ABSTRACT
Environmental factors such as photoperiod, temperature, phytohormones, sugars, and soil nutrients can affect the development of axillary meristems and emergence of shoot branches in plants. We investigated how an extended period of cold exposure to Arabidopsis plants before and after inflorescence meristem differentiation would affect plant growth and shoot branching. The number of rosette leaves and shoot branches increased when wild type (WT) juvenile seedlings, but not adult plants, were subjected to a prolonged cold exposure (10/7°C day/night cycle). As the duration of cold exposure to WT juvenile seedlings increased, so too did the rosette area, number of leaves, and rosette branches revealing an extended period of vegetative growth. The prolonged cold treatment also increased the primary inflorescence stem height and number of cauline branches in WT plants revealing a delay in reproductive development that could be altered by early (set domain group 8; sdg8) and late (methyltransferase 1; met1) flowering mutants. The axillary buds/leaf and rosette branches/leaf ratios declined significantly in WT, yet were enhanced in the loss-of-function of carotenoid cleavage dioxygenase 7 (ccd7) and teosinte branched 1 (brc1) hyper-branched mutants. This indicated that axillary meristem differentiation continued during the cold exposure, which did not directly impact axillary bud formation or shoot branching. We conclude that a prolonged cold exposure to juvenile seedlings prior to inflorescence meristem development extended vegetative growth and delayed the reproductive phase to allow additional leaf primordia and axillary meristems to differentiate that enhanced the number of shoot branches in Arabidopsis.
Introduction
Shoot branching is a highly plastic developmental trait of plants that determines plant architecture, foliar coverage, photosynthetic area, and fruit and seed production. An intricate interaction between the environment (e.g. light, temperature, and nutrients), phytohormones, and sugars control the development of axillary meristems, shoot bud formation, and branching architecture during plant development.Citation1,Citation2 The shoot tip of Arabidopsis juvenile seedlings develops leaf primordia in the periphery of the shoot apical meristem (SAM). Development of an axillary meristem within the axil of leaf initiates the formation of an axillary bud.Citation3,Citation4 The differentiation of the inflorescence meristem (IM) during the transition from vegetative to reproductive development ceases the formation of axillary meristems.Citation5 Axillary buds do not develop during vegetative development in Arabidopsis, except when plants are grown under a shorter photoperiod. Under a longer photoperiod, axillary buds are formed after flowering.Citation3,Citation6,Citation7
Environmental conditions show pronounced impacts on the shoot branching habit of plants. In Arabidopsis, a long photoperiod promotes early flowering and a rapid life cycle in comparison to plants grown under a short photoperiod.Citation8 The vegetative phase of a long-day grown plant ends 10–12 days after germination after the SAM transitions into an IM.Citation4 A shorter photoperiod delays IM development and extends the vegetative phase leading to an increase in the number of rosette leaves, branches, and plant biomass.Citation6,Citation8-Citation10 The bolting transition and cauline leaf numbers can be an indicator of a reproductive phase change in that an extended cauline zone correlates with an increased number of days to flowering.Citation11,Citation12 A change in temperature can alter the vegetative to reproductive transition. A warmer temperature can induce early flowering and shorten the life span in Arabidopsis.Citation13,Citation14 The winter-annual accessions of Arabidopsis that contain FRIGIDA require juvenile seedlings (or seeds) to be exposed to a prolonged period of cold exposure (30–40 days), a process called vernalization that promotes early flowering.Citation15 The vernalization-induced induction of flowering in winter-annual Arabidopsis is under epigenetic control.Citation16 Whether a duration of cold exposure (e.g. one or more weeks) to juvenile and adult plants can affect their shoot branching habit in Arabidopsis remained unclear.
The differential regulation of the biosynthesis of carotenoid-derived apocarotenoids affects shoot branching in Arabidopsis. The β-carotene derived apocarotenoid strigolactone (SL) is a phytohormone that inhibits lateral shoot bud outgrowth to control shoot branching in plants.Citation17,Citation18 The CAROTENOID ISOMERASE (CRTISO) catalyses the isomerization of 7,9,9′,7′-tetra-cis-lycopene to all-trans-lycopene, that undergoes cyclization to generate β-carotene.Citation19 CAROTENOID CLEAVAGE DIOXYGENASE (CCD) enzymes (CCD7 and CCD8) cleave β-carotene in the early steps of SL biosynthesis.Citation20 The loss-of-function in CCD7 (ccd7 or more axillary branching 3; max3) or CCD8 (ccd8 or max4) blocks SL biosynthesis leading to an increase in the number of rosette shoot branches.Citation17,Citation21 crtiso mutants also show an increased number of shoot branches in rice and Arabidopsis, presumably due to a perturbation in SL biosynthesis.Citation22,Citation23
The TEOSINTE BRANCHED 1 (BRC1) encodes a transcription factor proposed to regulate shoot architecture by integrating phytohormones, developmental and environmental signals that suppress axillary bud outgrowth.Citation24-Citation27 BRC1 suppresses auxin efflux and auxin accumulation in axillary buds; inhibiting lateral bud outgrowth such as in cucumber (Cucumis sativus L.).Citation28 The interaction of BRC1 with FLOWERING LOCUS T (FT) delays the floral transition in axillary meristems and extends the vegetative phase of development.Citation29 BRC1 acts as a signaling hub downstream of the strigolactone signaling pathway to arrest bud outgrowth.Citation24
Cold exposure can induce selective modifications in histone methylation patterns; subsequently, altering the gene expression landscapes and phenotypes in different plants.Citation30-Citation32 A prolonged cold treatment (e.g. vernalization) of juvenile Arabidopsis seedlings induces epigenetic silencing of the transcriptional repressor of flowering time, FLOWERING LOCUS C (FLC), and promotes early flowering under warmer growth conditions.Citation16,Citation33 The loss-of-function in SET DOMAIN GROUP8 (SDG8) mutant (sdg8 or early flowering in short days; efs, or chloroplast and carotenoid regulation 1; ccr1) has a shorter juvenile phase and flowers early independent of vernalization by repressing FLC expression.Citation23,Citation34 SDG8 promotes histone-3-lysine-4 (H3K4) and lysine-36 (H3K36) trimethylation surrounding the ATG start codon of FLC and has other pleiotropic effects on plant growth and metabolism.Citation35 For example, SDG8 promotes CRTISO gene expression and regulates carotenoid composition in a tissue-specific manner.Citation36 sdg8/ccr1 mutant alleles show an enhanced number of rosette and cauline branches despite having a shorter juvenile phase that causes early flowering in Arabidopsis Col-0.Citation23,Citation37
Epigenetic modifications such as DNA methylation at CG, CHG, and CHH (H is A, C, or T) sequences, can alter the plant’s response to a prolonged cold exposure.Citation32 In trees such as hybrid poplar (Populus tremula × alba), chilling-dependent induction of DEMETER-LIKE 10 (PtaDML10) activated progressive demethylation of genomic DNA and shoot bud outgrowth, revealing that DNA methylation is required to mediate axillary bud differentiation in response to winter chilling.Citation38 In Arabidopsis, DNA METHYLTRANSFERASE 1 (MET1) functions to establish and maintain CG context-dependent DNA methylation.Citation39 MET1 represses shoot regeneration by inhibiting the homeobox transcription factor WUSCHEL (WUS).Citation40 WUS is expressed in the organizing center of the SAM, axillary meristems, floral meristems, and adventitious shoot meristems where it maintains an undifferentiated stem cell niche.Citation41-Citation43 MET1-dependent methylation of the WUS promoter represses WUS expression, whereas demethylation activates WUS expression and induces axillary bud differentiation.Citation40 The stronger met1-1 mutant allele shows a delay in Arabidopsis (Col-0) flowering time and juvenile-to-adult phase transition that is accompanied by the production of additional rosette leaves and aerial cauline leaves prior to elongation of the primary inflorescence stem.Citation44,Citation45
Here we reveal how shoot branching can be enhanced by exposing juvenile (vegetative), but not adult (reproductive) Arabidopsis plants to a prolonged duration of cold. Arabidopsis mutants that display hyper-branching (crtiso; ccd7 and brc1), early flowering (sdg8), and extended juvenility (met1) were used to explore the impacts of prolonged cold exposure on branching phenotypes. All genotypes showed an enhanced number of rosette leaves and rosette branches. Our findings reveal that a prolonged cold exposure to juvenile seedlings extended vegetative growth and delayed the reproductive phase thereby enhancing shoot branching in Arabidopsis.
Materials and methods
Germplasm and plant growth conditions
The Arabidopsis thaliana (ecotype Col-0) and mutants crtiso (ccr2.1),Citation46 sdg8 (ccr1.1),Citation23 ccd7 (max3-11),Citation23 brc1-1 (SALK_091920 C), and met1-7 (SALK_076522) were used this study. Mutants were confirmed by carotenoid profiling, phenotyping, and/or genotyping. Arabidopsis plants were grown in a Climatron Star700 growth cabinets (Thermoline Scientific, Australia). Seeds were sown onto soil (Debco Seed Raising and Superior germinating soil mixture supplemented with 3% Osmocote) and stratified for 3 days at 4°C in the dark. Seeds were germinated and plants grown under normal growth conditions (16/8 h light/dark photoperiod; 130–150 µmolm−Citation2 sec−Citation1 cool fluorescent lighting; 22/18°C day/night temperatures) unless stated otherwise.
Cold treatments and plant phenotyping
Arabidopsis plants; 7, 14, and 21 days after stratification (DAS), were subject to a cold treatment (10/7°C day/night cycle, 16/8 h light/dark photoperiod; 130–150 µmolm−2sec−1 cool fluorescent lighting) for a period of between 4 weeks depending upon the experiment (). After the period of cold treatment, plants were grown under normal growth conditions until reproductive maturity. Juvenile Arabidopsis seedlings (1-week old; 7DAS) were exposed to the cold for 1–5 weeks, after which they were transferred back to normal growth conditions (22/18°C day/night cycle) and grown to reproductive maturity ().
Figure 1. Schematic representation of cold treatments and phenotype characterization of plants.
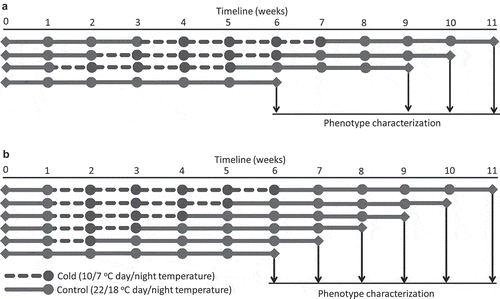
The rosette area, number of rosette leaves, and rosette biomass were measured when the primary inflorescence stem emerged (<5 mm). Mutants included in this study had different flowering times and therefore inflorescence stem height (to the first silique), the number of shoot branches, and shoot biomass for each genotype was determined when 3–5 basal siliques of the primary floral stem started yellowing and/or had opened to disperse seeds. The rosette area was measured by analyzing rosette pictures using ImageJ, an open-source Java-based image processing computer program.Citation47 The dry weight of rosettes was determined by drying for 48 h at 40°C. Each experiment was repeated at least twice to minimize the experimental variations assuring reproducibility of the data.
Results
Prolonged cold treatment to juvenile seedlings increases the number of rosette branches
Arabidopsis wild type (WT) and crtiso mutant plants were grown at normal temperatures (22/18°C day/night cycle) and exposed to 4 weeks of prolonged cold (10/7°C day/night cycle) at different developmental stages (7, 14, and 21 DAS); after which plants were returned to normal growth temperatures to quantify shoot branching (). Exposure of both WT and crtiso mutant juvenile seedlings (7 DAS) to 4 weeks of cold significantly increased the number of rosette and cauline branches, while branching was not significantly altered in plants transferred to the cold on 14 or 21 DAS (-b). The number of rosette branches were two-fold higher in crtiso compared to WT (), keeping consistent with a previous report showing that crtiso mutants can develop additional rosette branches under certain environmental conditions.Citation23 Therefore, a prolonged period of cold exposure to juvenile seedlings prior to IM development (before 10–12 DAS) can cause a greater number of rosette shoot branches to develop in Arabidopsis.
Figure 2. Prolonged cold exposure to juvenile seedlings enhances plant growth traits and shoot branching.
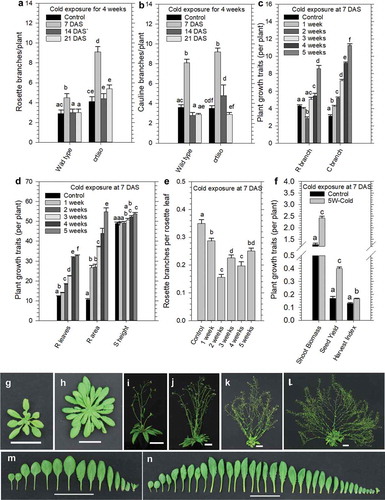
Next, we examined what effect extending the duration of cold exposure might have on various plant growth traits. WT juvenile seedlings were grown under normal temperature conditions (22/18°C day/night cycle) for 7 DAS and transferred to the cold (10/7°C day/night cycle) for 0, 1, 2, 3, 4, and 5 weeks; after which they were returned to normal temperatures (22/18°C day/night cycle) (). The number of rosette leaves, rosette area, rosette branches, cauline branches and primary inflorescence stem height increased with a longer duration of cold exposure (-d). The shoot biomass and seed yield were also significantly enhanced when juvenile seedlings were exposed to 5-weeks of cold treatment ().
The increased number of cauline branches and primary inflorescence stem (primary bolt) height indicated a possible delay in flowering time (-d,i-l). Plants exposed to 5-weeks of cold exposure showed a fivefold larger rosette area (-h) and 2.3- to 2-fold increase in the number of rosette leaves (,m-n) and rosette shoot branches (,i-l), respectively. Intriguingly, the number of rosette branches to leaf ratio significantly declined in cold exposed plants, revealing either a reduction in axillary meristem formation or increase leaf primordia development (). Taken together, a prolonged period of cold exposure to juvenile seedlings for 5 weeks can significantly enhance vegetative and reproductive growth traits, leading to a branchy phenotype in Arabidopsis.
Prolonged cold enhances rosette shoot branching in hyper-branched mutants
We next tested if the prolonged cold exposure affected axillary meristem formation using mutants impaired in strigolactone biosynthesis (ccd7/max3) and axillary bud development (brc1). Under normal growth conditions (control), the mutants showed a similar number of rosette leaves and cauline branches, and only a slight shorter primary inflorescence stem in comparison to WT (,d-e). ccd7 and brc1 produced few rosette buds (<5 mm) and showed a substantially higher number of rosette branches (>5 mm) relative to WT (-c). In response to the prolonged cold exposure, there was a significant increase in the number of rosette leaves, rosette buds, rosette branches, cauline branches, and primary inflorescence stem height (vegetative part below the first silique) in all germplasm (-e). The increase in shoot branching was highly pronounced in ccd7 and brc1 hyper-branched mutants as expected (). Therefore, the prolonged cold treatment of juvenile seedlings most likely extended vegetative development providing time to further increase the development of additional rosette branches in ccd7 and brc1 hyper-branched mutants.
Figure 3. Prolonged cold exposure of juvenile plants enhanced growth and branching traits in mutants displaying a hyper-branched phenotype and stem cell maintenance of the shoot apical meristem. Juvenile wild type (WT) and mutants (carotenoid cleavage dioxygenase 7; ccd7, branched 1; brc1, set domain group 8; sdg8, and DNA methyl transferase 1; met1 were grown for 7 DAS under normal conditions and then transferred to the cold (10/7°C day/night cycle) for 5-weeks, after which they were returned to normal growth conditions until reproductive maturity. Growth traits measured include; a) number of rosette leaves, b) number of emerged rosette shoot buds (<5 mm), c) number of rosette branches (>5 mm), d) number of cauline branches, e) primary inflorescence stem height to the first silique, F) number of shoot buds per rosette leaf, g) number of branches per rosette leaf, H) number of cauline branches per cm stem height. Mean values display standard error of the means (n = 15). Denoted letters indicate statistical variation (p < .05) in growth attributes within treatment groups and across genotypes as determined by a Two-Way ANOVA using a Holm-Sidak post hoc multiple comparison.
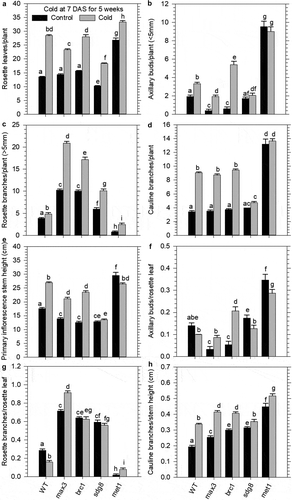
We next examined if prolonged cold affected axillary meristem formation. The significant increase in the ratio of cauline branches relative to the height of the primary inflorescence stem is consistent with the increased number of rosette leaves reflecting delayed flowering (). The ratio of the number of axillary buds relative to the rosette leaves remained unchanged in WT, yet increased in ccd7, and was substantially higher in brc1 following cold treatment, revealing axillary meristem formation continued under prolonged cold treatment (). The ratio of the number of rosette branches to leaves was decreased in WT, remained unchanged in brc1 and was considerably higher in ccd7 (). The rosette branch to leaf ratio of approximately one in ccd7 reflected an equal number of leaves and rosette branches developed during the cold treatment. Therefore, the prolonged cold does not impact axillary meristem formation, since the hyper-branched mutants ccd7 and brc1 were able to develop additional axillary buds and rosette branches.
Prolonged cold enhances the number of shoot branches by extending vegetative growth
sdg8 and met1 mutants flower early and late, respectively.Citation23,Citation44 Under normal growth conditions, the number of rosette leaves as well as the primary inflorescence stem height of sdg8 and met1 mutant plants significantly decreased and increased respectively, compared to WT (,e). The substantially higher inflorescence stem height allowed additional cauline branches to develop in met1 (). These phenotypes displayed by sdg8 and met1 were consistent with mutants that flower early and late, respectively. Exposure of sdg8 and met1 juvenile seedlings to prolonged cold significantly increased the number of rosette leaves, albeit to a slightly lesser extent compared to WT (). Therefore, SDG8 and MET1 activities were not required for the formation of additional leaf primordia during the extended vegetative phase. In contrast, the primary inflorescence stem height and cauline branch number in sdg8 and met1 were not enhanced by the cold treatment (-e), revealing that SDG8 and MET1 activities can affect the spatial organization of the cauline leaf zone between the rosette and the inflorescence.
sdg8 and met1 mutants developed additional and fewer rosette branches, respectively, to WT under normal growth conditions (). Compared to WT, sdg8 mutants displayed a similar axillary bud/leaf ratio and higher rosette branch/leaf ratio, exemplifying that sdg8 develops a hyper-branched phenotype under normal growth conditions. The substantially higher number of axillary buds and relatively low rosette branch/leaf ratio in met1 revealed that MET1 may function in controlling dormancy or outgrowth of the axillary bud into a rosette branch (). Exposure of sdg8 and met1 juvenile seedlings to prolonged cold did not change the number of axillary buds, and like WT, there was a significant decrease in the ratio of axillary buds relative to the number of rosette leaves (,f). Prolonged cold exposure of sdg8 and met1 juvenile seedlings significantly increased the number of rosette branches, and yet did not reduce the rosette branch/leaf ratio like that observed in WT (,g). These findings revealed that the cold-induced increase in the number of rosette branches could be a consequence of additional leaf primordia developing under the extended period of vegetative development.
Discussion
Prolonged cold treatment of juvenile seedlings extends vegetative development
Shoot branching contributes to foliage cover, leaf photosynthetic area, shoot biomass, and fruit and seed production in plants.Citation48 A shorter photoperiod during the winter season can enhance the number of rosette leaves and shoot branches in plants.Citation10 How a prolonged period of cold exposure to plants growing under a longer photoperiod impacts shoot branching in Arabidopsis remained unclear. We demonstrate that exposing juvenile WT and crtiso mutant seedlings (7 DAS) to 4 weeks of prolonged cold enhanced the number of rosette and cauline branches. The significantly higher number of rosette branches displayed by crtiso mutants subjected to cold exposure is consistent with a previous report describing that crtiso mutant alleles can generate additional branches depending upon the growth environment.Citation23 The adult WT and crtiso mutant plants (2-weeks or older in age) were unresponsive to the cold treatment and displayed a similar branching habit to the untreated control plants grown under normal growth temperatures () revealing an age-dependent effect of prolonged cold exposure on shoot branching. A longer duration of cold exposure to WT correlated with an increased number of rosette leaves, rosette area, and enhanced branching habit, that after 5 weeks collectively enhanced plant biomass, seed yield, and the harvest index (). These phenotypes were consistent to when Arabidopsis plants are grown under a shorter photoperiod, revealing that the prolonged cold exposure may have extended vegetative growth ().
Figure 4. Model showing how exposure of juvenile seedlings to prolonged cold enhances shoot branching in Arabidopsis. DNA METHYLTRANSFERASE 1 (MET1) negatively regulates WUSCHEL (WUS), which maintains the stem cell niche of the shoot apical meristem (SAM) in an undifferentiated state. The met1 mutant impairs CG context-dependent DNA methylation, enhancing WUS expression in the SAM that enhances the formation of axillary buds.Citation40,Citation49 Leaf primordia and axillary bud differentiation in the axillary meristem (AM) is programmed during juvenile seedling development (<12 days after stratification; DAS), after which the SAM transitions into a inflorescence meristem (IM; >14 DAS).Citation4 Upon flowering the primary inflorescence stem (FS; floral bolt) emerges from the IM. A cauline branch (CB) and leaf emerges at nodes along the primary inflorescence stem. SET DOMAIN GROUP 8 (SDG8) maintains CAROTENOID ISOMERASE (CRTISO) gene expression and accumulation of β-carotene, a substrate required for biosynthesis of strigolactone (SL).Citation23 SL regulates BRANCHED 1 (BRC1), which represses axillary bud outgrowth, that would otherwise emerge into a rosette branch (RB).Citation24,Citation50 sdg8 (ccr1) and crtiso (ccr2) mutants may impair SL biosynthesis,Citation51 while the loss-of-function in CAROTENOID CLEAVAGE DIOXYGENASE 7 mutant (ccd7) prevents SL biosynthesis.Citation21 Negative and positive regulation are indicated by solid red and green arrowed lines, respectively. Black arrowed lines indicate carotenoid biosynthesis steps in pathway. The red dashed lines indicate a presumed function of SDG8 in controlling histone methylation in the SAM.
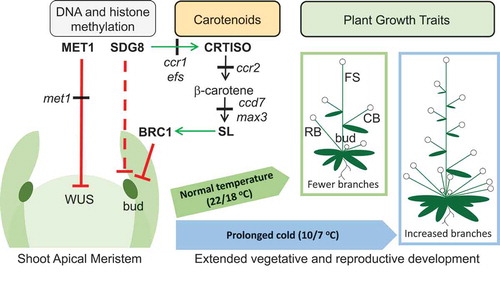
In Arabidopsis, the transition from vegetative to reproductive development is affected by day length, a critical checkpoint in determining the number of rosette leaves and branching habit.Citation10,Citation52,Citation53 Transcriptomic analysis of temporal changes in the SAM from long-day grown Arabidopsis plants demonstrated that the vegetative to reproductive transition occurs between 10 and 12 days after germination.Citation4 Therefore, we reason that moving adult plants to the cold after differentiation of the IM (>14 DAS) would be restricted from differentiating additional leaf primordia under the long photoperiod due to the reproductive transition (). A shorter photoperiod can extend vegetative growth and delay flowering to allow the differentiation of additional leaf primordia, rosette leaves, and shoot branches.Citation6,Citation10 We conclude that the prolonged cold treatment of juvenile seedlings extended vegetative growth and delayed the transition of the SAM into an IM, thereby increasing the number of leaves, axillary meristems, and rosette branches per plant ().
Prolonged cold delayed the reproductive phase to enhance the cauline zone
The SAM in juvenile seedlings would transition into an IM during the 5 weeks of prolonged cold exposure to extend the reproductive phase. Arabidopsis Col-0 will generally, flower within 3–4 weeks after sowing, with 6–8 rosette leaves and 2–3 cauline leaves, when normal growth conditions and a long-day photoperiod are provided. The prolonged cold caused a substantial increase in cauline leaf number and a taller primary inflorescence stem height (). This was indicative of an enhanced cauline leaf zone between the rosette and true inflorescence and hence delayed flowering.Citation11,Citation12 This prompted us to explore if mutants that disrupt flowering time could impair the cold-induced increase in the cauline zone. DNA and histone methylation can affect the timing of flowering in Arabidopsis. MET1 controls context-dependent CG DNA methylation and the loss-of-function met1 mutant alters shoot meristem identity to delay the reproductive phase in Arabidopsis (Figure 4).Citation44,Citation45 Consistent with these reports, met1 plants flowered late producing a greater number of rosette leaves and cauline branches as well as a taller primary inflorescence stem height causing a higher ratio in the number of cauline branches/stem height compared to WT.
SDG8 maintains permissive histone-3-lysine-4/36 trimethylation surrounding key genes associated with the developmental programming of the SAM (), and sdg8 mutant plants transition sooner to the reproductive phase.Citation23,Citation35,Citation54,Citation55 Consistent with these reports, sdg8 (Col-O ecotype) mutants flowered early generating fewer rosette leaves and a shorter inflorescence stem height per plant in comparison to WT. sdg8 mutant plants generated a slightly higher number of cauline branches, due to pleiotropic effects associated with the reduction in H3K4 tri-methylation at specific genomic lociCitation56 and/or the global reduction in H3K36 tri-methylationCitation54 that causes the formation of two cauline branches per node of the primary inflorescence stem.Citation23 As such the ratio of cauline branches to leaves was higher in sdg8 relative to WT when grown under normal growth temperatures.
The met1 and sdg8 inflorescence phenotypes are consistent with delayed and early flowering, respectively. Interestingly, both met1 and sdg8 impaired the cold-induced increase in primary inflorescence stem height and cauline branch number per plant that was characteristically observed in WT (). This was further exemplified by a modest increase in the cauline branches/inflorescence stem height ratio compared to WT and hyper-branched mutants. However, met1 and sdg8 like WT plants produced additional rosette leaves in response to prolonged cold. Therefore, the increased primary inflorescence stem height and cauline branch number triggered by the prolonged cold treatment of WT Arabidopsis seedlings could be due to an extended reproductive phase that delayed flowering and increased the cauline leaf zone between the rosette and the primary inflorescence ().
Axillary meristem differentiation and bud outgrowth continue under prolonged cold
Prolonged cold exposure of juvenile seedlings increased the number of axillary buds and shoot branches per plant, yet also increased the number of rosette leaves and hence leaf primoridia differentiation. Cold exposure to plants during vegetative growth can increase the level of sucrose to facilitate stress acclimation.Citation57–Citation59 Soluble sugars such as sucrose and trehalose-6 phosphate can regulate axillary bud outgrowth.Citation17,Citation60,Citation61 Increased sucrose suppresses the auxin-induced strigolactone pathway to trigger bud outgrowth.Citation62 To further investigate the effects of a prolonged cold exposure on axillary meristem formation we assessed ratios in the number of axillary buds/leaf as well as rosette branches/leaf. Contrary to the effect of prolonged cold increasing the number of rosette branches, a slightly lower ratio in the number of axillary buds/leaf and rosette branches/leaf indicated that either the differentiation of axillary meristems and/or leaf primordia were lower and/or greater, respectively ().
We first considered if the cold exposure to juvenile Arabidopsis plants affected axillary meristem differentiation or bud outgrowth. The ccd7 and brc1 hyper-branched mutants show enhanced axillary bud outgrowth, in concert with ccd7 being reported to have a twofold higher rosette branch/leaf ratio in Arabidopsis.Citation24,Citation63 Under normal growth conditions, the number of rosette branches/leaf ratio was greater than two-fold higher in ccd7 and brc1 keeping consistent with previous reports.Citation63,Citation64 This was further supported by a lower ratio in the number of axillary buds/leaf, indicating that axillary bud outgrowth was considerably higher in the hyper-branched mutants. The prolonged period of cold-induced a higher ratio in the number of axillary buds/leaf in the hyper-branched mutants, revealing that axillary meristem formation can proceed in the absence of CCD7 and BRC1 activities. The rosette branches/leaf ratio was further increased in ccd7 following cold exposure indicating that bud outgrowth could be further enhanced in the absence of strigolactone. The extraordinarily higher number of axillary buds and axillary bud/leaf ratio displayed by met1 remained higher than WT following cold exposure, further evidencing that axillary meristem differentiation and bud formation were not impacted by prolonged cold treatment. Collectively, these evidences highlight the progression of axillary meristem differentiation and rosette bud outgrowth into a rosette branch that can continue during prolonged cold treatment of Arabidopsis juvenile plants.
Rather, our evidence revealed that the prolonged cold exposure dramatically enhanced the number of leaf primordia, irrespective of the genotype being hyper-branched, early or late in flowering time. That is, WT, hyper-branched (ccd7/max3, brc1), early flowering (sdg8), and late flowering (met1) mutants all generated additional rosette leaves during the extended cold exposure. This would be in part due to the extended period of vegetative development allowing additional leaf primordia to develop. Given that axillary meristem differentiation in the leaf axils was not affected by the cold treatment, and that WT showed a relatively lower rosette branch/leaf ratio, the cold treatment more likely caused a subtle increase in leaf primordia differentiation in the SAM. The increased differentiation of leaf primordia could explain why the brc1 and sdg8 mutants did not show a higher rosette branch/leaf ratio like that observed for ccd7/max3. It can also explain why early (sdg8) and late (met1) flowering mutants, as well as WT, all showed a lower axillary bud/leaf ratio after cold exposure. Therefore, the cold treatment may have slightly enhanced leaf primordia development to, thereby, lower the axillary bud/leaf and rosette branch/leaf ratios.
We have demonstrated that a prolonged period of cold exposure to Arabidopsis juvenile seedlings extended vegetative development to allow additional leaf primordia with axillary meristems to develop extra shoot branches, and delayed flowering to allow a larger inflorescence stem height with additional cauline branches to form that collectively enhanced plant biomass and seed yield (). In Arabidopsis, previous reports demonstrated that gene-specific differential DNA methylation can affect shoot branching via MET1-dependent transcriptional regulation of WUS (). The MET1-mediated methylation repressed WUS expression inhibiting shoot regeneration, while demethylation of the WUS promoter as in met1 mutants can activate WUS expression and promote axillary bud formation.Citation40,Citation49 In concert with the phenotypes of loss-in-function in MET1 and CCD7, axillary meristem formation and axillary bud outgrowth can proceed during a prolonged cold exposure of Arabidopsis juvenile seedlings. The juvenility of the plant and duration of cold exposure (1–5 weeks) are key factors that underpin the Arabidopsis growth response to the prolonged period of cold exposure. The application of prolonged cold exposure to juvenile seedlings of winter-annual species could be harnessed to enhance plant biomass and yield within a protected cropping environment.
Author contribution
CIC and ND conceived ideas. ND performed experiments, data collection and analyses and prepared figures. ND wrote the manuscript with input from CIC. CIC supervised ND.
Disclosure of potential conflicts of interest
No potential conflicts of interest were disclosed.
Acknowledgments
This work was partially supported by Australian Research Council Discovery Project Grant (DP130102593) to CIC. ND was supported by an International Australian Postgraduate research fellowship awarded by Western Sydney University, Australia.
Additional information
Funding
References
- Rameau C, Bertheloot J, Leduc N, Andrieu B, Foucher F, Sakr S. Multiple pathways regulate shoot branching. Front Plant Sci. 2015;5:1. doi:10.3389/fpls.2014.00741.
- Barbier FF, Dun EA, Kerr SC, Chabikwa TG, Beveridge CA. An Update on the Signals Controlling Shoot Branching. Trends Plant Sci. 2019;24(3):220–10. doi:10.1016/j.tplants.2018.12.001.
- Grbic V, Bleecker AB. Axillary meristem development in Arabidopsis thaliana. Plant J. 2000;21(2):215–223. doi:10.1046/j.1365-313x.2000.00670.x.
- Klepikova AV, Logacheva MD, Dmitriev SE, Penin AA. RNA-seq analysis of an apical meristem time series reveals a critical point in Arabidopsis thaliana flower initiation. BMC Genomics. 2015;16(1):466. doi:10.1186/s12864-015-1688-9.
- Liu C, Thong Z, Yu H. Coming into bloom: the specification of floral meristems. Development. 2009;136(20):3379–3391. doi:10.1242/dev.033076.
- Beveridge CA, Weller JL, Singer SR, Hofer JM. Axillary meristem development. Budding relationships between networks controlling flowering, branching, and photoperiod responsiveness. Plant Physiol. 2003;131(3):927–934. doi:10.1104/pp.102.017525.
- Long J, Barton MK. Initiation of axillary and floral meristems in Arabidopsis. Dev Biol. 2000;218(2):341–353. doi:10.1006/dbio.1999.9572.
- Jeong S, Clark SE. Photoperiod regulates flower meristem development in arabidopsis thaliana. Genetics. 2005;169(2):907–915. doi:10.1534/genetics.104.033357.
- Stirnberg P, van de Sande K, Leyser HMO. MAX1 and MAX2 control shoot lateral branching in Arabidopsis. Development. 2002;129:1131–1141.
- Taylor MA, Wilczek AM, Roe JL, Welch SM, Runcie DE, Cooper MD, Schmitt J. Large-effect flowering time mutations reveal conditionally adaptive paths through fitness landscapes in Arabidopsis thaliana. Proc Natl Acad Sci U S A. 2019;116(36):17890–17899. doi:10.1073/pnas.1902731116.
- Koornneef M, Hanhart CJ, van der Veen JH. van der Veen JH. A genetic and physiological analysis of late flowering mutants in Arabidopsis thaliana. Mol Gen Genet. 1991;229(1):57–66. doi:10.1007/BF00264213.
- Pouteau S, Albertini C. The significance of bolting and floral transitions as indicators of reproductive phase change in Arabidopsis. J Exp Bot. 2009;60(12):3367–3377. doi:10.1093/jxb/erp173.
- Lutz U, Pose D, Pfeifer M, Gundlach H, Hagmann J, Wang C, Weigel D, Mayer KFX, Schmid M, Schwechheimer C, et al. Modulation of ambient temperature-dependent flowering in arabidopsis thaliana by natural variation of FLOWERING LOCUS M. PLoS Genet. 2015;11(10):e1005588. doi:10.1371/journal.pgen.1005588.
- McClung CR, Lou P, Hermand V, Kim JA. The Importance of ambient temperature to growth and the induction of flowering. Front Plant Sci. 2016;7:1266. doi:10.3389/fpls.2016.01266.
- Michaels SD, Bezerra IC, Amasino RM. FRIGIDA-related genes are required for the winter-annual habit in Arabidopsis. Proc Natl Acad Sci U S A. 2004;101(9):3281–3285. doi:10.1073/pnas.0306778101.
- Hepworth J, Antoniou-Kourounioti RL, Bloomer RH, Selga C, Berggren K, Cox D, Collier Harris BR, Irwin JA, Holm S, Säll T, et al. Absence of warmth permits epigenetic memory of winter in Arabidopsis. Nat Commun. 2018;9(1):639. doi:10.1038/s41467-018-03065-7.
- Gomez-Roldan V, Fermas S, Brewer PB, Puech-Pages V, Dun EA, Pillot J-P, Letisse F, Matusova R, Danoun S, Portais J-C, et al. Strigolactone inhibition of shoot branching. Nature. 2008;455(7210):189–194. doi:10.1038/nature07271.
- Umehara M, Hanada A, Yoshida S, Akiyama K, Arite T, Takeda-Kamiya N, Magome H, Kamiya Y, Shirasu K, Yoneyama K, et al. Inhibition of shoot branching by new terpenoid plant hormones. Nature. 2008;455(7210):195–200. doi:10.1038/nature07272.
- Baranski R, Cazzonelli C. Carotenoid biosynthesis and regulation in plants. In: Kaczor A, Baranska M editors. Carotenoids: nutrition, analysis and technology. Wiley-Blackwell; 2016. p. 161–189.
- Jia K-P, Li C, Bouwmeester HJ, Al-Babili S. Strigolactone biosynthesis and signal transduction. In: Koltai H, Prandi C, editors. Strigolactones - biology and applications. Cham: Springer International Publishing; 2019. p. 1–45.
- Booker J, Auldridge M, Wills S, McCarty D, Klee H, Leyser O. MAX3/CCD7 is a carotenoid cleavage dioxygenase required for the synthesis of a novel plant signaling molecule. Current Biology: CB. 2004;14(14):1232–1238. doi:10.1016/j.cub.2004.06.061.
- Liu L, Xie T, Peng P, Qiu H, Zhao J, Fang J, Patil SB, Wang Y, Fang S, Chu J, et al. Mutations in the MIT3 gene encoding a caroteniod isomerase lead to increased tiller number in rice. Plant Sci. 2018;267:1–10. doi:10.1016/j.plantsci.2017.11.001.
- Cazzonelli CI, Cuttriss AJ, Cossetto SB, Pye W, Crisp P, Whelan J, Finnegan EJ, Turnbull C, Pogson BJ. Regulation of carotenoid composition and shoot branching in Arabidopsis by a chromatin modifying histone methyltransferase, SDG8. Plant Cell. 2009;21(1):39–53. doi:10.1105/tpc.108.063131.
- Aguilar-Martinez JA, Poza-Carrion C, Cubas P. Arabidopsis BRANCHED1 acts as an integrator of branching signals within axillary buds. Plant Cell. 2007;19(2):458–472. doi:10.1105/tpc.106.048934.
- Gonzalez-Grandio E, Poza-Carrion C, Sorzano CO, Cubas P. BRANCHED1 promotes axillary bud dormancy in response to shade in arabidopsis. Plant Cell. 2013;25(3):834–850. doi:10.1105/tpc.112.108480.
- Wang M, Le Moigne M-A, Bertheloot J, Crespel L, Perez-Garcia M-D, Oge L, Demotes-Mainard S, Hamama L, Davière J-M, Sakr S, et al. BRANCHED1: a key hub of shoot branching. Front Plant Sci. 2019;10:76. doi:10.3389/fpls.2019.00076.
- Seale M, Bennett T, Leyser O. BRC1 expression regulates bud activation potential but is not necessary or sufficient for bud growth inhibition in Arabidopsis. Development. 2017;144(9):1661–1673. doi:10.1242/dev.145649.
- Shen J, Zhang Y, Ge D, Wang Z, Song W, Gu R, Che G, Cheng Z, Liu R, Zhang X, et al. CsBRC1 inhibits axillary bud outgrowth by directly repressing the auxin efflux carrier CsPIN3 in cucumber. Proc Natl Acad Sci U S A. 2019;116(34):17105–17114. doi:10.1073/pnas.1907968116.
- Niwa M, Daimon Y, Kurotani K, Higo A, Pruneda-Paz JL, Breton G, Mitsuda N, Kay SA, Ohme-Takagi M, Endo M, et al. BRANCHED1 interacts with FLOWERING LOCUS T to repress the floral transition of the axillary meristems in arabidopsis. Plant Cell. 2013;25(4):1228–1242. doi:10.1105/tpc.112.109090.
- Hu Y, Zhang L, He S, Huang M, Tan J, Zhao L, YAN S, LI H, ZHOU K, LIANG Y, et al. Cold stress selectively unsilences tandem repeats in heterochromatin associated with accumulation of H3K9ac. Plant Cell Environ. 2012;35(12):2130–2142. doi:10.1111/j.1365-3040.2012.02541.x.
- Sherman JD, Talbert LE. Vernalization-induced changes of the DNA methylation pattern in winter wheat. Genome. 2002;45:253–260. doi:10.1139/g01-147.
- Banerjee A, Wani SH, Roychoudhury A. Epigenetic Control of Plant Cold Responses. Front Plant Sci. 2017;8:1643. doi:10.3389/fpls.2017.01643.
- Dennis ES, Peacock WJ. Epigenetic regulation of flowering. Curr Opin Plant Biol. 2007;10:520–527. doi:10.1016/j.pbi.2007.06.009.
- Soppe WJ, Bentsink L, Koornneef M. The early-flowering mutant efs is involved in the autonomous promotion pathway of Arabidopsis thaliana. Development (Cambridge, England). 1999;126:4763–4770.
- Cazzonelli CI, Millar T, Finnegan EJ, Pogson BJ. Promoting gene expression in plants by permissive histone lysine methylation. Plant Signal Behav. 2009;4(6):484–488. doi:10.4161/psb.4.6.8316.
- Cazzonelli CI, Roberts AC, Carmody ME, Pogson BJ. Transcriptional control of SET DOMAIN GROUP 8 and CAROTENOID ISOMERASE during arabidopsis development. Mol Plant. 2010;3(1):174–191. doi:10.1093/mp/ssp092.
- Liu B, Berr A, Chang C, Liu C, Shen W-H, Ruan Y. Interplay of the histone methyltransferases SDG8 and SDG26 in the regulation of transcription and plant flowering and development. Biochimica Et Biophysica Acta (BBA) - Gene Regulatory Mechanisms. 2016;1859(4):581–590. doi:10.1016/j.bbagrm.2016.02.003.
- Conde D, Le Gac A-L, Perales M, Dervinis C, Kirst M, Maury S, González-Melendi P, Allona I. Chilling-responsive DEMETER-LIKE DNA demethylase mediates in poplar bud break. Plant Cell Environ. 2017;40(10):2236–2249. doi:10.1111/pce.13019.
- Lister R, O’Malley RC, Tonti-Filippini J, Gregory BD, Berry CC, Millar AH, Ecker JR. Highly integrated single-base resolution maps of the epigenome in Arabidopsis. Cell. 2008;133(3):523–536. doi:10.1016/j.cell.2008.03.029.
- Liu H, Zhang H, Dong YX, Hao YJ, Zhang XS. DNA METHYLTRANSFERASE1-mediated shoot regeneration is regulated by cytokinin-induced cell cycle in Arabidopsis. New Phytol. 2017;217:219–232. doi:10.1111/nph.14814.
- Wang J, Tian C, Zhang C, Shi B, Cao X, Zhang T-Q, Zhao Z, Wang J-W, Jiao Y. Cytokinin signaling activates WUSCHEL expression during axillary meristem initiation. Plant Cell. 2017;29(6):1373–1387. doi:10.1105/tpc.16.00579.
- Mayer KF, Schoof H, Haecker A, Lenhard M, Jurgens G, Laux T. Role of WUSCHEL in regulating stem cell fate in the Arabidopsis shoot meristem. Cell. 1998;95(6):805–815. doi:10.1016/S0092-8674(00)81703-1.
- Laux T, Mayer KF, Berger J, Jurgens G. The WUSCHEL gene is required for shoot and floral meristem integrity in Arabidopsis. Development. 1996;122:87–96.
- Kankel MW, Ramsey DE, Stokes TL, Flowers SK, Haag JR, Jeddeloh JA, Riddle NC, Verbsky ML, Richards EJ. Arabidopsis MET1 cytosine methyltransferase mutants. Genetics. 2003;163:1109–1122.
- Ronemus MJ, Galbiati M, Ticknor C, Chen J, Dellaporta SL. Demethylation-induced developmental pleiotropy in Arabidopsis. Science. 1996;273(5275):654–657. doi:10.1126/science.273.5275.654.
- Park H, Kreunen SS, Cuttriss AJ, DellaPenna D, Pogson BJ. Identification of the carotenoid isomerase provides insight into carotenoid biosynthesis, prolamellar body formation, and photomorphogenesis. Plant Cell. 2002;14(2):321–332. doi:10.1105/tpc.010302.
- Schneider CA, Rasband WS, Eliceiri KW. NIH Image to ImageJ: 25 years of image analysis. Nat Methods. 2012;9(7):671–675. doi:10.1038/nmeth.2089.
- Wang B, Smith SM, Li J. Genetic regulation of shoot architecture. Annu Rev Plant Biol. 2018;69(1):437–468. doi:10.1146/annurev-arplant-042817-040422.
- Li W, Liu H, Cheng ZJ, Su YH, Han HN, Zhang Y, Zhang XS. DNA methylation and histone modifications regulate de novo shoot regeneration in Arabidopsis by modulating WUSCHEL expression and auxin signaling. PLoS Genet. 2011;7(8):e1002243. doi:10.1371/journal.pgen.1002243.
- Braun N, de Saint Germain A, Pillot J-P, Boutet-Mercey S, Dalmais M, Antoniadi I, Li X, Maia-Grondard A, Le Signor C, Bouteiller N, et al. The pea TCP transcription factor PsBRC1 acts downstream of Strigolactones to control shoot branching. Plant Physiol. 2012;158(1):225–238. doi:10.1104/pp.111.182725.
- Cazzonelli CI, Yin K, Pogson BJ. Potential implications for epigenetic regulation of carotenoid biosynthesis during root and shoot development. Plant Signal Behav. 2009;4(4):339–341. doi:10.4161/psb.4.4.8193.
- Huijser P, Schmid M. The control of developmental phase transitions in plants. Development. 2011;138(19):4117–4129. doi:10.1242/dev.063511.
- Amasino R. Seasonal and developmental timing of flowering. Plant J. 2010;61(6):1001–1013. doi:10.1111/j.1365-313X.2010.04148.x.
- Zhao Z, Yu Y, Meyer D, Wu C, Shen W-H. Prevention of early flowering by expression of FLOWERING LOCUS C requires methylation of histone H3 K36. Nat Cell Biol. 2005;7(12):1256–1260. doi:10.1038/ncb1329.
- Dong G, Ma D-P, Li J. The histone methyltransferase SDG8 regulates shoot branching in Arabidopsis. Biochem Biophys Res Commun. 2008;373(4):659–664. doi:10.1016/j.bbrc.2008.06.096.
- Cazzonelli CI, Nisar N, Roberts AC, Murray KD, Borevitz JO, Pogson BJ. A chromatin modifying enzyme, SDG8, is involved in morphological, gene expression, and epigenetic responses to mechanical stimulation. Front Plant Sci. 2014;5:533. doi:10.3389/fpls.2014.00533.
- Bakht J, Bano A, Shafi M, Dominy P. Effect of abscisic acid applications on cold tolerance in chickpea (Cicer arietinum L.). Eu J Agron. 2013;44:10–21. doi:10.1016/j.eja.2012.07.006.
- Ruan Y-L. Sucrose metabolism: gateway to diverse carbon use and sugar signaling. Annu Rev Plant Biol. 2014;65(1):33–67. doi:10.1146/annurev-arplant-050213-040251.
- Nagele T, Stutz S, Hormiller II, Heyer AG. Identification of a metabolic bottleneck for cold acclimation in Arabidopsis thaliana. Plant J. 2012;72(1):102–114. doi:10.1111/j.1365-313X.2012.05064.x.
- Yao C, Finlayson SA. Abscisic acid is a general negative regulator of arabidopsis axillary bud growth. Plant Physiol. 2015;169(1):611–626. doi:10.1104/pp.15.00682.
- Cooper JW, Hu Y, Beyyoudh L, Yildiz Dasgan H, Kunert K, Beveridge CA, Foyer CH. Strigolactones positively regulate chilling tolerance in pea and in Arabidopsis. Plant Cell Environ. 2018;41(6):1298–1310. doi:10.1111/pce.13147.
- Bertheloot J, Barbier F, Boudon F, Perez‐Garcia MD, Peron T, Citerne S, Dun E, Beveridge C, Godin C, Sakr S, et al. Sugar availability suppresses the auxin-induced strigolactone pathway to promote bud outgrowth. New Phytol. 2020;225(2):866–879. doi:10.1111/nph.16201.
- Brewer PB, Dun EA, Gui R, Mason MG, Beveridge CA. Strigolactone inhibition of branching independent of polar auxin transport. Plant Physiol. 2015;168(4):1820–1829. doi:10.1104/pp.15.00014.
- Brewer PB, Yoneyama K, Filardo F, Meyers E, Scaffidi A, Frickey T, Akiyama K, Seto Y, Dun EA, Cremer JE, et al. LATERAL BRANCHING OXIDOREDUCTASE acts in the final stages of strigolactone biosynthesis in Arabidopsis. Proc Natl Acad Sci U S A. 2016;113(22):6301–6306. doi:10.1073/pnas.1601729113.