacterium binary vectors for plant transformation. Plant Mol Biol. 1994;25:989–994. doi:10.1007/BF00014672.
- Cheng Y, Zhang N, Hussain S, Ahmed S, Yang W, Wang S. Integration of a FT expression cassette into CRISPR/Cas9 construct enables fast generation and easy identification of transgene-free mutants in Arabidopsis. PLoS One. 2019;14:e0218583. doi:10.1371/journal.pone.0218583.
- Wang ZP, Xing HL, Dong L, Zhang HY, Han CY, Wang XC, Chen QJ. Egg cell-specific promoter-controlled CRISPR/Cas9 efficiently generates homozygous mutants for multiple target genes in Arabidopsis in a single generation. Genome Biol. 2015;16:144. doi:10.1186/s13059-015-0715-0.
- Chen S, Zhang N, Zhang Q, Zhou G, Tian H, Hussain S, Ahmed S, Wang T, Wang S. Genome editing to integrate seed size and abiotic stress tolerance traits in Arabidopsis reveals a role for DPA4 and SOD7 in the regulation of inflorescence architecture. Int J Mol Sci. 2019;20:2695. doi:10.3390/ijms20112695.
- Clough SJ, Bent AF. Floral dip: a simplified method for Agrobacterium-mediated transformation of Arabidopsis thaliana. Plant J. 1998;16:735–743. doi:10.1046/j.1365-313x.1998.00343.x.
- Guo J, Wang J, Xi L, Huang WD, Liang J, Chen JG. RACK1 is a negative regulator of ABA responses in Arabidopsis. J Exp Bot. 2009;60:3819–3833. doi:10.1093/jxb/erp221.
- Guo J, Wang S, Valerius O, Hall H, Zeng Q, Li JF, Weston DJ, Ellis BE, Chen JG. Involvement of Arabidopsis RACK1 in protein translation and its regulation by abscisic acid. Plant Physiol. 2011;155:370–383. doi:10.1104/pp.110.160663.
- Tian H, Guo H, Dai X, Cheng Y, Zheng K, Wang X, Wang S. An ABA down-regulated bHLH transcription repressor gene, bHLH129 regulates root elongation and ABA response when overexpressed in Arabidopsis. Sci Rep. 2015;5:17587. doi:10.1038/srep17587.
- Greiner S, Rausch T, Sonnewald U, Herbers K. Ectopic expression of a tobacco invertase inhibitor homolog prevents cold-induced sweetening of potato tubers. Nat Biotechnol. 1999;17:708–711. doi:10.1038/10924.
- Brummell DA, Chen RKY, Harris JC, Zhang H, Hamiaux C, Kralicek AV, McKenzie MJ. Induction of vacuolar invertase inhibitor mRNA in potato tubers contributes to cold-induced sweetening resistance and includes spliced hybrid mRNA variants. J Exp Bot. 2011;62:3519–3534. doi:10.1093/jxb/err043.
- Liu X, Lin Y, Liu J, Song B, Ou Y, Zhang H, Li M, Xie C. StInvInh2 as an inhibitor of StvacINV1 regulates the cold-induced sweetening of potato tubers by specifically capping vacuolar invertase activity. Plant Biotechnol J. 2013;11:640–647. doi:10.1111/pbi.12054.
- Mckenzie MJ, Chen RKY, Harris JC, Ashworth MJ, Brummell DA. Post-translational regulation of acid invertase activity by vacuolar invertase inhibitor affects resistance to cold-induced sweetening of potato tubers. Plant Cell Environ. 2013;36:176–185. doi:10.1111/j.1365-3040.2012.02565.x.
- Qin G, Zhu Z, Wang W, Cai J, Chen Y, Li L, Tian S. A tomato vacuolar invertase inhibitor mediates sucrose metabolism and influences fruit ripening. Plant Physiol. 2016;172:1596–1611. doi:10.1104/pp.16.01269.
- Hothorn M, Wolf S, Aloy P, Greiner S, Scheffzek K. Structural insights into the target specificity of plant invertase and pectin methylesterase inhibitory proteins. Plant Cell. 2004;16:3437–3447. doi:10.1105/tpc.104.025684.
- Tang X, Su T, Han M, Wei L, Wang W, Yu Z, Xue Y, Wei H, Du Y, Greiner S, et al. Suppression of extracellular invertase inhibitor gene expression improves seed weight in soybean (Glycine max). J Exp Bot. 2017;68:469–482. doi:10.1093/jxb/erw425.
- Ma X, Zhang Q, Zhu Q, Liu W, Chen Y, Qiu R, Wang B, Yang Z, Li H, Lin Y, et al. A robust CRISPR/Cas9 system for convenient, high-efficiency multiplex genome editing in monocot and dicot plants. Mol Plant. 2015;8:1274–1284. doi:10.1016/j.molp.2015.04.007.
- Li X, Xie Y, Zhu Q, Liu YG. Targeted genome editing in genes and cis-regulatory regions improves qualitative and quantitative traits in crops. Mol Plant. 2017;10:1368–1370. doi:10.1016/j.molp.2017.10.009.
- He Y, Zhu M, Wang L, Wu J, Wang Q, Wang R, Zhao Y. Programmed self-elimination of the CRISPR/Cas9 construct greatly accelerates the isolation of edited and transgene-free rice plants. Mol Plant. 2018;11:1210–1213. doi:10.1016/j.molp.2018.05.005.