ABSTRACT
Plants evolve diverse strategies to cope with herbivorous insects, in which the lipid-derived phytohormone jasmonic acid (JA) plays a crucial role. This study was conducted to investigate the differential responses of tomato and maize plants to Spodoptera exiguaherbivory and to clarify the role played by JA, methyl-jasmonate (MeJA) and jasmonoyl-L-isoleucine (JA-L-Ile) in their defense responses. JA, MeJA and JA-L-Ile were quantified using HPLC-MS/MS. The results showed that maize plant was more tolerant toS. exiguaherbivory than tomato. Spodopteraexigua attack induced JA, MeJA, and JA-L-Ile to high levels after 2 h of infestation in both test plants. Then, all studied JAsconcentration decreased gradually by increasing infestation time up to 1 week. JA concentration in infested maize was much higher than that in infested tomato leaves. However, MeJA concentration in infested tomato leaves was higher than that in maize. In control plants, JA was not recorded, while MeJA was recorded in comparable values both in tomato and maize. Our results showed that JA plays the main role in increasing defense responses to S. exigua infestation in the studied plants as a direct signaling molecule; however, MeJA could play an indirect role by inducing JA accumulation. JA-L-Ile indicated a less efficient role in defense responses to S. exigua attack in both test plants where its level is much lower than JA and MeJA.
Introduction
Jasmonates (JAs) are significant signaling molecules including JA and its derivatives. They are mainly active in plant resistance to various stress conditions as well as plant development. Among developmental processes, JAs modulate tuber formation, tendril coiling, leaf senescence, trichome development, flower and seed development.Citation1−Citation3 Insects and pathogens attack, wounding, ozone, and UV light are the most common stress conditions that can be controlled by JAs.Citation1Citation4-Citation6 In plants attacked by insect herbivores, many defense genes are upregulated in undamaged tissues within hours. Several studies have revealed that herbivore wounding results in rapid accumulation of JAs at the site of feeding damage and results in steady increase of JAs content all over the plant.Citation1,Citation7,Citation8 The JAs are thought to function as long-distance signaling molecules and be transported in the phloem to undamaged tissues.Citation9,Citation10
Jasmonates biosynthesis occurs via the octadecanoid pathway. In response to wounding, polyunsaturated fatty acids (PUFAs) are esterified by lipases and liberated from the cell and chloroplast membranes.Citation11,Citation12 Inside the chloroplast, the PUFAs linolenic acid (18:3) and hexadecatrienoic acid (16:3) are converted into 12-oxophytodienoic acid (OPDA) and dinor-OPDA by the successive activities of lipoxygenase, allene oxide synthase, and allene oxide cyclase. OPDA and dnOPDA are then transported into the peroxisomes and reduced by OPDA reductase 3 (OPR3) to the respective cyclopentanones, yielding 3-oxo-2(2ʹ-pentenyl)-cyclopentane-1-octanoic acid (OPC-8:0) from OPDA. Then, JA produced after three cycles of β-oxidation to shorten the OPC-8:0 side chain.Citation13 Subsequent conjugation to a number of different compounds (amino acids, sugars, methanol, sulfate) results in the formation of a variety of JAs with different signaling activities.Citation3
Strong evidence show that some JA metabolites have unique signaling properties, and that some processes are not controlled by JA, but rather by JA derivatives.Citation14 For instance, methyl-JA (MeJA) and jasmonoyl-L-isoleucine (JA-L-Ile) are potent elicitors of defense gene expression.Citation3 MeJA exhibits mostly the same signaling functions inside the plant when compared to JA. However, because of its volatility, it may also serves as a mobile signal between plants that helps in plant–plant communication.Citation15,Citation16 In addition,Citation17 Staswick and Tiryaki showed that JA-L-Ile plays a critical role in numerous jasmonate-signaled processes. The experiments of metabolic labeling done byCitation18 wang et al. showed that JA-L-Ile synthesis is necessary for the induced systemic defense responses in Nicotiana attenuate. One of the substantial problems facing agriculture worldwide is the phytophagous insects that cause considerable yield damage across all major cultivated areas. The beet armyworm, Spodoptera exigua (Hübner), is an extremely serious and destructive agricultural pest in Saudi ArabiaCitation19Citation19Citation19 and in tropical and subtropical areas of the world.Citation20 It is a polyphagous chewing insect that can defoliate numerous economically important cultivated crops.Citation20
Although it is well known that JA has a crucial role in enhancing defense responses, there is uncertainty regarding the importance of other JAs derivatives in the degree of plant defense they can induce and the specificity of their response.Citation21 Bosch et al. showed that defense responses of JAs to insect attack in the same plant are differing according to insect species. Furthermore,Citation22 Ayoub and Afroz concluded that not all plants stimulate the same kind of chemical defense responses against a single herbivore. Therefore, it would be interesting to study more variety of plants and insect herbivory to investigate the chemical induction and responses. The aim of this study is to investigate the differential responses of tomato and maize plants to Spodoptera exiguainfestation and the contributions of JA, MeJA, and JA-L-Ilein their defense responses.
Materials and methods
Plant growth and insect rearing
The present study was carried out at King Abdulaziz University Experimental Station, Saudi Arabia during spring seasons (March–June) of 2016, which tend to have high temperatures and high levels of humidity. The average daily maximum temperature during the experiment was 32.13ºC. The daily minimum temperature was 22.08ºC. No rain falls during the experimental period.
The seeds of tomato (Solanum lycopersicum, cv Super Strain B) and maize (Zea mays, cv Golden bantam) were brought from USA Seeds Company (Modesto seed Co. Inc.) and germinated in soil. The seeds sown in 30 cm diameter plastic pots filled with soil mixed of sand and potting soil (2:1). Plants were grown outdoors under natural conditions in a shade-free location. Pots were arranged in randomized complete design with three replicates. Each replicate consists of 10 plants per pot. The seeds were watered with tap water using100% field capacity until the fourth leaves appeared, and then the plants used for insect infestation. The Spodoptera exigua larvae were used in all experiments after they had been reared on lettuce for more than one generation. Early third instars larvae selected for all insect experiments.
Insect infestation
Immediately prior to the start of insect infestation, plants were placed into an outdoor chamber coated with a fine-mesh net to protect them from other insects. Seven-d-old S. exigua larvae all about the same size were distributed on the plant leaves. Spodoptera exigua larvae were starved for 35 min before the experiments. Plant samples were collected after 2 h, 2 d and 1 week after infection. Control groups for each time did not receive any larvae. For jasmonate analysis, plant leaves were collected after certain time periods and frozen immediately in liquid nitrogen. All assays were done in triplicate.
Determination of photosynthetic pigments
Chlorophyll a and b pigments were extracted from 0.05 g fresh leaf samples. The leaf tissues were suspended in 5 ml of 95% ethyl alcohol at 60°C, until being colorless. Then, the total volume was completed to 10 ml with 95% ethyl alcohol. The absorbance readings were determined spectrophotometry (Lamda 25 UV/Vis spectrophotometer) at wavelengths of 663 and 644 nm. Chlorophyll concentrations were calculated using the equations cited by.Citation23
Determination of the soluble primary metabolites
Soluble solutes in plant leaves were extracted using the method described by El-Sharkawi and Michel.Citation24 Oven-dried leaves were ground into fine powder. Then, it was suspended in 5 ml distilled water and boiled in a water bath for 2 h, then centrifuged and completed to a certain volume.
Soluble carbohydrate concentration in leaf extracts was determined according to the method described by FalesCitation25 and SctilegelCitation26 using anthrone sulfuric acid reagent. Lowry et al.Citation27 method was followed to quantify the soluble protein content using Folin reagent. Moore and SteinCitation28 method used to determine free amino acid concentration in the same water extract of proteins and carbohydrates.
Jasmonates analysis using HPLC-MS-MS
Jasmonic acid, MeJA, and JA-L-Ile were extracted and determined using a modified method of Wang et al.Citation18 About 0.2 g of leaf tissues were homogenized in liquid nitrogen and extracted with 1 mL of ethyl acetate spiked with JA, MeJA, and JA-L-Ile as internal standards. After centrifugation, the clear extracts were transferred to new tubes and evaporated under vacuum. The residue was dissolved in 0.5 mL of 70% methanol and centrifuged for 5 min at maximum speed. The extract used for JA, MeJA, and JA-L-Ile analysis using LC-MS/MS system.
Statistical analysis
The results statistically analyzed using the SPS package software, version 21.0 (SPSS, Chicago, USA). Differences between infested and control (uninfested) plants at the same time point were tested with t-test. Significant increase (*) expressed at p < .05, highly significant increase (**) expressed at p < .01, highly highly significant increase (***) expressed at p < .001. Differences between different time periods for infested and control plants separately were tested with one-way analysis of variance (ANOVA), followed by (Duncan) test at a significance level of 5% (P < .05). All values were expressed as mean with their standard deviation (SD).
Results
Effect of S. exigua infestation on Chlorophyll a/b ratio
No significant differences were observed in Chl a/b ratio for S. exigua infestation (t-test: P = .065, 0.615, 0.365, ) in tomato leaves compared to uninfested plants at each observed time (). However, Chl a/b ratio of infested tomato plants at 2 d significantly increased higher than that at 2 h and 1 week of infestation (ANOVA: F = 7.81; P = .021, ). In maize, S. exigua infestation significantly reduced chl a/b ratio at 2 h compared to uninfested plants (t-test: P = .02, 0.13, 0.55, Figure 1II). However, Chl a/b of infested maize plants at 2 d and 1 week time periods significantly increased higher than that at 2 h (ANOVA: F = 6.52; P = .03, ).
Figure 1. Chlorophyll a/b ratio of tomato (i) and maize (II) as affected by Spodoptera exigua larvae infestation after different periods of time. Each point is a mean of three replicates ± standard error. The different letters represent the statistical significance between different time periods of uninfested (a–c) and infested (a–c) plants at P ≤ 0.05. Asterisks show significant differences between uninfested and infested plants at each time period (*p < .05; **p < .01; ***p < .001).
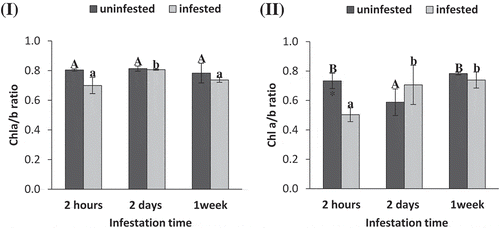
Effect of S. exigua infestation on the level of soluble carbohydrates
The statistical analysis showed no significant differences in soluble carbohydrate content in infested tomato plants compared to uninfested controls at each observed time (t-test: P = .46, 0.06, 0.33, ). However, in maize S. exigua infestation significantly increased soluble carbohydrate content compared to uninfested controls at 2 h and 2 d (t-test: P = .002, 0.04, 0.13, Figure 2II). The enhancement in soluble carbohydrate content in infested maize leaves was 44.35% and 29.36% higher than uninfested controls at 2 h and 2 d, respectively. Depending on ANOVA analysis, soluble carbohydrate content decreased significantly by increasing infestation time in maize leaves reaching its minimum value at 1 week. In tomato leaves, soluble carbohydrate content decreased significantly after 2 d compared to 2 hof S. exigua infestation, while no further decrease observed after 1 week. (ANOVA: F = 21.85, 276.53; P = .002, 0.002, ).
Figure 2. Soluble carbohydrate concentration (mg/g DW) of tomato (i) and maize (II) as affected by Spodoptera exigua larvae infestation after different periods of time. Each point is a mean of three replicates ± standard error. The different letters represent the statistical significance between different time periods of uninfested (a–c) and infested (a–c) plants at P ≤ 0.05. Asterisks show significant differences between uninfested and infested plants at each time period (*p < .05; **p < .01; ***p < .001).
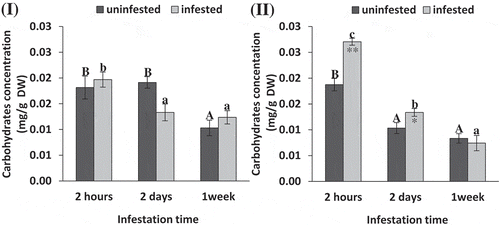
Effect of S. exigua infestation on the level of soluble proteins
After 2 h of infestation soluble proteins contentsignificantly increased in tomato leaves (t-test: P = .005, 0.188, 0.164, ) by about 37.18% higher than uninfested control. While in maize, no significant differences recorded in soluble protein content due to S. exigua infestation compared to uninfested plants at all observed time periods (t-test: P = .24, 0.48, 0.45, Figure 3II). As inferred from ANOVA analysis, the soluble protein content recorded in tomato and maize plant after 2 d of S. exigua feeding recorded the lowest values compared to 2 h and 1 week (ANOVA: F = 126.08, 85.53; P = .000, 0.000, ).
Figure 3. Soluble protein concentration (mg/g DW) of tomato (i) and maize (II) as affected by Spodoptera exigua larvae infestation after different periods of time. Each point is a mean of three replicates ± standard error. The different letters represent the statistical significance between different time periods of uninfested (a–c) and infested (a–c) plants at P ≤ 0.05. Asterisks show significant differences between uninfested and infested plants at each time period (*p < .05; **p < .01; ***p < .001).
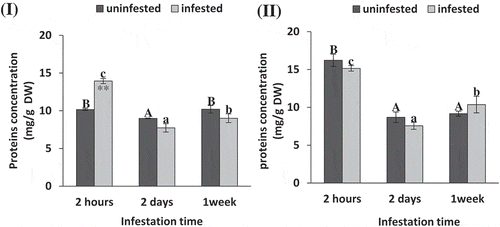
Effect of S. exigua infestation on the level of free amino acids
Free amino acid content at different periods of herbivory was significantly different in tomato leaves as affected by S. exigua infestation (t-test: P = .016, 0.015, 0.031) and infestation time (ANOVA: F = 276.90, P = .000). At 2 h, 2 d and 1 week infested tomato leaves showed a significant reduction of amino acid by about 35.42%, 15.91%, and 10.21%, respectively, lower than their corresponding controls. By increasing time period amino acid content increased in tomato leaves, reaching its maximum value after 1 week of infestation (). In maize, S. exigua infestation significantly enhances amino acid content after 2 h and 2 d by about 51% and 21% higher than their corresponding unifested control (t-test: P = .0003, 0.033, 0.538). Free amino acid content in infested maize leaves significantly enhanced after 2 d and 1 week of infestation compared to 2 h (ANOVA: F = 166.40, P = .000, Figure 4II).
Figure 4. Free amino acid concentration (μg/g DW) of tomato (i) and maize (II) as affected by Spodoptera exigua larvae infestation after different periods of time. Each point is a mean of three replicates ± standard error. The different letters represent the statistical significance between different time periods of uninfested (a–c) and infested (a–c) plants at P ≤ 0.05. Asterisks show significant differences between uninfested and infested plants at each time period (*p < .05; **p < .01; ***p < .001).
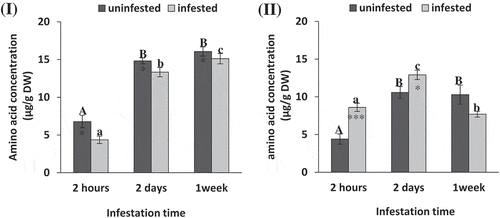
Effect of S. exigua infestation on the level of jasmonates
To investigate the possible roles played by jasmonate derivatives in response to S. exigua infestation, HPLC-MS/MS analysis carried out to determine JA, MeJA, and JA-L-Ile concentrations in tomato and maize leaves of infested and uninfested control plants under specific time periods. Analytes identification and quantitation depend on chromatograms shown in . and reveal that JA and JA-L-Ile contents were less than the detection limit in healthy tomato and maize plants, while MeJArecorded in high levels. On the other hand, S. exigua infestation induced JA, MeJA, and JA-L-Ile accumulation in both test plants in varying patterns.
Table 1. Concentrations of jasmonic acid (JA), methyl-JA (MeJA), and jasmonoyl-L-isoleucine JA-L-Ile (μg/mg DW), in tomato leaves as affected by Spodoptera exigua larvae infestation after different periods of time.
Table 2. Concentrations of jasmonic acid (JA), methyl-JA (MeJA), and jasmonoyl-L-isoleucine (JA-L-Ile) (μg/mg DW), in maize leaves as affected by Spodoptera exigua larvae infestation after different periods of time.
Figure 5. HPLC chromatogram analysis jasmonates. Peak No. (a), jasmonic acid; (b), methyl-jasmonate; (c), jasmonoyl-L-isoleucine.
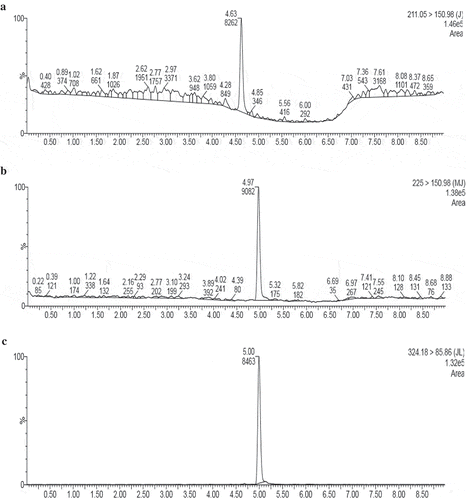
Depending on the t-test data, JA, MeJA, and JA-L-Ile of infested tomato (t-test: JA, p = .000, 0.000, 0.000; MeJA, p = .003, 0.048, 0.002; JA-L-Ile, p = .000, 0.000, 0.000, ) and maize (t-test: JA, p = .000, 0.000, 0.000, MeJA, p = .001, 0.002, 0.001; JA-L-Ile, P = .000, 0.000, 0.000, ) plants significantly increased higher than controls at all infestation periods. Jasmonic acid induction in infested leaves recorded its highest value after 2 h of infestation and by increasing infestation time its concentration significantly decreased reached minimum value at 1 week both in tomato (ANOVA: F = 1270.53, P = .000, ) and maize (ANOVA: F = 13749, P = .000, ). It is worth to mention that JA concentration in infested maize leaves was higher than that in tomato by about one and half order of magnitude at all studied time periods.
Similarly, MeJA accumulation due to S. exigua infestation recorded its highest value after 2 h, then significantly decreased by increasing infestation time recorded its minimum after 1 week in tomato (ANOVA: F = 1188.7, P = .000, ) and maize (ANOVA: F = 508.40, P = .000, ) leaves. MeJA concentration in infested tomato leaves was 70.62%, 57.62%, and 50.52% higher than that in uninfested control after 2 h, 2 d, and 1 week of infestation, respectively. In maize, MeJA concentration in infested leaves was 20.89%, 21.72%, and 10.12% higher than uninfested controls at 2 h, 2 d, and 1 week, respectively. By increasing infestation time, JA-L-Ile concentration showed a significantly steady decrease reached minimum value after 1 week in tomato leaves (ANOVA: F = 1521.7, P = .000, ) and significant increase reached maximum value after 1 week in maize (ANOVA: F = 39.20, P = .000, ). Remarkably, MeJA and JA-L-Ile in infested tomato leaves were considerably higher than that in maize at all studied time periods ( and ).
Discussion
Under stressful conditions, the photosynthetic rate in cultivated plants may be decreased due to the destruction of chlorophyll. Insect attack causes chlorophyll damage as a consequence of feeding damage to the mesophyll tissue of the infested leaves; however, plants could compensate by increasing chlorophyll content of the remaining undamaged leaf tissues.Citation29 Earlier studies have shown that chlorophyll b, the main component of the photosystems, is damaged more than chlorophyll a in stressed plants.Citation30 In addition, previous studies revealed that the elevation in chlorophyll content in the process of pigment degradation could be due to the conversion of chlorophyll b to chlorophyll a.Citation31 Hence, Chl a/b ratio could be used as a more reliable indicator for photosynthetic response to different stress conditions. In this study, the ratio of Chl a/b in infested tomato leaves was almost same as that in control plants at all studied time periods. However, in maize, Chl a/b ration significantly reduced due to S. exigua infestation after 2 h compared to control. In general, the reduction in Chl a/b ratio in a given species increases its ability to cope with the stress conditions.Citation32 Therefore, our results suggest that the S. exigua attack did not cause significant structural changes in the photosynthetic apparatus of tomato, while maize shows a greater adaptability at the beginning of the infestation.
Supporting Chl a/b results in our study, carbohydrate content in infested tomato leaves did not decrease significantly under all the studied time periods and in maize, carbohydrate content significantly increased more than control at 2 h and 2 d indicating higher tolerance in maize compared to tomato. In the same context,Citation33 El-Zohri et al. demonstrated that maize plant was more tolerant of S. exigua attack than tomato.Citation34 Jyothsna et al. showed that the variance in carbohydrates content as a result of insect attack was linked to the degree of feeding injury in plants and plant tolerance. In accordance with our results, herbivory increased significantly carbohydrate concentration in castor.Citation35 However, the levels of carbohydrates in sweet potato plant infestation by S. litura reduced lower than normal healthy plants.Citation36 The reduction in soluble carbohydrate content observed in this study by increasing experimental time in both infested and control plants could attribute to the consumption of this primary metabolite in plant growth; therefore, soluble carbohydrates could be incorporated into structural form to continue to shoot and root growth.
Based on chen et al.,Citation37 plants accumulate proteins in response to herbivory attack, these proteins could affect feeding pest’s digestive physiology. The enhancement in protein content in infested plants more than healthy controls has shown in many studies.Citation38,Citation39 In our study, soluble protein accumulation due to S. exigua infestation was recorded only in tomato leaves after 2 h of infestation. High levels of free amino acids are proved to have toxic effects on herbivore; therefore, it has a potential role in defining the suitability of the infested plants to insect infestation.Citation34,Citation40 After larval feeding, S. litura infested plants recorded raised quantities of foliar free amino acids higher than undamaged plants.Citation36 In the current study, the free amino acid quantity significantly reduced as a result of S. exigua infestation in tomato leaves compared with control at all study periods.Citation41 Sogawa recorded that Nilaparvata lugens feeding cause a reduction in the free amino acids in rice plants. The results of our study support the idea that the plant’s defense response to insect attack increases by increasing the amino acid content. Where the reduction in amino acid content in infested tomato leaves was less than uninfested plant could illustrate in parts its low tolerance compared to maize that accumulated higher concentrations of free amino acids in infested plants after 2 h and 2 d of infestation more than their corresponding controls.
Defense responses of JAs in the same plant are differing according to the insect species.Citation21 Our results proved that different plant species show different patterns of JAs accumulation in response to the same insect. Depending on the results of this study, JA induced in response to S. exigua in both test plants; however, its concentration in infested maize (more resistant plant) was much higher than that in infested tomato leaves (more sensitive plant). Similarly, the induction of endogenous JA levels was recorded in tobacco in response to Manducta sexta herbivory.Citation42 On the other hand, MeJA concentration in infested tomato leaves was higher than that in maize. Depending on these data we suggest that JA plays the main role in increasing defense responses to S. exigua infestation as a direct signaling molecule; however, MeJA could play an indirect role by inducing JA accumulation. This suggestion could be supported by the results that reordered by kumari et al.,Citation43 who revealed that 13-lipoxygenase pathway was upregulated as a result of the oxidative burst that induced by MeJA leading to the induction of a fatty acid oxidation and the synthesis of hydroxy-oxylipins. Another explanation could be that Me-JA is a storage form for JA in the healthy cells before herbivory attack (MeJA rather than JA recorded in control plants). The defense response could be initiated by hydrolyzing MeJA to JA by esterase enzyme after infestation. This illustrates the decrease in MeJA content in infested maize compared to tomato, although they have comparable values in their controls, while JA in infested maize was higher than that in tomato. Wu et al.Citation8 found that the resistance of Nicotiana attenuata plant to Manduca sexta herbivore increases when the plant treated with MeJA. They conclude that the defense response stimulated by MeJA treatment is not stimulated directly by exogenously applied MeJA but by JA produced from MeJA demethylation. Future investigations on the transient effect of S. exiguainfestation on MeJA esterase activity in tomato and maize are strongly recommended and would help us to a greater degree of accuracy on this matter.
Although JA-L-Ile recorded in the current study only after S. exigua infestation, its level was much lower than JA and MeJA in the leaves of both test plants indicating a less efficient role for JA-L-Ile in defense responses to S. exigua attack. Koo and HoweCitation5, and Gfeller et al.Citation44, also showed that MeJA level was of the same magnitude as JA, whereas JA-L-Ile was present in levels an order of magnitude lower. Our results reported that all studied JAs decreased by increasing infestation time in tomato and maize in response to S. exigua attack. Similar results observed by Caarls et al.,Citation45 who concluded that plants can quickly shut off JA-dependent responses after their activation to prevent negative effects of high JA levels on growth and development.
Conclusion
We could conclude generally that maize plant shows greater tolerance to S. exigua infestation more than tomato. In the main while, both test plants display differential defense responses of JAs to S. exigua attack, where JA concentration in infested maize was much higher than that in infested tomato leaves. On the other hand, MeJA and JA-L-Ile concentrations in infested tomato leaves were higher than that in maize. JA and JA-L-Ile levels were less than the detection limit, while MeJA was recorded in high levels in control plants. Out of the studied jasmonates, JA suggested to be the most effective mediator for defense response to S. exigua attack in the studied plants.
Disclosure of potential conflicts of interest
No potential conflicts of interest were disclosed.
Additional information
Funding
References
- Browse J, Howe GA. New weapons and a rapid response against insect attack. Plant Physiol. 2008;146:1–8. doi:10.1104/pp.107.115683.
- Reinbothe C, Springer A, Samol I, Reinbothe S. Plant oxylipins: role of jasmonic acid during programmed cell death, defence and leaf senescence. FEBS J. 2009;276:4666–4681. doi:10.1111/j.1742-4658.2009.07193.x.
- Wasternack C. Jasmonates: an update on biosynthesis, signal transduction and action in plant stress response, growth and development. Ann Bot. 2007;100:681–697. doi:10.1093/aob/mcm079.
- de-Freitas TFS, Stoutb MJ, Sant’Anaa J. Effects of exogenous methyl jasmonate and salicylic acid on rice resistance to Oebalus pugnax. Pest Manag Sci. 2019;75:744–752. doi:10.1002/ps.5174.
- Koo AJ, Howe GA. Catabolism and deactivation of the lipid-derived hormone jasmonoyl-isoleucine. Front Plant Sci. 2012;3:19. doi:10.3389/fpls.2012.00019.
- Ma QH, Tian B, Li YL. Overexpression of a wheat jasmonate-regulated lectin increases pathogen resistance. Biochimie. 2010;92:187–193. doi:10.1016/j.biochi.2009.11.008.
- War AR, Taggar GK, Hussain B, Taggar MS, Nair RM, Sharma HC. Plant defense against herbivory and insect adaptations. AoB Plants. 2018:10. doi:10.1093/aobpla/ply037.
- Wu J, Wang L, Baldwin IT. Methyl jasmonate-elicited herbivore resistance: does MeJA function as a signal without being hydrolyzed to JA? Planta. 2008;227:1161–1168. doi:10.1007/s00425-008-0690-8.
- Farmer EE. Plant biology: jasmonate perception machines. Nature. 2007;448:659–660. doi:10.1038/448659a.
- Gatehouse JA. Plant resistance towards insect herbivores: a dynamic interaction. New Phytol. 2002;156:145–169. doi:10.1046/j.1469-8137.2002.00519.x.
- Bachmann A, Hause B, Maucher H, Garbe E, Vörös K, Weichert H, Wasternack C, Feussner I. Jasmonate-induced lipid peroxidation in barley leaves initiated by distinct 13-lox forms of chloroplasts. Biol Chem. 2002;383:1645–1657. doi:10.1515/BC.2002.185.
- Dave A, Graham IA. Oxylipin signaling: a distinct role for the jasmonic acid precursor cis-12-oxo-phytodienoic acid. Front Plant Physiol. 2012;3:42. doi:10.3389/fpls.2012.00042.
- Schaller F, Schaller A, Stintzi A. Biosynthesis and metabolism of jasmonates. J Plant Growth Regul. 2005;23:179–199. doi:10.1007/BF02637260.
- Thines B, Katsir L, Melotto M, Niu Y, Mandaokar A, Liu G, Nomura K, He SY, Howe GA, Browse J. JAZ repressor proteins are targets of the SCF(COI1) complex during jasmonate signalling. Nature. 2007;448:661–665. doi:10.1038/nature05960.
- Gish M, Moraes CMD, Mescher MC. Herbivore-induced plant volatiles in natural and agricultural ecosystems: open questions and future prospects. Curr Opin Insect Sci. 2015;9:1–6. doi:10.1016/j.cois.2015.04.001.
- Qin J, Wu M, Liu H, Gao Y, Ren A. Endophyte infection and methyl jasmonate treatment increased the resistance of Achnatherum sibiricum to insect herbivores independently. Toxins. 2019;11:7–21. doi:10.3390/toxins11010007.
- Staswick PE, Tiryaki I. The oxylipin signal jasmonic acid is activated by an enzyme that conjugates it to isoleucine in Arabidopsis. Plant Cell. 2004;16:2117–2127. doi:10.1105/tpc.104.023549.
- Wang L, Halitschke R, Kang JH, Berg A, Harnisch F, Baldwin IT. Independently silencing two JAR family members impairs levels of trypsin proteinase inhibitors but not nicotine. Planta. 2007;226:159–167. doi:10.1007/s00425-007-0477-3.
- Al-Kherb WA. Virulence bio-assay efficiency of beauveria bassiana and metarhizium anisopliae for the biological control of spodoptera exigua hübner(Lepidoptera: Noctuidae) eggs and the 1st instar larvae. Aust J Basic Appl Sci. 2014;8:313–323.
- Mardani-Talaei M, Nouri-Ganbalani G, Naseri B, Hassanpour M. Life history studies of the beet armyworm, Spodoptera exigua (Hübner) (Lepidoptera: Noctuidae) on 10 corn hybrids. J Entomol Res Soc. 2012;14:9–18.
- Bosch M, Berger S, Schaller A, Stintzi A. Jasmonate-dependent induction of polyphenol oxidase activity in tomato foliage is important for defense against Spodoptera exigua but not against Manduca sexta. BMC Plant Biol. 2014;257:257–274. doi:10.1186/s12870-014-0257-8.
- Ayoub A, Afroz A. Jasmonic acid pathway in plants: in response to wounding and insect attack. EC Microbiol. 2017;11:227–231.
- Lichtenthaler HK. Chlorophylls and carotenoids: pigment of photosynthetic biomembranes. In: Packer L, Douce R, editors. Methods in Enzymology. 1987. p. 350–382. doi:10.1016/0076-6879(87)48036-1.
- El-Sharkawi HM, Michel BE. Effects of soil water matric potential and air humidity on CO2 and water vapour exchange of two grasses. Photosynthetica. 1977;11:176–182.
- Fales FW. The assimilation carbohydrates and degradation of by yeast cells. J Biol Chem. 1951;193:113–124.
- Sctilegel HG. Die veiwertung organischer sauren durch chlorella im licht. Planta. 1956;47:510–526. doi:10.1007/BF01935418.
- Lowry OH, Rosenbrough NJ, Farr AL, Randall RJ. Protein measurement with the Folin phenol reagent. J Biol Chem. 1951;193:265–275.
- Moore S, Stein WH. Photometric ninhydrin method for use in the chromatography of amino acids. J Biol Chem. 1948;179:367–388.
- Ni X, Quisenberry SS, Heng-Moss T, Markell JP, Higley LG, Baxendale F, Sarath G, Klucas R. Dynamic change in photosynthetic pigments and chlorophyll degradation elicited by cereal aphid feeding. Entomol Exp Appl. 2002;105:43–53. doi:10.1046/j.1570-7458.2002.01031.x.
- Mauchamp A, Méthy M. Submergence-induced damage of photosynthetic apparatus in Phragmites australis. Environ Exp Bot. 2004;51:227–235. doi:10.1016/j.envexpbot.2003.11.002.
- Eckardt NA. A new chlorophyll degradation pathway. Plant Cell. 2009;21:700. doi:10.1105/tpc.109.210313.
- Terletskaya N, Zobova N, Stupko V, Shuyskaya E. Growth and photosynthetic reactions of different species of wheat seedlings under drought and salt stress. Period Biol. 2017;119:37–45. doi:10.18054/pb.v119i1.4408.
- El-Zohri M, Bafeel SO, Al-Zahrani W. Differential oxidative and biochemical responses of tomato and maize leaves to Spodopter aexigua herbivory. Pak J Bot. 2020;52(4).
- Jyothsna Y, Kapil M, Usha RP. Effects of herbivore feeding on biochemical and nutrient profile of castor bean, Ricinus communis L. plants. Allelopathy J. 2009;24:131–142.
- Ananthakrishnan G, Ravikumar R, Anand RP, Vengadesan G, Ganapathi A. Induction of somatic embryogenesis from nucellus-derived callus of Anacardium occidentale L. Sci Hortic. 1999;79:91–99. doi:10.1016/S0304-4238(98)00202-7.
- Sandhyarani K, Rani PU. Insect herbivory induced foliar oxidative stress: changes in primary compounds, secondary metabolites and reactive oxygen species in sweet potato Ipomoea batata (L). Allelopathy J. 2013;31:157–168.
- Chen H, Gonzales-Vigil E, Wilkerson CG, Howe GA. Stability of plant defense proteins in the gut of insect herbivores. Plant Physiol. 2007;143:1954–1967. doi:10.1104/pp.106.095588.
- Dam NMV, Raaijmakers CE, Putten WHVD. Root herbivory reduces growth and survival of the shoot feeding specialist Pieris rapae on Brassica nigra. Entomol Exp Appl. 2004;115:161–170. doi:10.1111/j.1570-7458.2005.00241.x.
- Singh P, Sinhal VK. Effect of aphid infestation on the biochemical constituents of mustard (Brassica juncea) plant. J Phytol. 2011;3:28–33.
- Huang T, Jander G, Vos M. Non-protein amino acids in plant defense against insect herbivores: representative cases and opportunities for further functional analysis. Phytochemistry. 2011;72:1531–1537. doi:10.1016/j.phytochem.2011.03.019.
- Sogawa K. Effects of feeding of the brown plant hopper on the components in the leaf blade of rice plants. Jap J Appl Entomol Z. 1971;15:175–179. doi:10.1303/jjaez.15.175.
- Dicke M, van-Poecke RMP. Signaling in plant-insect interactions: signal transduction in direct and indirect plant defence. In: Scheel D, Wasternack C, editors. Plant signal transduction. Oxford University Press. 2002. p. 289–316.
- Kumari P, Reddy CR, Jha B. Methyl jasmonate-induced lipidomic and biochemical alterations in the intertidal macroalga Gracilaria dura (Gracilariaceae, Rhodophyta). Plant Cell Physiol. 2015;56:1877–1889. doi:10.1093/pcp/pcv115.
- Gfeller A, Dubugnon L, Liechti R, Farmer EE. Jasmonate biochemical pathway. Sci Signal. 2010;3(109):cm3. doi:10.1126/scisignal.3109cm3.
- Caarls L, Elberse J, Awwanah M, Ludwig NR, de Vries M, Zeilmaker T, Van Wees SCM, Schuurink RC, van den Ackervekan G. Arabidopsis JASMONATE-INDUCED OXYGENASES down-regulate plant immunity by hydroxylation and inactivation of the hormone jasmonic acid. Proc Natl Acad Sci USA. 2017;114:6388–6393. doi:10.1073/pnas.1701101114.