ABSTRACT
The hypericin is assumed as a highly demanded and key bioactive compound, which has antiviral, antimicrobial, antioxidant, and antitumor properties isolated from Hypericum perforatum. Nowadays, increasing bioactive molecules’ contents through generating novel compounds is one of the major research objectives of H. perforatum biotechnology; however, this plant remains recalcitrant and unmanageable to Agrobacterium mediated transformation and genetic improvement programs. In order to overcome these challenges, many researchers have focused on this unruly herb using biotic and abiotic eliciting strategies. Therefore, two experiments were separately designed for the evaluation of two types of abiotic elicitors, aiming at increasing the productivity of hypericin in the adventitious root suspension culture of H. perforatum. The first one was accomplished to evaluate the effect of UV-B light elicitors (the exposure time of 30, 60, and 90 min) and the recovery treatment (with or without) on hypericin content while the second one was assessed the effect of various temperatures (4°C, 8°C, 16°C, and 25°C) in three different exposure times (24 h, 72 h, and 7 d). Based on the results, UV-B (60 min) treatment followed by the recovery produced 0.430 µg/g DW hypericin and was distinguished as the most effective UV-B elicitation treatment. In addition, a temperature of 4°C for a period of 72 hours is required to get the highest amount of hypericin content. These findings indicate the fact that hypericin biosynthesis is notably affected by UV-B exposure time and Low-temperature. The data also clearly elucidate further mechanisms of hypericin production in H. perforatum adventitious root culture.
Introduction
Hypericum perforatum commonly known as St. John’s wort, is an important medicinal plant, which has been spread worldwide. From the Hypericum genus, only H. perforatum has been reported so far in the European Pharmacopoeia and is largely used to treat depression and other psychic disorders. Hypericum extracts such as hypericin are now in high demand.Citation1 Hypericin is a natural polycyclic aromatic dianthraquinone.Citation2 It is recognized as one of the most important compounds isolated from H. perforatum which together with hyperforin, have several health care properties such as antiviral, antimicrobial, antioxidant, and antitumor.Citation3 Furthermore, its other valuable pharmacological effects on diabetic surgical wound, depression, ulcer, burbs, hemorrhoids,Citation4 and short-term memoryCitation5 have also been statistically approved.
Hence, increasing bioactive molecules’ contents and generating novel compounds are the key features of H. perforatum biotechnology.Citation6 Despite available genome editing tools, H. perforatum has remained recalcitrant and unmanageable to Agrobacterium-mediated transformation and genetic improvement programs.Citation7 To overcome these challenges, various advances as well as precursor addition, hairy root culture, endophytes’ application, and scaling up bioreactor and immobilization were suggestedCitation8 which among them, biotic and abiotic eliciting strategies, have focused on this unruly herb.Citation7
Abiotic stresses related to environmental factors consist of light intensity, water accessibility, temperature (high or low), radiation (UV), gaseous toxins (ozone), pesticides, and metals (Ni, Cd, Co, Fe, Zn).Citation9 These stresses can promote the production of Reactive Oxygen Species (ROS). Moreover, some research carried out in different plants uncovered the possibility of enhanced production of secondary metabolites such as phenolic compounds, lignins, flavonones and flavonols, anthocyanins, stilbenes, alkaloids, terpenes and glucosinolates and cyanogenic glucosides under the UV-B radiation.Citation10
The light is an abiotic elicitor that can trigger the production of plant secondary metabolite.Citation11 Ultraviolet (UV) radiation stimulates secondary metabolite production. The UV-B radiated (6 h per day for 20 d) leaves of Aloe vera L. brought about the total content enhancement of anthraquinones.Citation12 UV-B possibly stimulates diverse changes in the plant metabolism and also the production of secondary metabolites which are frequently involved in the plant defense system such as anthraquinones, alkaloids, and flavonoids.Citation13 The total phenolic contents in P. vulgaris under the UV-B radiation were slightly higher than those contents in the control treatment.Citation2 Manaf et al.Citation14 also obtained similar results. By exposing four Alternanthera species to UV-B radiation for the duration of 0, 2, 4, 6, and 8 h Agati et al.Citation15 concluded that the maximum estimated flavonoid levels were obtained in A. sessilis exposed to UV-B radiation for 8 h, followed by a 24-h recovery phase. Höll et al.Citation16 examined the response of Arabidopsis plantlets to continuous UV-B and pulsed UV-B exposure (with intervening recovery periods). Their results showed that the pulsed UV-B results in flavonols’ accumulation increase. Thus, the recovery phase enhances the accumulation of stress-induced metabolites.
Flavonoid, tannin, and hypericin concentrations of St. John’s wort’ leaves, in the pots of sandy soil, were investigated under different UV-B radiation levels.Citation17 Also, the effect of UV-B light on hyperforin, hypericin, and pseudohypericin content in H. perforatum grown in a controlled growth chamber was studied.Citation18 The researchers revealed that significant transient metabolite concentration increases were induced by the UV light exposure.
Moreover, various experiments have underlined that plant growth parameters and secondary plant metabolites’ production are influenced by low temperature. Nevertheless, there are not sufficient research concerning temperature impacts on hypericin and hyperforin biosynthesis in H. perforatum. The effect of different temperatures on hypericin and hyperforin biosynthesis was evaluated by in vitro node culture of mentioned herb and the findings accentuated that the contents of hypericin and other bioactive compounds significantly increase along with temperature reduction.Citation19
In this study, in two separate experiments, the effects of UV-B light and the recovery treatment as well as low temperatures (<25°C) in different exposure times were systematically interrogated on H. perforatum for the first time.
Materials and methods
Plant’s materials and the culture conditions
The seeds prepared from the cultivar Topaz of Isfahan Pakan Seed Company, were used in this experiment. They were firstly sterilized with 70% ethanol and then with 10% sodium hypochlorite solution and were finally rinsed with sterile distilled water four to five times. Thereafter, the seeds were cultured in MS medium in sterile containers and in a growth chamber under the temperature of 25°C with 8 h’ dark interchange and 16 h of fluorescent light. To do adventitious root induction, the leaf explants of aseptic in vitro grown plantlets were transferred to Petri dishes, which contained ½ MS medium supplemented with one mgl−1 IBA and six gl−1 plant agar. All cultures were kept at 25 ± 1°C under the dark condition. After 2 weeks, primary adventitious roots were observed. After 4 weeks, approximately five mg of roots were sub-cultured in 250 ml Erlenmeyer flasks containing 50 ml of ½ MS medium liquid with the same ingredients as mentioned above. These cultures were carried out under similar conditions with previous step and were sub-cultured by 21 d’ intervals, aiming at greater biomass production.
UV-B radiation treatment
A laminar flow was equipped with UV-B Broadband lamp (Philips-TL 40 W/12 RS SLV with a peak at 302 nm). The lamp was placed 50 cm above the flasks containing three weeks old adventitious roots under aseptic condition. Then, in each treatment, a number of six flasks were exposed to UV-B for 30, 60, and 90 min at the same stage. Immediately after the radiation, half of the flasks used in each exposure time treatment were collected, rinsed with distilled water and fully dried at 60°C for 24 h and were stored at −80°C to be utilized for subsequent biochemical analysis. Remaining half of the flasks was transferred to the shaker for another one-week culture as the recovery treatment. Furthermore, the control treatment (no UV-B radiation) was kept on the shaker under the same above mentioned condition.
Low-temperature treatment
Another experiment was executed to evaluate the effect of low temperatures on hypericin yield in the root suspension culture of H. perforatum. The three weeks’ adventitious root cultures were exposed to the treatment of various temperatures including 4°C, 8°C, and 16°C. Each treatment was replicated three times for three different exposure times (24 h, 72 h, and 7 d). The root tissue went through biochemical analysis as stated earlier. The control treatment remained on the shaker under 25°C and 100 rpm and the dark condition.
Extract preparation and hypericin quantification
In both experiments, the sample of frozen root tissue was obtained from each treatment and was grinded into a fine powder. The powder (100 mg) was soaked in 20 ml methanol and then transferred to the ultrasonic apparatus for 1 h. Subsequently, the samples were centrifuged at 8000 rpm under the temperature of 4°C for 5 min. The supernatants were collected and their final volume was adjusted to 20 ml by adding methanol and was kept at 4°C for the measurement in following HPLC analysis. The hypericin quantification method was described elsewhere.Citation20
Statistical analysis
The present study was performed as a factorial experiment in a Randomized Complete Block Design (RCBD). All data were subjected to statistical analysis (ANOVA) using SAS statistical package, version 9.4, SAS Institute Inc., Cary, NC, USA. Means comparisons were performed by Least Significant Difference (LSD) test at 5% level. Values followed by the same letter(s) are not significantly different.
Results
Two experiments were separately designed for the evaluation of two types of abiotic elicitors on hypericin content of the root suspension culture of H. perforatum.
The effect of UV-B radiation time and the recovery treatment
According to &, the content of hypericin and dry weight were significantly affected (p < .01) by UV-B application as an abiotic elicitor, recovery, and their interaction.
Table 1. The ANOVA Procedure for the effect of UV-B radiation and recovery on the content of hypericin (µg/gDW) in the root suspension culture of H. Perforatum.
Table 2. The ANOVA Procedure for the effect of UV-B radiation and recovery on dry weight (g) in the root suspension culture of H. Perforatum.
The mean comparison of the interaction effect of UV-B radiation*recovery () showed that the highest content of hypericin (0.430 µg/gDW) was observed upon 60 min UV-B radiation with recovery. In addition, the t-test underlined that, all UV-B treatments’ recovery had a positive effect on hypericin concentration at the p-value of 0.05 and the effectiveness was increased by increasing UV-B radiation duration. The recovery caused a statistically significant difference between UV-B (60 min) and UV-B (90 min); while, both treatments generated similar results without the recovery.
Figure 1. The interaction effect of UV-B radiation * recovery on the content of hypericin (µg/gDW) in the adventitious root culture of H. perforatum. Control, 30 min, 60 min, and 90 min denotes the UV-B radiation exposure times while without and with state the use of recovery, respectively.
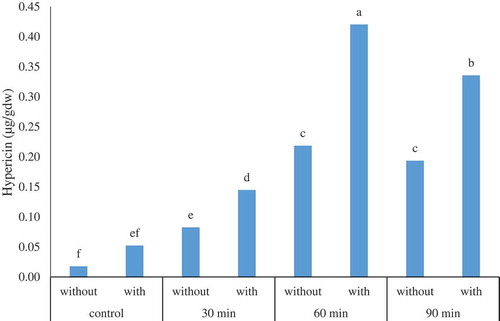
In addition, the means comparison among UV-B radiation and various recovery treatments’ effect () revealed the fact that the highest dry weight (0.5633 g) was achieved in 60 min UV-B radiation with recovery which is an agreement with hypericin content result.
Figure 2. The interaction effect of UV-B radiation * recovery on the dry weight (g) in adventitious root culture of H. perforatum. Control, 30 min, 60 min, and 90 min denote the UV-B radiation exposure times while without and with state the use of recovery, respectively. Values followed by the same letter(s) are not significantly different (LSD, 0.05).
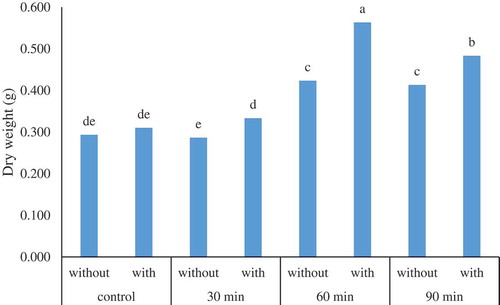
The hypericin production obtained from the most appropriate treatment was nearly eight times bigger than its amount in the control condition ().
The effect of low-temperature treatments and the durations
The results (&) illustrated that hypericin content and dry weight were significantly affected (p < .01) by low temperature, duration, and their interaction.
Table 3. The ANOVA Procedure for the effect of low temperature and duration of time on the content of hypericin (µg/gDW) in root suspension culture of H. Perforatum.
Table 4. The ANOVA Procedure for the effect of low temperature and duration of time on dry weight (g) in the root suspension culture of H. Perforatum.
The mean comparison among various temperature treatments indicated that four and 8°C treatments resulted in maximum hypericin content, respectively. This outcome displayed that as the temperature increases, the hypericin content decreases. On the other hand, the minimum hypericin content belonged to 16°C and 25°C treatments, respectively. The longer time length (7 d and 72 h) of the low temperatures produced the more hypericin content. In addition, as demonstrated in , increasing the temperature and decreasing the duration led to a significant dry weight decrease. The highest hypericin content (0.60 µg/gDW) was obtained through the temperature of 4°C for 7 d (). However, no significant differences were observed in hypericin content among 72 h and 7 d’ treatments at 4°C. This treatment was better than 24 h exposure to 4°C and 72 h and 7 d exposure to 8°C. At the temperature of 8°C, all duration times (24 h, 72 h, and 7 d) had the same effect on hypericin elicitation. Regardless of duration times, 16°C and 25°C showed the lowest amounts and statistically no significant difference was observed between them (). Hypericin content would enhance up to ten-fold by reducing the medium temperature from 25°C to 4°C.
Discussion
In this study, various exposure times (30, 60, and 90 min) to UV-B light, were tested and the hypericin level was increased to 8-fold by placing the root suspension culture of H. perforatum under the UV-B radiation. The effect of these radiation volumes, on overall dry weight was also positive. This bilateral effect of increasing dry weight and hypertine per gram dry weight indicates an effective strategy to achieve high levels of secondary metabolite production.
Zhang et al.21, proposed that increasing the total phenolic contents of P. vulgaris has beneficial effects on scavenging ROS generated by UV-B radiation. UV-B radiation increases hyperoside content in P. vulgaris. Therefore, enlarged hyperoside of P. vulgaris may perform as a compensator to scavenge the ROS produced by UV-B radiation.Citation21
Exposing to UV-B as a stress condition, triggers the UV-B specific pathways and results in various photomorphogenic events, DNA damage signals, and antioxidant enzymes’ activity to produce signaling messengers. UV-B photons absorbed by DNA can cause DNA modifications, which further leads to mutagenicity and altered metabolism.Citation22 These signaling cascades are able to excite the expression of several defense-responsive genes Citation23,as well as promoting plant survival under the UV-B radiation. At the first level, the genes responsible for the biosynthesis of pigments and UV-B absorbing secondary metabolites, act as a defense barrier, which would cause limited penetration of UV-B radiation into the plant tissues. For example, the enzymes required for the biosynthesis of phenolic compounds including phenylalanine ammonia lyase (PAL) and chalcone synthase (CHS) are over-expressed by the UV-B radiation.Citation24
The sensing of the elicitor signal recruits a signal transduction pathway and activates the transcription factors as the first response, and subsequently facilities the expression of genes responsible for producing defense secondary metabolites.Citation25 Based on the results of Klein et al.Citation26, the maximum flavonoid levels were attributed to Alternanthera sessilis, exposed to UV-B radiation for 8 h, followed by a 24-h recovery period. The authors concluded that, with the existence of the elicitor, plants could have activated genes' transcription for betacyanins’ biosynthesis route. The higher level of betacyanins over the time also might be related to an increase in their levels within the plants after the recovery period. Thus, it is expected that the concentration of UV-absorbing secondary metabolites would be increased under the UV stress condition, which could play the role of potent antioxidants that quickly neutralize radicals.Citation27
Our results also indicated that along with increasing the UV-B exposure time from 60 to 90 min, the hypericin content was no significantly altered. Nevertheless, after the recovery, the samples with 60 min exposure time were able to produce notably higher levels of hypericin than the samples with 90 min exposure time. The reason might be that less exposure time, results in less damage to the plant system. The recovery after 90 min exposure time also increased the product, although its increase was not comparable with the recovery after 60 min of UV-B treatment. This could be due to the toxicity of longer time of UV-B radiation. Following the constant stress exposure, plants show diminished metabolic stress responses due to regulatory feedback loops.Citation26 The effects of UV-B on secondary metabolites of H. perforatum grown-up in controlled environments were studied by Brechner et al.Citation18. In their research, various single exposure periods for UV-B irradiation (10, 20, 40, 80, and 160 min) were applied and the hypericin content was measured. It was revealed that 40 min exposure time brings about the maximum increase comparing the control and other longer exposure time treatments (80 or 160 min). As a negative consequence, a tissue injury was detected at the tips of plants’ leaves.
Many researchers have confirmed that low temperatures (cold stress) affect the metabolic activity and rise secondary metabolite production. According to , the hypericin-content has reached its highest amount under the treatment of 4°C for 7 d. Since this value is significantly equivalent with the treatment of 4°C for 72 h, for economic efficiency reasons, 72 h exposure times at 4°C is suggested to enhance hypericin production performance. However, it is seen that by increasing the temperatures from four to 25°C or by falling the duration of low temperature, the hypericin content and dry weight have been reduced (, ). Through the comparison of four and 8°C temperatures, it can be concluded that the temperature duration has a significant role and provides appropriate circumstance for a better temperature elicitation performance. St. john’s wort is a freezing-tolerant plant and naphthodianthrones’ hypericin and pseudohypericin are increased when the plants are exposed to minus 4°C for 48 h as a consequence of a systemic defense in the freezing-tolerant hypericin-producing species.Citation28 The accumulation of anthocyanins after the exposure to Mikania micrantha at 4°C (12 h), was proposed as an adaptation approach during low winter temperatures.Citation21 Temperature-dependent growth and hypericin biosynthesis in H. perforatum have been implemented. After exposing plants’ seedlings to three different temperatures (15°C, 22°C, and 30°C), the maximum amounts of aerial biomass was recorded at 15°C (the lowest temperature) with 1.3 and 1.6 fold increase compared to the temperatures of 22°C and 30°C. Authors indicated that Hyp-1 gene involved in hypericin biosynthesis is up-regulated at 15°C.Citation19 These results support our findings, where they underline that the decrease in hypericin content occurs as the temperature increases. This can be associated with better stimulation of the genes involved in signaling pathways in response to low temperatures as a cold stress. Low temperatures have the potential to increase the transcripts of flavonoid biosynthetic genes including PAL and CHS within the leaves and stems of Arabidopsis thaliana.Citation29
Figure 4. The interaction effect of low temperatures * durations on the dry weight (g) in root suspension culture of H. perforatum. 4°C, 8°C, 16°C, and 25°C denote the low temperatures while 1 week, 72 h and 24 h state the duration of low temperatures, respectively.
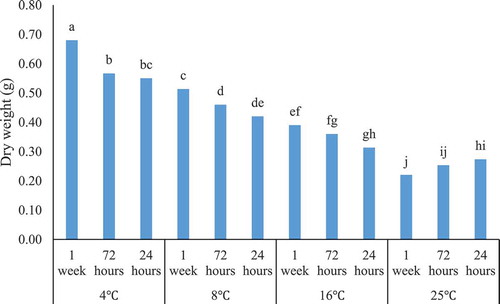
The role of flavonoids in freezing tolerance and cold acclimation in plants has been proved.Citation30 This function of secondary metabolites has been raised mostly for plant-chilling tolerance responses in contradiction with low temperatures above 0°C. Since flavonoids are localized in the vacuole, nucleus, and chloroplast, they could increase leaves’ tolerance to low temperatures through cell membrane protection against freezing and by protein stability increase via preventing their aggregation.Citation15
Overall, it could be concluded that low temperatures are able to raise hypericin content greater than UV-B radiation.
Conclusion
It seems that active components of elicitors have an effective role in defense responses’ acceleration and enhancement of secondary metabolites’ production. In recent years, it has been accentuated that some elicitors could expedite the production of new secondary metabolites in the plant. Due to this phenomenon, the findings of this research, underline significant role of low temperature and UV-B in the quality and medicinal value improvement of Hypericum perforatom.
Considering the costs of UV-B lamp equipment installation and comparing secondary metabolite growth measured in low temperature and UV-B radiation treatments, lowering the temperature is a better economical way for hypericin production increase. Moreover, hypericin proliferation is higher in a lower temperature treatment than the radiation treatment.
Eventually, it is recommended that researchers continue to focus on applying both low temperatures and UV-B treatments simultaneously.
Correction Statement
This article has been republished with minor changes. These changes do not impact the academic content of the article.
Additional information
Funding
References
- Rizzo P, Altschmied L, Stark P, Rutten T, Gündel A, Scharfenberg S, Franke K, Bäumlein H, Wessjohann L, Koch M, et al. Discovery of key regulators of dark gland development and hypericin biosynthesis in St. John’s Wort (Hypericum perforatum). Plant Biotechnol J. 2019;17(12):1–7. doi:10.1111/pbi.13141.
- Zhang Y, Shang K, Wu X, Song S, Li Z, Pei Z, Pei Y. Highly efficient green synthesis and photodynamic therapeutic study of hypericin and its derivatives. RSC Adv. 2018;8(39):21786–21792. doi:10.1039/C8RA03732A.
- Napoli E, Siracusa L, Ruberto G, Carrubba A, Lazzara S, Speciale A, Cimino F, Saija A, Cristani M. Phytochemical profiles, phototoxic and antioxidant properties of eleven Hypericum species–A comparative study. Phytochemistry. 2018;152:162–173. doi:10.1016/j.phytochem.2018.05.003.
- Altıparmak M, Eskitaşçıoğlu T. Comparison of systemic and topical Hypericum perforatum on diabetic surgical wounds. J Invest Surg. 2018;31(1):29–37. doi:10.1080/08941939.2016.1272654.
- Yechiam E, Ben-Eliezer D, Ashby NJ, Bar-Shaked M. The acute effect of Hypericum perforatum on short-term memory in healthy adults. Psychopharmacology. 2019;236(2):613–623. doi:10.1007/s00213-018-5088-0.
- Shakya P, Marslin G, Siram K, Beerhues L, Franklin G. Elicitation as a tool to improve the profiles of high‐value secondary metabolites and pharmacological properties of Hypericum perforatum. J Pharm Pharmacol. 2019;71(1):70–82. doi:10.1111/jphp.12743.
- Hou W, Shakya P, Franklin G. A perspective on Hypericum perforatum genetic transformation. Front Plant Sci. 2016;7:879. doi:10.3389/fpls.2016.00879.
- Hussain MS, Fareed S, Saba Ansari M, Rahman A, Ahmad IZ, Saeed M. Current approaches toward production of secondary plant metabolites. J Pharm Bioallied Sci. 2012;4(1):10. doi:10.4103/0975-7406.92725.
- Espinosa-Leal CA, Puente-Garza CA, García-Lara S. In vitro plant tissue culture: means for production of biological active compounds. Planta. 2018;248(1):1–18. doi:10.1007/s00425-018-2910-1.
- Matsuura HN, de Costa F, Yendo ACA, Fett-Neto AG. Photoelicitation of bioactive secondary metabolites by ultraviolet radiation: mechanisms, strategies, and applications. In: Chandra S, Lata H, Varma A (eds) Biotechnology for medicinal plants. Springer; 2013. p. 171–190.
- Naik PM, Al-Khayri JM. Abiotic and biotic elicitors-role in secondary metabolites production through in vitro culture of medicinal plants. In: Shanker A, Shanker Ch (eds) Abiotic and Biotic Stress Plants-recent Adv. Future Perspect. 2016. p. 247–277.
- Hong-xing W, Tong-wen Y, Jing-yua L. Effects of enhanced UV-B radiation on leaf anthraquinones content and cell ultrastructure of Aloe vera L. Yingyong Shengtai Xuebao. 2010;21:260–264.
- Schreiner M, Martínez‐Abaigar J, Glaab J, Jansen M. UV‐B induced secondary plant metabolites: potential benefits for plant and human health. Optik & Photonik. 2014;9(2):34–37. doi:10.1002/opph.201400048.
- Manaf HH, Rabie KA, El-Aal MS. Impact of UV-B radiation on some biochemical changes and growth parameters in Echinacea purpurea callus and suspension culture. Ann Agr Sci. 2016;61(2):207–216. doi:10.1016/j.aoas.2016.08.001.
- Agati G, Azzarello E, Pollastri S, Tattini M. Flavonoids as antioxidants in plants: location and functional significance. Plant Sci. 2012;196:67–76. doi:10.1016/j.plantsci.2012.07.014.
- Höll J, Lindner S, Walter H, Joshi D, Poschet G, Pfleger S, Ziegler T, Hell R, Bogs J, Rausch T. Impact of pulsed UV‐B stress exposure on plant performance: how recovery periods stimulate secondary metabolism while reducing adaptive growth attenuation. Plant Cell Environ. 2019;42(3):801–814. doi:10.1111/pce.13409.
- Germ M, Stibilj V, Kreft S, Gaberščik A, Kreft I. Flavonoid, tannin and hypericin concentrations in the leaves of St. John’s wort (Hypericum perforatum L.) are affected by UV-B radiation levels. Food Chem. 2010;122(3):471–474. doi:10.1016/j.foodchem.2010.03.008.
- Brechner ML, Albright LD, Weston LA. Effects of UV‐B on secondary metabolites of St. John’s Wort (Hypericum perforatum L.) grown in controlled environments. Photochem Photobiol. 2011;87(3):680–684. doi:10.1111/j.1751-1097.2011.00904.x.
- Yao Y, Kang T, Jin L, Liu Z, Zhang Z, Xing H, Sun P, Li M. Temperature-dependent growth and hypericin biosynthesis in Hypericum perforatum. Plant Physiol Biochem. 2019;139:613–619. doi:10.1016/j.plaphy.2019.04.012.
- Sobhani Najafabadi A, Khanahmadi M, Ebrahimi M, Moradi K, Behroozi P, Noormohammadi N. Effect of different quality of light on growth and production of secondary metabolites in adventitious root cultivation of Hypericum perforatum. Plant Signal Behav. 2019;14(9):1640561. doi:10.1080/15592324.2019.1640561.
- Zhang Q, Zhai J, Shao L, Lin W, Peng C. Accumulation of anthocyanins: an adaptation strategy of Mikania micrantha to low temperature in winter. Front Plant Sci. 2019;10:1049. doi:10.3389/fpls.2019.01049.
- Britt AB, Chen JJ, Wykoff D, Mitchell D. A UV-sensitive mutant of Arabidopsis defective in the repair of pyrimidine-pyrimidinone(6–4) dimers. Science. 1993;261(5128):1571–1574. doi:10.1126/science.8372351.
- Jenkins GI. Signal transduction in responses to UV-B radiation. Annu Rev Plant Biol. 2009;60(1):407–431. doi:10.1146/annurev.arplant.59.032607.092953.
- Logemann JA, Pauloski BR, Rademaker AW, Colangelo LA, Kahrilas PJ, Smith CH. Temporal and biomechanical characteristics of oropharyngeal swallow in younger and older men. J Speech Lang and Hearing Res. 2000;43(5):1264–1274. doi:10.1044/jslhr.4305.1264.
- Zhao J, Davis LC, Verpoorte R. Elicitor signal transduction leading to production of plant secondary metabolites. Biotechnol Adv. 2005;23(4):283–333. doi:10.1016/j.biotechadv.2005.01.003.
- Klein FRS, Reis A, Kleinowski AM, Telles RT, Amarante L, Peters JA, Braga EJB. UV-B radiation as an elicitor of secondary metabolite production in plants of the genus Alternanthera. Acta Bot Brasilica. 2018;32(4):615–623. doi:10.1590/0102-33062018abb0120.
- Gandía-Herrero F, García-Carmona F, Escribano J. Floral fluorescence effect. Nature. 2005;437(7057):334. doi:10.1038/437334a.
- Bruňáková K, Petijová L, Zámečník J, Turečková V, Čellárová E. The role of ABA in the freezing injury avoidance in two Hypericum species differing in frost tolerance and potential to synthesize hypericins. Plant Cell, Tissue and Organ Culture (PCTOC). 2015;122(1):45–56. doi:10.1007/s11240-015-0748-9.
- Yang L, Wen K-S, Ruan X, Zhao Y-X, Wei F, Wang Q. Response of plant secondary metabolites to environmental factors. Molecules. 2018;23:762.
- Schulz E, Tohge T, Zuther E, Fernie AR, Hincha DK. Flavonoids are determinants of freezing tolerance and cold acclimation in Arabidopsis thaliana. Sci Rep. 2016;23(6):34027. doi:10.1038/srep34027.