ABSTRACT
The Aminophospholipid ATPase (ALA) family of plant lipid flippases is involved in the selective transport of lipids across membrane bilayers. Recently, we demonstrated that double mutants lacking both ALA4 and −5 are severely dwarfed. Dwarfism in ala4/5 mutants was accompanied by cellular elongation defects and various lipidomic perturbations, including a 1.4-fold increase in the accumulation of glucosylceramides (GlcCers) relative to total sphingolipid content. Here, we present a potential model for flippase-facilitated GlcCer catabolism in plants, where a combination of ALA flippases transport GlcCers to cytosolic membrane surfaces where they are degraded by Glucosylceramidases (GCDs). GCDs remove the glucose headgroup from GlcCers to produce a ceramide (Cer) backbone, which can be further degraded to sphingoid bases (Sphs, e.g, phytosphingosine) and fatty acids (FAs). In the absence of GlcCer-transporting flippases, GlcCers are proposed to accumulate on extracytoplasmic (i.e., apoplastic) or lumenal membrane surfaces. As GlcCers are potential precursors for Sph production, impaired GlcCer catabolism might also result in the decreased production of the secondary messenger Sph-1-phosphate (Sph-1-P, e.g., phytosphingosine-1-P), a regulator of cell turgor. Importantly, we postulate that either GlcCer accumulation or reduced Sph-1-P signaling might contribute to the growth reductions observed in ala4/5 mutants. Similar catabolic pathways have been proposed for humans and yeast, suggesting flippase-facilitated GlcCer catabolism is conserved across eukaryotes.
KEYWORDS:
Text
P4-type ATPases (i.e., lipid flippases) are enzymes that consume ATP to facilitate the selective transport of lipids across membrane bilayers, specifically the movement from non-cytosolic surfaces to cytosolic surfaces. In plants, flippases are referred to as Aminophospholipid ATPases (ALAs), and require ALA-Interacting Subunits (ALISs) for proper localization and activity.Citation1,Citation2 Arabidopsis (Arabidopsis thaliana) has 12 ALAs distributed among three of the eight clades comprising eukaryotic flippases, P4A-b, P4A-e, and P4 C,Citation3 which can be further divided into five phylogenetic clusters conserved in angiosperms ().Citation27 Flippases from each of these clusters show both overlapping and distinct lipid transport activities.Citation5,Citation28 For example, while flippases from all five ALA clusters appear to transport at least some phosphatidylserine (PS), only members from cluster 2, 3, or 4 appear to transport phosphatidylcholine (PC).Citation2,Citation5,Citation29-Citation31
Figure 1. Model showing a role for ALA flippases in GlcCer catabolism.
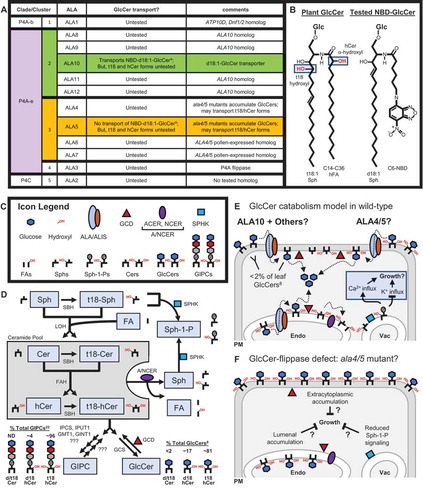
Distinct plant phenotypes have now been reported for lines deficient in each specific ALA cluster. For example, RNAi lines that target ALA1 (P4A-b; cluster 1) display vegetative growth reductions under chilling stress,Citation29 ala2 mutants (P4C; cluster 5) have increased sensitivity to viral pathogens,Citation32,Citation33 ala3 mutants (P4A-e; cluster 4) have disrupted vesicular trafficking and reductions in vegetative growth and pollen fertility,Citation34–Citation36 ala6/7 double mutants (P4A-e; cluster 3) have reduced pollen fertility,Citation37 and ala10 mutants (P4A-e; cluster 2) have perturbed stomatal conductance and membrane desaturation levels.Citation31,Citation38 There is also evidence that an ala3/4/5/9/10/11 mutant (P4A-e; cluster 2, 3, and 4) is dwarfed and non-viable, suggesting members from the P4A-e clade together are essential to Arabidopsis.Citation39
Recently, we demonstrated that the loss of both ALA4 and −5 (ala4/5 mutant; P4A-e; cluster 3) is sufficient to cause severe plant dwarfism and reduced cell growth.Citation5 These growth defects correlated with changes in the concentrations of various membrane lipids, including perturbations in glycosphingolipids that are thought to be critical for vegetative growth and development.Citation13,Citation40 Specifically, ala4/5 mutants displayed 1.4-fold increases in glucosylceramides (GlcCers; ) and corresponding 1.3-fold decreases in glycosylinositolphosphoceramides (GIPCs), relative to total sphingolipid content. While it is not yet clear why GlcCer levels are increased in ala4/5 mutants, or whether this specific sphingolipid accumulation (i.e., sphingolipidosis) is the underlying cause of ala4/5 mutant dwarfism, we speculated that ALA4 and −5 might flip GlcCers to facilitate their catabolism on cytosolic membrane surfaces. Importantly, this speculation does not rule out the potential for ALAs to transport GIPCs, or for ala4/5 mutants to be dwarfed due to GIPC reductions.
Here we offer a working model for GlcCer catabolism in plant cells that integrates a potential role for ALA flippases (). A key feature of this model is a need to flip GlcCers across a membrane to facilitate their degradation into glucose and a ceramide backbone (Cers). Once cytosolically exposed, glucose removal could be catalyzed by glucosylceramidases. In Arabidopsis, there are four genes encoding Glucosylceramidases (GCDs) that show homology to a well-characterized mammalian glucosylceramidase, named GBA2. For GCD3, the GlcCer-degrading activity has been confirmed and a GFP-fusion was shown to localize to the ER and PM,Citation14 likely on cytosolic membrane surfaces similar to GBA2.Citation41 After glucose removal, the Cer portion is then further degraded to a sphingoid base (Sph, e.g., phytosphingosine) and a fatty acid (FA) by Golgi/ER-localized Neutral Ceramidases (NCERs)Citation16,Citation17 and two phylogenetically distinct Alkaline Ceramidases (ACERs), named ACER1Citation18 and Turgor Regulation Defect 1 [TOD1;Citation15]. Sphs can also be phosphorylated by tonoplast-localized Sphingosine Kinases (SPHKs) to produce Sph-1-phosphate (Sph-1-P, e.g., phytosphingosine-1-P),Citation19–Citation21 a turgor-regulating secondary messenger that stimulates Ca2+ influxCitation23 and inhibits K+ influx.Citation24 We propose that a GlcCer-flippase deficient mutant might result in a GlcCer-catabolism defect that 1) increases GlcCer concentrations on lumenal and/or extracytoplasmic (i.e., apoplastic) membrane surfaces, and 2) reduces the production of GlcCer-catabolism byproducts, including signaling molecules such as Sphs and Sph-1-Ps. We speculate that either GlcCer accumulation or reduced Sph signaling could reduce plant growth and contribute to the underlying mechanism of dwarfism in ala4/5 mutants ().
An important feature of this model is the predicted spatial separation between GlcCers and the GlcCer-degrading GCDs, which require an ALA lipid flippase for convergence. Evidence suggests GlcCers accumulate on the apoplastic surface of the plant PM under normal growth conditions,Citation42,Citation43 where they are protected from any cytosol-localized degrading enzymes. Other plant membranes known to accumulate GlcCers include the vacuole and other parts of the endomembrane system, Citation25,Citation44,Citation45 but assays have not been performed to determine the leaflet-specific distribution of GlcCers in these membranes. As for GCDs, while GCD3-GFP fusions have been shown to localize to PM and endomembrane systems,Citation14 these experiments did not address leaflet-specific localization. GCD1, −2, and −4 also remain uncharacterized for activity or localization. However, studies on the mammalian homolog GBA2 suggest that this class of enzymes localizes to cytosolic membrane surfaces,Citation41 which provides an expectation that plant GCDs also have cytosolic orientations.
In the proposed model, it is not yet clear which ALAs are directly transporting GlcCers (). ALA10 from cluster 2 is the only plant flippase with demonstrated GlcCer transport activity, shown via the uptake of a fluorescent NBD-d18:1-GlcCer substrate in yeast.Citation4 ALA1 from cluster 1 might also transport GlcCers as it has the highest sequence homology to ATP10D (human) and Dnf1/2 (yeast),Citation3 which have been shown to transport GlcCers.Citation46 ALA3 might also flip GlcCers as they belong to the same hierarchal P4A flippase clade as Dnf1/2, ATP10D, and ALA10.Citation3 Currently, most ALAs have not been tested for GlcCer transport activity ().
As to whether ALA4 or −5 from cluster 3 can transport GlcCers, ALA5 failed to show detectable uptake of an NBD-d18:1-GlcCer substrate in a yeast assay, despite showing uptake for at least one other NBD-labeled sphingolipid (sphingomyelin – a non-plant lipid).Citation5 However, evidence suggests that some lipid flippase activities are not reliably detected by fluorescent lipid uptake assays. For example, ALA5 failed to show uptake of an externally supplied NBD-PS substrate when expressed in a yeast flippase mutant, but in an independent assay still showed the capacity to flip and remove endogenous PS from the extracytoplasmic surface of these same lines and confer resistance to the toxin papuamide A.Citation5
The sphingolipid test substrate NBD-d18:1-GlcCer is also an imperfect proxy for plant sphingolipids, which have several important variations that could alter substrate recognition by ALA flippases (). For example, plant GlcCers are modified by hydroxylation events near the head group. One modification, α-hydroxylation of the FA chain by Sphingolipid-FA Hydroxylase 1 and −2 (FAHs) converts Cers to hydroxyCers (hCers), a modification that occurs in >98% of GlcCers in Arabidopsis leaves.Citation8 Additionally, C4-hydroxylation of the Sph chain by Sphingoid Base Hydroxylase 1 and −2 (SBHs) converts d18 sphingolipids to t18, a modification that occurs in ~81% of GlcCers in Arabidopsis leaves.Citation8 Interestingly, both fah1/2 mutantsCitation8 and sbh1/2 mutantsCitation6 display growth defects, suggesting that these modifications are important for plant growth. Thus, it remains possible that ALA4 and −5 could still facilitate the transport of t18-GlcCer or d/t18-Glc-hCer plant sphingolipids, despite the inability to recognize a related NBD-labeled test substrate.
Our working model that correlates ala4/5 mutant growth defects to reductions in GlcCer catabolism predicts several other mutations that might cause similar sphingolipidosis-related dwarfisms. For example, the model predicts that a mutant lacking all four GCDs would be completely deficient in GlcCer catabolism, and thus should accumulate GlcCers and be dwarfed like ala4/5 mutants. At present, only knockdown mutants in GCD3 have been characterized, which failed to show any obvious growth defects.Citation14 The normal growth associated with gcd3 mutants might indicate that the four GCDs in Arabidopsis provide some degree of functional redundancy. Thus, a mutant combination that is completely lacking all four GCDs is needed to determine whether a disruption in this first stage of GlcCer catabolism can partially pheno-mimic an ala4/5-like growth deficiency.
The model also predicts that mutants lacking signaling components downstream of GCD activity might show dwarfism. However, functional redundancy might also be an issue for finding phenotypes associated with the loss of three NCERs and two ACERS. At present, no ala4/5-like growth phenotypes have been reported for single mutants of ncer1,Citation16 ncer2, Citation47 acer1,Citation18 or tod1 (an ACER),Citation15 or for an ncer1/2 double mutant.Citation47 Similarly, double mutants lacking both SPHK1 and −2 might show growth defects, due to a complete deficiency in direct Sph-1-P synthesis. However, at present only sphk1 and sphk2 single mutants have been characterized, both of which have normal vegetative growth.Citation48,Citation49 Thus, additional genetic studies are needed to test the relative importance of each subsequent stage in GlcCer catabolism.
It is also worth considering the changes in sphingolipid metabolite concentrations predicted by this model. Besides GlcCer increases, a GlcCer-catabolism deficiency is expected to decrease the concentrations of hCer and Sph byproducts. While neither of these changes were observed in ala4/5 mutants, evidence suggests plants compensate for reduced sphingolipid production by reducing growth, as seen in RNAi lines deficient in the Sph biosynthesis-gene Long Chain Base 1 (LCB1), which are dwarfed but still have normal Sph/sphingolipid contents.Citation22
While the model illustrated here is for a plant cell, the potential importance of flippase-facilitated GlcCer catabolism is not restricted to plants. In humans, mutations in the flippase ATP10D have been correlated with elevated plasma GlcCer levels.Citation50 Recently, ATP10D was confirmed to transport GlcCers,Citation46 and a similar model for GlcCer catabolism in humans has been proposed where ATP10D flips GlcCers across membranes to facilitate their cytosolic degradation by GBA2. Additionally, Saccharomyces cerevisiae has two flippases, Dnf1p and −2p, that can uptake GlcCers,Citation46 and at least one cytosolic enzyme capable of glucosylceramidase activity, Egh1.Citation51 As S. cerevisiae is one of several yeast species that lack GlcCers,Citation52,Citation53 the continued presence of an enzyme for GlcCer uptake might indicate a functional role in the context of nutrition or perception of other organisms. However, other yeast species such as Cryptococcus neoformans still produce endogenous GlcCers, and their homologs of Dnf1p/2pCitation54 and Egh1Citation55 might still function in the context of a flippase-facilitated GlcCer homeostasis pathway. Thus, similar flippase-facilitated GlcCer-catabolism pathways appear to be conserved in plants, animals, and at least some fungi.
Additional information
Funding
References
- López-Marqués RL, Poulsen LR, Palmgren MG. A putative plant aminophospholipid flippase, the arabidopsis p4 atpase ala1, localizes to the plasma membrane following association with a β-subunit. PLoS One. 2012;7(4):1. doi:10.1371/journal.pone.0033042.
- Poulsen LR, López-Marqués RL, McDowell SC, Okkeri J, Licht D, Schulz A, Palmgren MG. The Arabidopsis P4-ATPase ALA3 localizes to the golgi and requires a β-subunit to function in lipid translocation and secretory vesicle formation. Plant Cell. 2008;20(3):658–5. doi:10.1105/tpc.107.054767.
- Palmgren M, Østerberg JT, Nintemann SJ, Poulsen LR, López-Marqués RL. Evolution and a revised nomenclature of P4 ATPases, a eukaryotic family of lipid flippases. Biochimica Et Biophysica Acta Biomembr. 2019. doi:10.1016/j.bbamem.2019.02.006.
- Jensen MS, Costa SR, Duelli AS, Andersen PA, Poulsen LR, Stanchev LD, Palmgren GM, Pomorski TG, López-Marqués RL. Phospholipid flipping involves a central cavity in P4 ATPases. Sci Rep. 2017;7(1):17621. doi:10.1038/s41598-017-17742-y.
- Davis JA, Pares RB, Bernstein T, McDowell SC, Brown E, Stubrich J, Harper JF. The lipid flippases ALA4 and ALA5 play critical roles in cell expansion and plant growth. Plant Physiol. 2020. doi:10.1104/pp.19.01332.
- Chen M, Markham JE, Dietrich CR, Jaworski JG, Cahoon EB. Sphingolipid long-chain base hydroxylation is important for growth and regulation of sphingolipid content and composition in Arabidopsis. Plant Cell. 2008;20(7):1862–1878. doi:10.1105/tpc.107.057851.
- Markham JE, Molino D, Gissot L, Bellec Y, Hématy K, Marion J, Belcram K, Palauqui JC, Satiat-JeuneMaître B, Faure J-D. Sphingolipids containing very-long-chain fatty acids define a secretory pathway for specific polar plasma membrane protein targeting in Arabidopsis. Plant Cell. 2011;23(6):2362–2378. doi:10.1105/tpc.110.080473.
- König S, Feussner K, Schwarz M, Kaever A, Iven T, Landesfeind M, Feussner I. Arabidopsis mutants of sphingolipid fatty acid α-hydroxylases accumulate ceramides and salicylates. New Phytol. 2012;196(4):1086–1097. doi:10.1111/j.1469-8137.2012.04351.x.
- Wang W, Yang X, Tangchaiburana S, Ndeh R, Markham JE, Tsegaye Y, Xiao S. An inositolphosphorylceramide synthase is involved in regulation of plant programmed cell death associated with defense in arabidopsis. Plant Cell. 2008;20(11):3163–3179. doi:10.1105/tpc.108.060053.
- Rennie EA, Ebert B, Miles GP, Cahoon RE, Christiansen KM, Stonebloom S, Scheller HV. Identification of a sphingolipid α-glucuronosyltransferase that is essential for pollen function in Arabidopsis. Plant Cell. 2014;26(8):3314–3325. doi:10.1105/tpc.114.129171.
- Fang L, Ishikawa T, Rennie EA, Murawska GM, Lao J, Yan J, Tsa AYi, Baidoo EEK, Xu J, Keasling JD, et al. Loss of inositol phosphorylceramide sphingolipid mannosylation induces plant immune responses and reduces cellulose content in arabidopsis. Plant Cell. 2016;28(12):2991–3004. doi:10.1105/tpc.16.00186.
- Ishikawa T, Fang L, Rennie EA, Sechet J, Yan J, Jing B, Moore W, Cahoon EB, Scheller HV, Kawai-Yamada M, et al. GLUCOSAMINE INOSITOLPHOSPHORYLCERAMIDTRANSFERASE1 (GINT1) is a GlcNAc-containing glycosylinositol phosphorylceramide glycosyltransferase. Plant Physiol. 2018;177(3):938–952. doi:10.1104/pp.18.00396.
- Msanne J, Chen M, Luttgeharm KD, Bradley AM, Mays ES, Paper JM, Paper JM, Boyle DL, Cahoon RE, Kathrin Schrick, Cahoon EB. Glucosylceramides are critical for cell-type differentiation and organogenesis, but not for cell viability in Arabidopsis. Plant J. 2015;84(1):188–201. doi:10.1111/tpj.13000.
- Dai GY, Yin J, Li KE, Chen DK, Liu Z, Bi FC, Rong C, Yao N. The Arabidopsis AtGCD3 protein is a glucosylceramidase that preferentially hydrolyzes long-acyl-chain glucosylceramides. J Biol Chem. 2020;295(3):717–728. doi:10.1074/jbc.RA119.011274.
- Chen LY, Shi DQ, Zhang WJ, Tang ZS, Liu J, Yang WC. The Arabidopsis alkaline ceramidase TOD1 is a key turgor pressure regulator in plant cells. Nat Commun. 2015;6(1):1–10. doi:10.1038/ncomms7030.
- Li J, Bi FC, Yin J, Wu JX, Rong C, Wu JL, Yao N. An Arabidopsis neutral ceramidase mutant ncer1 accumulates hydroxyceramides and is sensitive to oxidative stress. Front Plant Sci. 2015;6(JUNE):1–8. doi:10.3389/fpls.2015.00460.
- Pata MO, Wu BX, Bielawski J, Xiong TC, Hannun YA, Ng CKY. Molecular cloning and characterization of OsCDase, a ceramidase enzyme from rice. Plant J. 2008;55(6):1000–1009. doi:10.1111/j.1365-313X.2008.03569.x.
- Wu JX, Li J, Liu Z, Yin J, Chang ZY, Rong C, Wu JL, Bi FC, Yao N. The Arabidopsis ceramidase AtACER functions in disease resistance and salt tolerance. Plant J. 2015;81(5):767–780. doi:10.1111/tpj.12769.
- Guo L, Mishra G, Taylor K, Wang X. Phosphatidic acid binds and stimulates arabidopsis sphingosine kinases. J Biol Chem. 2011;286(15):13336–13345. doi:10.1074/jbc.M110.190892.
- Imai H, Nishiura H. Phosphorylation of Sphingoid Long-chain Bases in Arabidopsis: functional Characterization and Expression of the First Sphingoid Long-chain Base Kinase Gene in Plants. Plant Cell Physiol. 2005;46(2):375–380. doi:10.1093/pcp/pci023.
- Marion J, Bach L, Bellec Y, Meyer C, Gissot L, Faure J-D. Systematic analysis of protein subcellular localization and interaction using high-throughput transient transformation of Arabidopsis seedlings. Plant J. 2008;56(1):169–179. doi:10.1111/j.1365-313X.2008.03596.x.
- Chen M, Han G, Dietrich CR, Dunn TM, Cahoon EB. The essential nature of sphingolipids in plants as revealed by the functional identification and characterization of the Arabidopsis LCB1 subunit of serine palmitoyltransferase. Plant Cell. 2006;18(12):3576–3593. doi:10.1105/tpc.105.040774.
- Ng CKY, Carr K, McAinsh MR, Powell B, Hetherington AM. Drought-induced guard cell signal transduction involves sphingosine-1-phosphate. Nature. 2001;410(6828):596–599. doi:10.1038/35069092.
- Coursol S, Fan LM, Stunff HL, Splegel S, Gilroy S, Assman SM. Sphingolipid signalling in Arabidopsis guard cells involves heterotrimeric G proteins. Nature. 2003;423(6940):651–654. doi:10.1038/nature01643.
- Verhoek B, Linscheid M, Wrage K, Heinz E. Lipids and enzymatic activities in vacuolar membranes isolated via protoplasts from oat primary leaves. Zeitschrift Fur Naturforschung Sect C J Biosci. 1983;38(9–10):770–777. doi:10.1515/znc-1983-9-1018.
- Warnecke D, Heinz E. Recently discovered functions of glucosylceramides in plants and fungi. Cell Mol Life Sci. 2003;60:919–941. doi:10.1007/s00018-003-2243-4.
- Baxter I, Tchieu J, Sussman MR, Boutry M, Palmgren MG, Gribskov M, Axelsen KB. Genomic comparison of P-type ATPase ion pumps in Arabidopsis and rice. Plant Physiol. 2003;132(2):618–628. doi:10.1104/pp.103.021923.
- Nintemann SJ, Palmgren M, López-Marqués RL. Catch you on the flip side: a critical review of flippase mutant phenotypes. Trends Plant Sci. 2019;24:468–478. doi:10.1016/j.tplants.2019.02.002.
- Gomès E, Jakobsen MK, Axelsen KB, Geisler M, Palmgren MG. Chilling tolerance in arabidopsis involves ALA1, a member of a new family of putative aminophospholipid translocases. Plant Cell. 2000;12. doi:10.1105/tpc.12.12.2441.
- López-Marqués RL, Poulsen LR, Hanisch S, Meffert K, Buch-Pedersen MJ, Jakobsen MK, Pomorski TG, Palmgren MG. Intracellular targeting signals and lipid specificity determinants of the ALA/ALIS P4-ATPase complex reside in the catalytic ALA alpha-subunit. Mol Biol Cell. 2010;21(5):791–801. doi:10.1091/mbc.e09-08-0656.
- Poulsen LR, López-Marqués RL, Pedas PR, McDowell SC, Brown E, Kunze R, Palmgren M. A phospholipid uptake system in the model plant Arabidopsis thaliana. Nat Commun. 2015;6(1):7649. doi:10.1038/ncomms8649.
- Guo Z, Lu J, Wang X, Zhan B, Li W, Ding S-W. Lipid flippases promote antiviral silencing and the biogenesis of viral and host siRNAs in Arabidopsis. Proc Natl Acad Sci. 2017;114(6):1377–1382. doi:10.1073/pnas.1614204114.
- Zhu B, Gao H, Xu G, Wu D, Song S, Jiang H, Xie D. Arabidopsis ALA1 and ALA2 mediate RNAi-based antiviral immunity. Front Plant Sci. 2017;8:422. doi:10.3389/fpls.2017.00422.
- McDowell SC, López-Marqués RL, Poulsen LR, Palmgren MG, Harper JF. Loss of the arabidopsis thaliana P4-ATPase ALA3 reduces adaptability to temperature stresses and impairs vegetative, pollen, and ovule development. PLoS One. 2013;8(5):e62577. doi:10.1371/journal.pone.0062577.
- Underwood W, Ryan A, Somerville SC. An arabidopsis lipid flippase is required for timely recruitment of defenses to the host-pathogen interface at the plant cell surface. Mol Plant. 2017;10(6):805–820. doi:10.1016/j.molp.2017.04.003.
- Zhang X, Oppenheimer DG. IRREGULAR TRICHOME BRANCH 2 (ITB2) encodes a putative aminophospholipid translocase that regulates trichome branch elongation in Arabidopsis. Plant J. 2009;60(2):195–206. doi:10.1111/j.1365-313X.2009.03954.x.
- McDowell SC, López-Marqués RL, Cohen T, Brown E, Rosenberg A, Palmgren MG, Harper JF. Loss of the Arabidopsis thaliana P4-ATPases ALA6 and ALA7 impairs pollen fitness and alters the pollen tube plasma membrane. Front Plant Sci. 2015;6:197. doi:10.3389/fpls.2015.00197.
- Botella C, Sautron E, Boudiere L, Michaud M, Dubots E, Yamaryo-Botté Y, Jouhet J. ALA10, a phospholipid flippase, controls FAD2/FAD3 desaturation of phosphatidylcholine in the ER and affects chloroplast lipid composition in arabidopsis thaliana. Plant Physiol. 2016;170(3):1300–1314. doi:10.1104/pp.15.01557.
- Zhang X, Adamowski M, Marhava P, Tan S, Zhang Y, Rodriguez L, Zwiewka M, Pukyšová V, Sánchez AS, Raxwal VK, et al. Arabidopsis flippases cooperate with ARF GTPase exchange factors to regulate the trafficking and polarity of PIN Auxin transporters. Plant Cell. 2020;32(5):1644. doi:10.1105/tpc.19.00869.
- Pinneh EC, Mina JG, Stark MJR, Lindell SD, Luemmen P, Knight MR, steel PG, Denny PW. The identification of small molecule inhibitors of the plant inositol phosphorylceramide synthase which demonstrate herbicidal activity. Sci Rep. 2019;9(1):1–8. doi:10.1038/s41598-019-44544-1.
- Körschen HG, Yildiz Y, Raju DN, Schonauer S, Bönigk W, Jansen V, Kremmer E, Kaupp UB, Wachten D. The non-lysosomal β-glucosidase GBA2 is a non-integral membrane-associated protein at the endoplasmic reticulum (ER) and Golgi. J Biol Chem. 2013;288(5):3381–3393. doi:10.1074/jbc.M112.414714.
- Lynch DV, Phinney AJ. The transbilayer distribution of glucosylceramide in plant plasma membrane. In: Plant lipid metabolism. 1995; p. 239–241. doi:10.1007/978-94-015-8394-7_66.
- Tjellström H, Hellgren LI, Wieslander Å, Sandelius AS. Lipid asymmetry in plant plasma membranes: phosphate deficiency‐induced phospholipid replacement is restricted to the cytosolic leaflet. Faseb J. 2010;24(4):1128–1138. doi:10.1096/fj.09-139410.
- Lynch DV, Steponkus PL. Plasma membrane lipid alterations associated with cold acclimation of winter rye seedlings (Secale cereale L. cv Puma)1. Plant Physiol. 1987;83(4):761–767. doi:10.1104/pp.83.4.761.
- Sperling P, Franke S, Lüthje S, Heinz E. Are glucocerebrosides the predominant sphingolipids in plant plasma membranes? Plant Physiol Biochem. 2005;43(12):1031–1038. doi:10.1016/j.plaphy.2005.10.004.
- Roland BP, Naito T, Best JT, Arnaiz-Yépez C, Takatsu H, Yu RJ, Shin HW, Graham TR. Yeast and human P4-ATPases transport glycosphingolipids using conserved structural motifs. J Biol Chem. 2019;294(6):1794–1806. doi:10.1074/jbc.RA118.005876.
- Zienkiewicz A, Gömann J, König S, Herrfurth C, Liu Y, Meldau D, Feussner I. Disruption of Arabidopsis neutral ceramidases 1 and 2 results in specific sphingolipid imbalances triggering different phytohormone‐dependent plant cell death programmes. New Phytol. 2020;226(1):170–188. doi:10.1111/nph.16336.
- Guo L, Mishra G, Markham JE, Li M, Tawfall A, Welti R, Wang X. Connections between sphingosine kinase and phospholipase D in the abscisic acid signaling pathway in Arabidopsis. J Biol Chem. 2012;287(11):8286–8296. doi:10.1074/jbc.M111.274274.
- Worrall D, Liang YK, Alvarez S, Holroyd GH, Spiegel S, Panagopulos M, Gray JE, Hetherington AM. Involvement of sphingosine kinase in plant cell signalling. Plant J. 2008;56(1):64–72. doi:10.1111/j.1365-313X.2008.03579.x.
- Hicks AA, Pramstaller PP, Johansson Å, Vitart V, Rudan I, Ugocsai P, Aulchenko Y ,Franklin CS, Liebisch G, Erdmann J, et al. Genetic determinants of circulating sphingolipid concentrations in European populations. PLoS Genet. 2009;5(10):e1000672. doi:10.1371/journal.pgen.1000672.
- Watanabe T, Tani M, Ishibashi Y, Endo I, Okino N, Ito M. Ergosteryl-β-glucosidase (Egh1) involved in sterylglucoside catabolism and vacuole formation in Saccharomyces cerevisiae. Glycobiology. 2015;25(10):1079–1089. doi:10.1093/glycob/cwv045.
- Dickson RC, Lester RL. Yeast sphingolipids. Biochimica Et Biophysica Acta Gen Subj. 1999;1426:347–357. doi:10.1016/S0304-4165(98)00135-4.
- Saito K, Takakuwa N, Ohnishi M, Oda Y. Presence of glucosylceramide in yeast and its relation to alkali tolerance of yeast. Appl Microbiol Biotechnol. 2006;71(4):515–521. doi:10.1007/s00253-005-0187-3.
- Hu G, Caza M, Bakkeren E, Kretschmer M, Bairwa G, Reiner E, Kronstad J. A P4-ATPase subunit of the Cdc50 family plays a role in iron acquisition and virulence in Cryptococcus neoformans. Cell Microbiol. 2017;19:6. doi:10.1111/cmi.12718.
- Ishibashi Y, Ikeda K, Sakaguchi K, Okino N, Taguchi R, Ito M. Quality control of fungus-specific glucosylceramide in Cryptococcus neoformans by endoglycoceramidase-related protein 1 (EGCrP1). J Biol Chem. 2012;287(1):368–381. doi:10.1074/jbc.M111.311340.