ABSTRACT
Due to irrigation practices and industrial pollution, large areas of the lands in the world are simultaneously affected by salinity and heavy metal contamination. It has been considered that halophytes have adapted to salinity, and can be used to remediate heavy metal-contaminated saline soils. Suaeda salsa L. (S. salsa) is a high salt-resistance plant, which can efficiently absorb and accumulate salt and toxic metals from saline soils, suggesting that this may be potential plant species that can be used for the restoration of saline soils contaminated with heavy metals. The present brief review sheds light on the characteristics of S. salsa in the uptake and accumulation of high levels of heavy metals. Furthermore, the physiological and molecular mechanisms for heavy metal tolerance were highlighted. The potential values of S. salsa in the remediation of saline soils were also summarized.
Introduction
Soil salinity has been considered as the most devastating environmental stress restricting land usage and production of agricultural crops.Citation1,Citation2 It was estimated that 50% of cultivated land would be affected by salinization by 2050.Citation3 The yield loss induced by salinity would have a great impact on agriculture.Citation4 There is evidence that the economic loss caused by soil salinity is estimated to exceed 27 billion US$ per year.Citation4 At the same time, due to irrigation practices and industrial pollution, many areas in the world, particularly the coastal areas, and arid and semi-arid regions, are simultaneously affected by salinization and heavy metal contamination. This causes the saline soil to be contaminated with heavy metals, becoming a worldwide environmental problem.Citation5
Heavy metals are persistent contaminants in the environment, because these elements cannot be degraded by biological or chemical processes.Citation6 The most common heavy metals that can cause soil contamination include cadmium (Cd), lead (Pb), mercury (Hg), copper (Cu), and zinc (Zn).Citation6–11 High concentrations of heavy metals are toxic to plants and pose a serious threat to human health. To date, two strategies are used to remediate heavy metal-contaminated saline soils. One strategy is the use of the conventional strategies, such as soil washing, vapor extraction, electrokinetics, and thermal desorption, while the other strategy is phytoremediation.Citation12 Phytoremediation is defined as the use of specific plant species to remove or stabilize contaminants from the environment or to make these harmless.Citation12,Citation13 Compared with conventional strategies, phytoremediation has received increasing attention from many researchers due to its low cost, low invasiveness, and high safety.Citation12–14 However, most of the known phytoremediators are difficult to grow in a saline environment. Hence, these could not be used to repair heavy metal-contaminated saline soils (). Halophytes of approximately 1% of the global flora can survive and reproduce in environments where salt concentrations are approximately 0.8–4.2%, based on dry soil or more, and these tolerate salt concentrations that can kill up to 99% of other species.Citation15,Citation16 Furthermore, several studies have shown that certain halophytes may exhibit a high tolerance to heavy metals, and even accumulate high concentrations of bioavailable heavy metals in their tissues.Citation17 Thus, halophytes are the most suitable plant species for remediating saline soils contaminated with heavy metals.
Table 1. Common heavy metal hyperaccumulators
Suaeda salsa L. (S. salsa) is an euhalophyte that grows both on inland saline soil and intertidal zones ().Citation18,Citation19 This species has a high salt tolerance during seed germination,Citation20–23 seedling growth,Citation24,Citation25 and the reproductive stages.Citation26–28 This may be used for developing saline agriculture, since its fresh branches are highly valued as a vegetable, and the seeds contain edible oil rich in unsaturated fatty acids.Citation29–31 Furthermore, this species is a good model for understanding salt tolerance.Citation15,Citation32,Citation33 It has been reported that S. salsa has a high tolerance to heavy metals. For example, this can accumulate more arsenic (As), Pb, chromium (Cr), and Cu, when compared to Phragmites australis (Cav.) Trin. ex Steud., Spartina alterniflora Lois., and Typha orientalis Presl.Citation34 In addition, S. salsa has high ecological value and a broad planting prospect. For example, in a field investigation, 54.97% of the total desalination in soil can be reached by planting and harvesting the overground parts of S. salsa.Citation35 As a pioneer plant in saline soils, S. salsa may form a monospecific community when the concentration of salt in soils exceeds 20 g kg−1 of dry soil in inland saline soils or in the intertidal zones, indicating that S. salsa has potential for remediating and improving coastal saline soils contaminated with heavy metals.Citation36 In the present study, S. salsa was used as an example to evaluate the likelihood of using euhalophytes in the phytoremediation of salt-affected soils contaminated with heavy metals.
Heavy metal uptake, transfer and accumulation in S. salsa
The root system is one of the most important organs of plants. Plants rely on the root system to absorb heavy metals from the soil and accumulate these in the roots or aboveground part tissues through specific transfer processes. In general, the heavy metal uptake by plants depends on numerous factors, such as the speciation and bioavailability of metals in soil, the age and growth period of plants, seasonal variations, and metal characteristics.Citation12,Citation37 Salinity influences heavy metal speciation, increases metal mobility, and promotes the transfer of heavy metals from the roots to the shoots.Citation12,Citation38 Wang et al. reported that moderate NaCl additions enhanced the bioavailability of Pb in the rhizosphere, increased the Pb absorption and translocation from the roots to the shoots, and as a result, increased the total content of Pb in S. salsa.Citation38 In addition, the rhizosphere effect of plants can influence the bioavailability of heavy metals in the soil, thereby affecting the heavy metal uptake of plants.Citation12,Citation39
It appears that the Cd uptake by S. salsa is regulated by Ca2+ transporters or nonselective cation channels in root cell plasma membranes, as a root pretreatment with verapamil and LaCl3, which are two kinds of Ca2+ channel blockers that significantly suppress Cd influx to the roots.Citation40 The Cd uptake by S. salsa is also regulated by P-type ATPase. That is, the absorption of Cd by S. salsa is an energy-dependent process.Citation41 In addition, the linkage to proteins and low-molecular-weight SH-containing compounds is also an essential process for Cd uptake by S. salsa.Citation40 These results are compatible with the hypothesis of similar heavy metal uptake and transport mechanism between halophytes and glycophytes.Citation40 Surprisingly, there is no exhaustive finding on the precise mechanisms of heavy metal uptake and the corresponding genes involved in the regulation of the heavy metal uptake and accumulation in S. salsa. Further studies are needed to identify the modalities of heavy metal uptake, transport, and accumulation in S. salsa, especially the chemical form of heavy metal translocation, the mechanism of compartmentalization, and the metal transporters in S. salsa.
The accumulation of heavy metals in plants is the ultimate consequence of heavy metal uptake and translocation from the roots to the shoots.Citation41 The enrichment factor (EF) refers to the ratio of metal content in plant tissues to that in the soil. This parameter is used to indicate the efficiency of heavy metal accumulation in plants.Citation12 This differs according to the concentration and type of heavy metal in the environment.Citation12,Citation42 The transfer factor (TF) refers to the ratio of metal content in the shoots to that in the roots, and this coefficient indicates the efficiency of the heavy metal transfer from the roots to the shoots.Citation12,Citation42 S. salsa has a high capacity to accumulate Cd, Cu, Zn, and Pb from soil.Citation42 Several studies have reported that the maximum EF value of Cd, Cu, Zn, and Pb in S. salsa can reach 64.32, 24.80, 10.30, and 14.20, respectively ().Citation42,Citation43 Furthermore, it has been reported that after Zn was absorbed by the rhizosphere of S. salsa, more than half of these were transferred to the shoots, indicating that S. salsa has high efficiency in the transportation of Zn.Citation42 However, the study on the transfer coefficient of metal elements in S. salsa revealed that the roots of S. salsa have a stronger ability for Cu and Pb accumulation, but have low transfer efficiency, indicating that Cu and Pb would mainly accumulate in the roots, rather than in the shoots.Citation42 These findings may provide a stable theoretical basis for the phytoremediation of heavy metal-contaminated saline soils.
Table 2. The characteristics of absorption, translocation, and accumulation of certain heavy metals in Suaeda salsa.
Heavy metals toxicity in S. salsa
Heavy metals are toxic to plants at high concentrations. Metals, such as Zn and Cu, are essential for plant growth at low concentrations, but these can pose serious threats to plant growth when excessively absorbed by plants.Citation9,Citation10 Other metals, such as Cd, Pb, and Hg, are harmful to plants even at very low concentrations.Citation6 Metal toxicity inhibits plant growth and development mainly by imposing several constraints, such as oxidative stress, secondary osmotic stress, and ionic toxicity ().Citation44,Citation45 Using NMR-based metabolomics, Liu et al. reported the extensive metabolic alterations associated with osmotic regulation and energy metabolism induced by Cd in S. salsa.Citation46 In addition, Cong et al. confirmed that Cd exposure significantly modulates the expression of several genes (CAT, GST, and Prx Q) involved in the redox reaction.Citation47 These results suggest that Cd exposure can exert osmotic and oxidative stress on S. salsa. In addition, the abundance of a variety of proteins was characterized in S. salsa with 20 ug L−1 of Hg2+ and 500 mM of NaCl exposure for 30 days. The proteomic responses indicated that the functions of these proteins are involved in biological processes, such as material metabolism, photosynthesis, energy metabolism, stress response, signal transduction, immunosuppression, protein synthesis, and folding, which were significantly altered.Citation48,Citation49 Although excess heavy metals can disturb the normal physiological metabolism of plants, some tolerant plants, such as S. salsa, actually have a higher capacity to cope with heavy metal stress, when compared to sensitive plants, indicating that certain adaptive mechanisms must exist.Citation46–51
Heavy metals tolerance of S. salsa
Understanding the tolerance mechanism and genetic basis of heavy metal tolerance is very important in phytoremediation. Halophytes are naturally present in saline soils and can deal with the several abiotic stresses that frequently occur in their natural environment.Citation52 These plants are characterized by the significant accumulation of toxic ions, which were mainly Na+ and Cl−.Citation52 Studies have revealed that these specific features of halophytes make these confer tolerance to other toxic metal ions.Citation52 In other words, the tolerance to salinity and heavy metals may partly be based on common physiological and molecular mechanisms. Halophytes have evolved different strategies to withstand excess heavy metal ion toxicity.Citation52 Some adaptive strategies include the immobilization and exclusion of heavy metals to reduce the accumulation in plants, the chelation or compartmentalization the free metal ions in cells, the induction of oxidative stress defense systems, and the synthesis of osmotica and signaling molecules, including heat shock protein and hormones.Citation45,Citation52 Therefore, as a pioneer plant in saline soil, S. salsa exhibits good adaptability to heavy metal stress in saline soils ().
Exclusion, immobilization and exudation
The absorption of heavy metals by plants includes the root interception of metal ions, the entrance of metal ions into the roots, and the translocation from the roots to the shoots.Citation45 One strategy to reduce the toxicological effects of heavy metals is to prevent or lessen the excess heavy metals that enter the plant.Citation45 Under the stress of heavy metals, such as Cu, Pb, and Cd, S. salsa may use two approaches to prevent or lessen metal ions that enter into the roots. One approach is to precipitate heavy metals by increasing the pH of the plant rhizosphere or by excreting several anions such as phosphate.Citation45 The other approach is for S. salsa to increase their rhizosphere exudate, in order to form stable metal chelation complexes with free metal ions in the rhizosphere, there reducing the activity of heavy metal ions, and lessening the absorption of metal ions through the roots.Citation53,Citation54 In addition, it has been reported that iron plaques have a high capacity to adsorb heavy metals, which can allow heavy metal retention onto the root surface, and preventing these from being absorbed by plants.Citation12 However, there is no finding on the presence of iron plaque involved in heavy metal tolerance in S. salsa.
Cell walls may immobilize large portions of toxic heavy metal ions, preventing these from entering the cytoplasm, and affecting the intracellular metabolic activity.Citation12 For example, Halimione portulacoides (L.) Aellen immobilizes an average of 65% of heavy metal in the root cell wall.Citation55 The subcellular distribution analysis of S. salsa revealed that Cd was mainly distributed in the cell wall, followed by soluble components, such as the vacuole.Citation56 In addition, the plasma membrane has a negative polar charge that can also combine with heavy metal ions to prevent heavy metals from entering the cell.Citation45Citation104
Plants may use several strategies to deal with the toxicity of heavy metals after these have entered the cell. One strategy includes the transportation of heavy metals out of the cell or concentrating these into the vacuole.Citation57–59 The plasma membrane can also participate in heavy metal tolerance by increasing the efflux of metal ions through heavy transporter proteins.Citation45 Heavy metal excretion through leaf trichomes and salt glands has been frequently regarded as a possible detoxification mechanism in certain halophytes.Citation12,Citation60,Citation61 It has been reported that Sesuvium portulacastrum is able to increase the leaf succulence under the toxicity of heavy metal conditions.Citation62 S. salsa is a leaf succulence halophyte, that is, the leaf water content of S. salsa can reach 10 mL g−1 of dry weight, with an exposure of 600 mM of NaCl.Citation63 Toxic metals may concentrate in the vacuoles, and leaf succulence may contribute to the increase in the capacity of vacuole storage. This can help to improve the efficiency of metal detoxification in S. salsa.
Chelation and sequestration
Free metal ions in cytosol can be eliminated by chelation with specific high-affinity ligands, such as phytochelatins (PCs), metallothioneins (MTs), amino acids, and organic acids.Citation64,Citation65 PCs, a family of low-molecular-weight Cys-rich peptides, can form complexes with toxic metals in cytosol and contributes to its transportation into the vacuoles.Citation64 Glutathione (GSH) acts as a precursor to PCs synthesis.Citation45,Citation64 Fan reported that the content of non-protein thiol peptide (NPT), GSH, and PC in the roots of S. salsa increased under Cd-induced stress, which means that the root system of S. salsa may reduce the toxicity of metal ions by synthesizing a large number of non-protein sulfhydryl substances.Citation41 MTs are low-molecular-weight, intracellular, cysteine-rich metal proteins that efficiently bind to metals.Citation64 Two genes involved in metal chelation have been molecularly characterized in S. salsa.Citation66,Citation67 For example, the PCS gene (SsPCS) was obtained from S. salsa using the homologous cloning approach, and the expression levels of SsPCS in S. salsa are significantly upregulated after exposure to Pb and Hg.Citation66 The type 2 metallothionein gene, SsMT2, was cloned from S. salsa, and its functions were evaluated. The expression of the SsMT2 gene was upregulated after S. salsa was under Cd-induced stress. Furthermore, the overexpression of SsMT2 greatly increased the Cd accumulation and tolerance in transgenic yeast and Arabidopsis plants.Citation67 These results suggest that the SsMT2 gene may play an important role in the accommodation and detoxification of Cd stress in S. salsa plants. The successful cloning of the SsMT2 and SsPCS gene would help to better understand the mechanism of heavy metal tolerance in S. salsa, and provide a theoretical basis for the phytoremediation of heavy metal-contaminated saline soils in the future. Furthermore, organic acids, amino acids, and phosphate derivatives may play an important role in the response to metal toxicity in plants and may be involved in the tolerance of S. salsa to heavy metals.Citation65
The compartmentalization and concomitant detoxification of excess metal ions usually occur within the vacuole.Citation68,Citation69 Metal transporters are mainly involved in the storage or compartmentalization of excess heavy metal into vacuoles. Many studies have revealed that the ATP binding cassette (ABC), cation diffusion facilitators (CDF), heavy metal ATPases (HMA) transporters, cation/H+ exchanger antiporter (CAX), and natural resistance-associated macrophage protein (NRAMP) are correlated to vacuole metal sequestration and heavy metal selectivity.Citation68,Citation69 S. salsa has a high ability for ion compartmentalization, such as Na+ and Cl−, in vacuoles.Citation15 Therefore, it can be suggested that vacuole metal sequestration should be a strategy for the heavy metal tolerance of S. salsa.
Osmotic stress tolerance
When exposed to high concentrations of salt in soil, S. salsa meets the challenge of osmotic stress. In order to maintain the normal water content of plants, osmoprotectants, including inorganic and organic solutes, were accumulated to cope with the low external water potential.Citation70 Proline is an important organic osmoticum that often accumulates in cytosol under osmotic stress.Citation70 The euhalophyte S. salsa accumulates proline when growing in a high-salt environment, and the content of proline was 10–100 times of the initial value.Citation71 The high ability of S. salsa to quickly synthesize proline in response to the toxic effect of salinity may, to some extent, explain their good adaption to heavy metal stress. Pyrroline-5-carboxylate synthetase (P5CS) is an important enzyme involved in proline synthesis. The SsP5CS gene was cloned from S. salsa, and the expression of the SsP5CS gene was upregulated under osmotic stress.Citation72 In addition to its osmotic regulation function, proline also exhibits protective functions for enzymes, membranes, and ROS scavenging.Citation73 Khan et al. reported that the ethephon-induced accumulation of proline can protect the photosynthetic apparatus from the oxidative stress induced by Ni through maintaining water relations, and through decreasing oxidative stress parameters in Ni-stressed Indian mustard plants.Citation74 It has been reported that other amino acids, such as alanine, asparagine, glutamine, and histidine, can form complexes with heavy metals, and are involved in heavy metal tolerance.Citation65 For example, cysteine is required for the synthesis of MT and GSH/PC, which is regarded as a key metabolite in the antioxidant defense system and sequestration of metal.Citation12 S. salsa exhibits a clear increase in branch-chain amino acids, such as isoleucine, leucine, valine, phenylalanine, glutamate, glutamine, and tyrosine, under Cd stress, but it remains unclear whether this accumulation is due to a strategy of heavy metal stress tolerance or the results of protein degradation.Citation12
Glycinebetaine, which is another main organic osmotica, has been widely found at a high level in S. salsa under heavy metal stress.Citation46,Citation70,Citation75 Its physiological functions include participating in osmotic regulation to reduce ion toxicity and affect the intracellular ion distribution. Furthermore, the accumulation of glycinebetaine may contribute to the stabilization of the structure of macromolecules, and protection of chloroplasts and photosystem II.Citation70,Citation75 Using NMR-based metabolomics, the changes in metabolites induced by environmentally relevant concentrations (2, 10, and 50 μg L−1) of Cd were detected in S. salsa. The results revealed that all 1H NMR spectra were dominated by betaine and that the content of betaine in S. salsa tissues was approximately 10–100 times higher than that of other metabolites.Citation46 Choline monooxygenase (CMO) and betaine aldehyde dehydrogenase (BADH) are the two key enzymes involved in glycinebetaine synthesis.Citation15 In S. salsa, the SsBADH and SsCMO genes were cloned from S. salsa. Furthermore, transgenic tobacco with SsCMO significantly increased the ability of osmotic regulation.Citation15
Myo-inositol-1-phosphate synthase (MIPS) plays an important role in myo-inositol synthesis and contributes to the growth, development, oxidation, and cellular defense of plant cells.Citation47,Citation76 The MIPS transcripts were significantly upregulated under Cd stress in S. salsa. Furthermore, MIPS is a key enzyme that catalyzes the synthesis of myo-inositol, and the metabolic products (such as myo-inositol hexakisphosphate) impact the uptake and transfer of heavy metals. Furthermore, the metabolic pathway of myo-inositol and the detoxification function of its downstream products may be modified under Cd stress.Citation12,Citation47,Citation76
Oxidative stress tolerance
Halophytes possess powerful antioxidant systems to cope with the reactive oxygen species (ROS) induced by metal ions. Halophytes can induce the synthesis for the production of antioxidants, which can be enzymatic, including ROS scavenging enzymes, such as superoxide dismutase (SOD), ascorbate peroxidase (APX), peroxidase (POD), catalase (CAT), peroxiredoxin reductase (PrxR), glutathione S-transferase (GST) and some ascorbic acid (AsA)-glutathione (GSH) cycle enzymes, or non-enzymatic, including low-molecular-mass antioxidants (GSH, AsA and carotenoids).Citation77 Studies have revealed that the SOD dismutation, GPX pathway, PrxR pathway, CAT pathway, and AsA-GSH cycle may play the main role in scavenging the ROS produced by salt stress in S. salsa.Citation77 Cong et al. reported that the expression levels of some antioxidant enzyme genes, including the GST, PrxQ, and CAT2 genes, were upregulated in response to Cd stress in S. salsa.Citation47 In the same plant species, the activities of the antioxidant enzymes SOD, glutathione peroxidase (GPx), and CAT increased in response to Zn stress, or the combined stress of Zn and Pb.Citation51 These results show that different metal stresses can synergistically regulate the high-efficiency antioxidant system of S. salsa. Antioxidants such as glutathione (GSH) assume crucial functions in balancing the redox relations under heavy metal stress. Since GST acts by promoting the conjugation of GSH with some electrophilic substances, such as heavy metals and aromatic hydrocarbons, the activity of GST increases under Cd stress. This may imply that there are more GSHs involved in the formation Cd-binding complexes that alleviate the toxic effects of Cd.Citation47 Several studies have revealed that betacyanin, flavonoids, and some other compounds, such as quercetin, isorhamnetin, 2,4-dihydroxybenzoicacid, and 2-hydroxygenistein, may play an important role as a non-enzymatic antioxidant agent and regulatory substance in S. salsa.Citation32
Zhou et al. reported that SsPETE2, which is a plastocyanin gene obtained from euhalophyte S. salsa, has a novel antioxidant function, which is involved in the copper-chelating activity of SsPETE2. In S. salsa, the expression of the SsPETE2 gene was upregulated to deal with the oxidative stress, and the transgenic Arabidopsis with SsPETE2 exhibited more antioxidant ability.Citation78
It has been reported that the SOD activity possesses high efficiency in protecting halophytes from adverse environmental changes. The activities of different isoforms of SOD, including CuZn-SOD, Mn-SOD, and Fe-SOD, were detected in S. salsa, and the results revealed that these SOD isoforms may contribute to the increase in tolerance of oxidative stress.Citation79 Furthermore, SsGST, SsPrxQ, SsCAT1, SsCAT2, SsAPX, and SsTypA1, which are the genes related to the oxidative stress tolerance of S. salsa, were cloned, and their functions were tested.Citation80–84 The overexpression of the Ss.sAPX gene in transgenic Arabidopsis plants exhibited an obvious increase in germination, survival rate, cotyledon growth, and salt tolerance.Citation15 This means that S. salsa has a high ability in dealing with salt-induced oxidative stress. However, further studies are needed to determine whether the ability to cope with oxidative stress is correlated to heavy metal tolerance in S. salsa.
The potential of S. salsa in the remediation of heavy metal-contaminated saline soils
Compared with the conventional approaches to remediate heavy metal-contaminated saline soil, phytoremediation is a more environmentally friendly technology that can be efficiently applied to immobilize, degrade, and remove contaminants.Citation12 There are five different phytoremediation treatments for remediating heavy metal-contaminated environments: phytoextraction, phytofiltration, phytostabilization, phytodegradation, and phytovolatilization.Citation12,Citation16 Among these, phytoextraction and phytostabilization have been highlighted as the most common techniques for the remediation of HM-contaminated saline soil.Citation16,Citation39
Phytoextraction was defined as using plants to remove toxic metals from soil by accumulating these in harvestable plant parts.Citation16 Usually, hyperaccumulators and high-biomass plants are the two ideal candidates for phytoextraction.Citation16 It has been reported that the dry mass of the above-ground biomass of S. salsa under high-salinity cultivation in the field could reach up to 18,978 kg·hm−2, which is 1.8 times higher than that for Cd-hyperaccumulator Solanum nigrum L. and 6.3 times higher than that for Cd-hyperaccumulator Viola-baoshanensis Shu, Liu et Lan (Violaceae).Citation43,Citation85 The high biomass of S. salsa has great potential in the practice of the phytoremediation of heavy metal-contaminated saline soils. The latest findings have shown that S. salsa can accumulate more Cr, Cu, Pb, and As, when compared to other halophytes, such as P. australis, S. alterniflora, and T. orientalis.Citation34 Furthermore, S. salsa has an advantage over P. australis and Limonium bicolor (Bunge) Kuntze in terms of tolerance to Cd stress and the remediation of Cd-contaminated saline soils.Citation86 In terms of the ability of uptake, translocation, and extraction of Cd, S. salsa exhibits great advantages over other halophytes, such as Melilotus suaveolens Ledeb., Limonium gmelinii (Willd.) Kuntze and Atriplex aucheri Moq.Citation43 The growth of S. salsa reduced the content of Cd in soil and changed the chemical binding state of Cd in soil around the roots.Citation43 In a sand culture pot experiment, S. salsa accumulated 132.12 mg·kg−1 of Cd in the above-ground part tissues, and the extraction rate of Cd was 70.12% under the stress of Cd and salt.Citation43 In a field experiment, S. salsa concentrated 296.7 mg·kg−1 of Zn in the plant tissues, and more than half of these were transferred to the stems and leaves.Citation42 These findings suggest that S. salsa has strong application potential to remediate the Zn- and Cd-contaminated saline soils.
Phytostabilization was defined as the use of plants to immobilize metal ions by accumulating these in the roots or precipitating these within the rhizosphere.Citation16 Phytostabilization is a feasible approach for stabilizing toxic heavy metals in saline soils. This aims to establish a persistent vegetation cover that maintains the stabilization of metal ions in the soil, protecting the groundwater, crops, and soil from heavy metal contamination.Citation16 S. salsa can simultaneously over-accumulate toxic metals, such as Cu and Pb, in their roots, limiting the translocation to the shoots.Citation43 For example, with the addition of soil Cu (50–1,500 mg·kg−1), the content of Cu increased to values within 96–955 mg· kg−1 in the roots of S. salsa.Citation87 This indicates that S. salsa has great potential to be used as a Cu phytostabilizor in saline soils.
S. salsa is an euhalophyte and is one of the important vegetations in high-salinity habitats.Citation15 Many studies have shown that planting S. salsa can significantly reduce the salt content, and improve the physical and chemical properties of soil.Citation88–90 For example, in a field plot experiment, S. salsa removed 2,116 kg·hm−2 of salinity from saline soil.Citation90 Furthermore, S. salsa accumulated Cd and Zn in the shoots and Cu and Pb in the roots. This can be used as a potential phytoextractor and phytostabilizer in the remediation of heavy metal-contaminated saline soils (). In addition, S. salsa plays a certain role in purifying the coastal ecosystem. It has been shown that S. salsa can increase soil organic matter and total soil nitrogen, when compared with soils that are not planted with S. salsa.Citation15 Meanwhile, planting S. salsa increases the amount of actinomyces and fungi.Citation15 Furthermore, in terms of the ability to decompose the total petroleum hydrocarbon content in soil, S. salsa exhibits great advantages over Salicornia europaea Linn. and A. aucheri.Citation91 This suggests that planting S. salsa can be used as a potential species for repairing coastal saline soils contaminated by heavy metals.
Figure 3. Schematic presentation of the phytoremediation processes by Suaeda salsa (adapted from Rascio and Navari-Izzo, 2010): (1) Metal ions are absorbed at the root surface; (2) Bioavailable metals bind to the cell wall or enter the cytosol; (3) Chelation in the cytosol; (4) The sequestration of metal ions in the vacuole; (5) The metal efflux from the cytosol into the apoplast through heavy metal transporter proteins; (6–7) Roots-to-shoots translocation; (8) Metals bind to the cell wall or enter the cytosol; (9) Chelation in the cytosol; (10) The sequestration of metal ions in the vacuole; (11) The metal efflux from cytosol into the apoplast through heavy metal transporter proteins
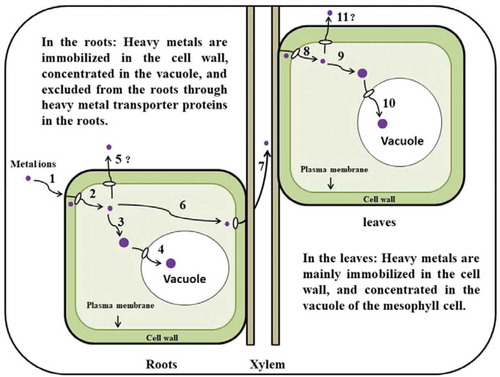
Concluding remarks and perspectives
In general, the advantages and feasibility of using S. salsa to remediate saline soils contaminated by heavy metals rely on the following: (1) the specific mechanisms to survive under high salinity and heavy metal stress; (2) the salinity can activate heavy metals in soils and improve their bioavailability, thereby promoting the efficiency of heavy metal absorption by S. salsa; (3) S. salsa can extract or stabilize large amounts of heavy metals from saline soils. Meanwhile, S. salsa can actively absorb and accumulate salts from saline soils, thereby improving the quality of saline soils.Citation39 Although phytoremediation has the advantages of low cost, green and high safety, and great progress has been made in the remediation of heavy metal pollution in saline soils in recent years, due to its limitations, it also faces certain challenges in practical applications. For example, salinity frequently improves the bioavailability of heavy metals to a certain extent, which is conducive to its absorption and accumulation in S. salsa. Furthermore, when the bioavailability of heavy metals increases, its toxicological effects on S. salsa correspondingly increases. When the heavy metal content of saline soils exceeds its ability of heavy metal tolerance, S. salsa would be damaged. At present, the use of S. salsa to remediate and improve saline soils contaminated by heavy metals is limited to the experimental stage. More experiments should be conducted for S. salsa, in terms of the efficiency in removing heavy metals from saline soils in the field.
Author contributions
Cailing Shang wrote the manuscript. Lei Wang and Changyan Tian assisted in revising the manuscript. Jie Song revised and gave the final approval of the manuscript.
Disclosure statement
No potential conflicts of interest were disclosed.
Additional information
Funding
References
- Munns R. Genes and salt tolerance: bringing them together. New Phytol. 2005;167(3):1–9. doi:https://doi.org/10.1111/j.1469-8137.2005.01487.x.
- Munns R, Tester M. Mechanisms of salinity tolerance. Annu Rev Plant Biol. 2008;59:651–681. doi:https://doi.org/10.1146/annurev.arplant.59.032607.092911.
- Butcher K, Wick AF, Desutter T, Chatterjee A, Harmon J. Soil salinity: a threat to global food security. Agron J. 2016;108:2189–2200. doi:https://doi.org/10.2134/agronj2016.06.0368.
- Christian Z, Christoph-Martin G, Karl-Josef D. Salinity and crop yield. Plant Biol. 2018;21:31–38. doi:https://doi.org/10.1111/plb.12884.
- Wang HL, Tian CY, Jiang L, Wang L. Remediation of heavy metals contaminated saline soils: a halophyte choice? Environ Sci Technol. 2014;48:21–22. doi:https://doi.org/10.1021/es405052j.
- Nagajyoti PC, Lee KD, Sreekanth TVM. Heavy metals, occurrence and toxicity for plants: a review. Environ Chem Lett. 2010;8:199–216. doi:https://doi.org/10.1007/s10311-010-0297-8.
- Anjum SA, Tanveer M, Hussain S, Shahzad B, Ashraf U, Fahad S, Hassan W, Jan S, Khan I, Saleem MF, et al. Osmoregulation and antioxidant production in maize under combined cadmium and arsenic stress. Environ Sci Pollut R. 2016;23:11864–11875. doi:https://doi.org/10.1007/s11356-016-6382-1.
- Patra M, Bhowmik N, Bandopadhyay B, Sharma A. Comparison of mercury, lead and arsenic with respect to genotoxic effects on plant systems and the development of genetic tolerance. Environ Exp Bot. 2004;52:199–223. doi:https://doi.org/10.1016/j.envexpbot.2004.02.009.
- Adrees M, Ali S, Rizwan M, Ibrahim M, Abbas F, Farid M, Zia-ur-Rehman M, Irshad MK, Bharwana SA. The effect of excess copper on growth and physiology of important food crops: a review. Environ Sci Pollut R. 2015;22:8148–8162. doi:https://doi.org/10.1007/s11356-015-4496-5.
- Yang YQ, Sun CC, Yao YN, Zhang YM, Achal V. Growth and physiological responses of grape (Vitis vinifera “Combier”) to excess zinc. Acta Physiol Plant. 2011;33(4):1483–1491. doi:https://doi.org/10.1007/s11738-010-0687-3.
- Sharma VK, Li XY, Wu GL, Bai WX, Parmar S, White JF Jr, Li HY. Endophytic community of Pb-Zn hyperaccumulator Arabis alpina and its role in host plants metal tolerance. Plant Soil. 2019;437:397–411. doi:https://doi.org/10.1007/s11104-019-03988-0.
- Lutts S, Lefèvre I. How can we take advantage of halophyte properties to cope with heavy metal toxicity in salt-affected areas? Ann Bot. 2015;115:509–528. doi:https://doi.org/10.1093/aob/mcu264.
- Saxena G, Purchase D, Mulla SI, Saratale GD, Bharagava RN. Phytoremediation of heavy metal-contaminated sites: eco-environmental concerns, field studies, sustainability issues, and future prospects. Rev Environ Contamt. 2019;249:71–131. doi:https://doi.org/10.1007/398_2019_24.
- Sarwar N, Imran M, Shaheen MR, Ishaque W, Kamran MA, Matloob A, Rehim A, Hussain S. Phytoremediation strategies for soils contaminated with heavy metals: modifications and future perspectives. Chemosphere. 2017;171:710–721. doi:https://doi.org/10.1016/j.chemosphere.2016.12.116.
- Song J, Wang BS. Using euhalophytes to understand salt tolerance and to develop saline agriculture: suaeda salsa as a promising model. Ann Bot. 2015;115:541–553. doi:https://doi.org/10.1093/aob/mcu194.
- Liang LC, Liu WT, Sun YB, Huo XH, Li S, Zhou QX. Phytoremediation of heavy metal contaminated saline soils using halophytes: current progress and future perspectives. Environ Rev. 2017;25:269–281. doi:https://doi.org/10.1139/er-2016-0063.
- Van Oosten MJ, Maggio A. Functional biology of halophytes in the phytoremediation of heavy metal contaminated soils. Environ Exp Bot. 2015;111:135–146. doi:https://doi.org/10.1016/j.envexpbot.2014.11.010.
- Wang FX, Xu YG, Wang S, Shi WW, Liu RR, Feng G, Song J. Salinity affects production and salt tolerance of dimorphic seeds of Suaeda salsa. Plant Physiol Biochem. 2015;95:41–48. doi:https://doi.org/10.1016/j.plaphy.2015.07.005.
- Wang FX, Yin CH, Song YP, Li Q, Tian CY, Song J. Reproductive allocation and fruit-set pattern in the euhalophyte Suaeda salsa in controlled and field conditions. Plant Biosyst. 2017;152:749–758. doi:https://doi.org/10.1080/11263504.2017.1330776.
- Song J, Zhou JC, Zhao WW, Xu HL, Wang FX, Xu YG, Wang L, Tian CY. Effects of salinity and nitrate on production and germination of dimorphic seeds both applied through the mother plant and exogenously during germination in Suaeda salsa. Plant Spec Biol. 2016;31:19–28. doi:https://doi.org/10.1111/1442-1984.12071.
- Song J, Shi WW, Liu RR, Xu YG, Sui N, Zhou JC, Feng G. The role of the seed coat in adaptation of dimorphic seeds of the euhalophyte Suaeda salsa to salinity. Plant Spec Biol. 2017;32:107–114. doi:https://doi.org/10.1111/1442-1984.12132.
- Xu YG, Liu RR, Sui N, Shi WW, Wang L, Tian CY, Song J. Changes in endogenous hormones and seed coat phenolics during seed storage of two Suaeda salsa populations. Aust J Bot. 2016;64:325–332. doi:https://doi.org/10.1071/BT16014.
- Zhou JC, Fu TT, Sui N, Guo JR, Feng G, Fan JL, Song J. The role of salinity in seed maturation of the euhalophyte Suaeda salsa. Plant Biosyst. 2016;150:83–90. doi:https://doi.org/10.1080/11263504.2014.976294.
- Song J, Shi GW, Gao B, Fan H, Wang BS. Waterlogging and salinity effects on two Suaeda salsa populations. Physiol Plantarum. 2011;141:343–351. doi:https://doi.org/10.1111/j.1399-3054.2011.01445.x.
- Liu QQ, Liu RR, Ma YC, Song J. Physiological and molecular evidence for Na+ and Cl− exclusion in the roots of two Suaeda salsa populations. Aquat Bot. 2018;146:1–7. doi:https://doi.org/10.1016/j.aquabot.2018.01.001.
- Guo JR, Dong XX, Han GL, Wang BS. Salt-enhanced reproductive development of Suaeda salsa L. coincided with ion transporter gene upregulation in flowers and increased pollen K+ content. Front Plant Sci. 2019;10:333. doi:https://doi.org/10.3389/fpls.2019.00333.
- Guo JR, Suo SS, Wang BS. Sodium chloride improves seed vigour of the euhalophyte Suaeda salsa. Seed Sci Res. 2015;25:335–344. doi:https://doi.org/10.1017/S0960258515000239.
- Guo JR, Li YD, Han GL, Song J, Wang BS. NaCl markedly improved the reproductive capacity of the euhalophyte Suaeda salsa. Funct Plant Biol. 2017;45:350–361. doi:https://doi.org/10.1071/FP17181.
- Zhao YQ, Ma YC, Li Q, Yang Y, Guo JR, Song J. Utilisation of stored lipids during germination in dimorphic seeds of euhalophyte Suaeda salsa. Funct Plant Biol. 2018;45:1009–1016. doi:https://doi.org/10.1071/FP17309.
- Zhao YQ, Yang Y, Song YP, Li Q, Song J. Analysis of storage compounds and inorganic ions in dimorphic seeds of euhalophyte Suaeda salsa. Plant Physiol Bioch. 2018;130:511–516. doi:https://doi.org/10.1016/j.plaphy.2018.08.003.
- Zhao YQ, Ma YC, Duan HM, Liu RR, Song J. Traits of fatty acid accumulation in dimorphic seeds of the euhalophyte Suaeda salsa in saline conditions. Plant Biosyst. 2018;153:514–520. doi:https://doi.org/10.1080/11263504.2018.1508090.
- Li Q, Song J. Analysis of widely targeted metabolites of the euhalophyte Suaeda salsa under saline conditions provides new insights into salt tolerance and nutritional value in halophytic species. BMC Plant Biol. 2019;19:388. doi:https://doi.org/10.1186/s12870-019-2006-5.
- Sui N, Tian SS, Wang WQ, Wang MJ, Fan H. Overexpression of glycerol-3-phosphate acyltransferase from Suaeda salsa improves salt tolerance in Arabidopsis. Front Plant Sci. 2017;8:1337. doi:https://doi.org/10.3389/fpls.2017.01337.
- Gao YF, Li XQ, Dong GC, Liu F, Wang YN, ke H. Purification of several salt marsh plants to the coastal wetlands in the estuary of Yellow River. J Anhui Agric Sci. 2010;38:19499–19501. [ in Chinese].
- Pan SX, Sun LB, Zhang SM, Maimaitiaili BDS, Jia HT, Feng G. Growth regularity of Suaeda salsa L. and its effects on salt soil improvement in the northern Xinjiang. Acta Agric Boreali-Occidentalis Sin. 2014;23:201–206. [in Chinese].
- Gu FT. Research in exploiting the green series of edibles-Suaeda Salsa. J Binzhou Educ Coll. 1999;5:43–48. [ in Chinese].
- Cacador I, Vale C, Catarino F. Seasonal variation of Zn, Pb, Cu and Cd concentrations in the root-sediment system of Spartina maritima and Halimione portulacoides from Tagus estuary salt marshes. Mar Environ Res. 2000;49:279–290. doi:https://doi.org/10.1016/s0141-1136(99)00077-x.
- Wang FL, Song NN. Salinity-induced alterations in plant growth, antioxidant enzyme activities, and lead transportation and accumulation in Suaeda salsa: implications for phytoremediation. Ecotoxicology. 2019;28:520–527. doi:https://doi.org/10.1007/s10646-019-02048-8.
- Liang LC, Liu WT, Zhang X, Chen C, Huo XH, Li S. Research progress in phytoextraction of heavy metal contaminated saline soil. J Agro Environ Sci. 2016;35:1233–1241. [ in Chinese].
- Li LZ, Liu XL, Peijnenburg WJGM, Zhao JM, Chen XB, Yu JB, Wu HF. Pathways of cadmium fluxes in the root of the halophyte Suaeda salsa. Ecotox Environ Safe. 2012;75:1–7. doi:https://doi.org/10.1016/j.ecoenv.2011.09.007.
- Fan XR. Study on the mechanism of absorption and accumulation of heavy metal Cd in roots of Suaeda heteroptera [MSc thesis]. Dalian (China): Dalian Ocean University; 2019. [ in Chinese].
- Zhu MH, Ding YS, Ding DW. Seasonal variation about accumulation distribution and transference of heavy metals in Suaeda heteroptera. China Environ Sci. 2006;26:110–113. [ in Chinese].
- Chen KH. Phytoextraction in Cd or Cd-Pb contaminated soils by Suaeda salsa [MSc thesis]. Guangzhou (China): Jinan University; 2017. [ in Chinese].
- Patra DK, Pradhan C, Patra HK. Toxic metal decontamination by phytoremediation approach: concept, challenges, opportunities and future perspectives. Environ Technol Inno. 2020;18:100672. doi:https://doi.org/10.1016/j.eti.2020.100672.
- Hossain MA, Piyatida P, Silva JAT, Fujita M. Molecular mechanism of heavy metal toxicity and tolerance in plants: central role of glutathione in detoxification of reactive oxygen species and methylglyoxal and in heavy metal chelation. J Bot. 2012;2012:1–37. doi:https://doi.org/10.1155/2012/872875.
- Liu XL, Yang CY, Zhang LB, Li LZ, Liu SJ, Yu JB, You LP, Zhou D, Xia CH, Zhao JM, et al. Metabolic profiling of cadmium-induced effects in one pioneer intertidal halophyte Suaeda salsa by NMR-based metabolomics. Ecotoxicology. 2011;20:1422–1431. doi:https://doi.org/10.1007/s10646-011-0699-9.
- Cong M, Lv J, Liu XL, Zhao JM, Wu HF. Gene expression responses in Suaeda salsa after cadmium exposure. SpringerPlus. 2013;2:232. doi:https://doi.org/10.1186/2193-1801-2-232.
- Liu XL, Wu HF, Ji CL, Wei L, Zhao JM, Yu JB. An integrated proteomic and metabolomic study on the chronic effects of mercury in Suaeda salsa under an environmentally relevant salinity. PLoS One. 2013;8:e64041. doi:https://doi.org/10.1371/journal.pone.0064041.
- Wu HF, Liu XL, Zhao JM, Yu JB. Toxicological responses in halophyte Suaeda salsa to mercury under environmentally relevant salinity. Ecotox Environ Safe. 2012;85:64–71. doi:https://doi.org/10.1016/j.ecoenv.2012.03.016.
- Liu XL, Shen XJ, Lai YK, Ji K, Sun HS, Wang YY, Hou CZ, Zou N, Wan JL, Yu JB. Toxicological proteomic responses of halophyte Suaeda salsa to lead and zinc. Ecotox Environ Safe. 2016;134:163–171. doi:https://doi.org/10.1016/j.ecoenv.2016.07.017.
- Wu HF, Liu XL, Zhao JM, Yu JB. Regulation of metabolites, gene expression, and antioxidant enzymes to environmentally relevant lead and zinc in the halophyte Suaeda salsa. J Plant Growth Regul. 2013;32:353–361. doi:https://doi.org/10.1007/s00344-012-9305-5.
- Nikalje GC, Suprasanna P. Coping with metal toxicity – cues from halophytes. Front Plant Sci. 2018;9:777. doi:https://doi.org/10.3389/fpls.2018.00777.
- Xin Q, Liu CF, Liu Y, Wang YT, Wei HF, Li J. Secretion of total organic carbon and inorganic carbon from roots of seepweed Suaeda heteroptera in response to Zn (II) stress. Chin J Appl Environ Biol. 2014;20:134–138. doi:https://doi.org/10.3724/SP.J.1145.2014.00134. [ in Chinese].
- Liu H. Effect of extracellular products of roots of Suaeda heteroptera on Cd removal by bacteria [MSc thesis]. Dalian (China): Dalian Ocean University; 2019. [ in Chinese].
- Sousa AI, Cac¸ador I, Lillebø AI, Pardal MA. Heavy metal accumulation in Halimione portulacoides: intra- and extra-cellular metal binding sites. Chemosphere. 2008;710:850–857. doi:https://doi.org/10.1016/j.chemosphere.2007.07.012.
- Yang YZ, Zhang CH, Zheng QS, Zhang CY, Ge Y. Uptake, translocation and subcellular distribution characteristics of cadmium and sodium in Suaeda salsa and Atriplex triangularis. J Agro Environ Sci. 2015;34:619–626. [ in Chinese].
- DalCorso G, Farinati S, Maistri S, Furini A. How plants cope with cadmium: staking all on metabolism and gene expression. J Integr Plant Biol. 2008;50:1268–1280. doi:https://doi.org/10.1111/j.1744-7909.2008.00737.x.
- DalCorso G, Farinati S, Furini A. Regulatory networks of cadmium stress in plants. Plant Signal Behav. 2010;5:663–667. doi:https://doi.org/10.4161/psb.5.6.11425.
- Clemens S. Molecular mechanisms of plant metal tolerance and homeostasis. Planta. 2001;212:475–486. doi:https://doi.org/10.1007/s004250000458.
- McFarlane GR, Burchett MD. Zinc distribution and excretion in the leaves of the grey mangrove Avicennia marina (Forsk.) Vierh. Environ Exp Bot. 1999;41:167–175. doi:https://doi.org/10.1016/s0098-8472(99)00002-7.
- Manousaki E, Kadukova J, Papadantonakis N, Kalogerakis N. Phytoextraction and phytoexcretion of Cd by the leaves of Tamarix smyrnensis growing on contaminated non-saline and saline soils. Environ Res. 2008;106:326–332. doi:https://doi.org/10.1016/j.envres.2007.04.004.
- Wang DY, Wang HY, Han B, Wang B, Guo AP, Zheng D, Liu CJ, Chang LL, Peng M, Wang XC. Sodium instead of potassium and chloride is an important macronutrient to improve leaf succulence and shoot development for halophyte Sesuvium portulacastrum. Plant Physiol Bioch. 2012;51:53–62. doi:https://doi.org/10.1016/j.plaphy.2011.10.009.
- Song J, Chen M, Feng G, Jia YH, Wang BS, Zhang FS. Effect of salinity on growth, ion accumulation and the roles of ions in osmotic adjustment of two populations of Suaeda salsa. Plant Soil. 2009;314:133–141. doi:https://doi.org/10.1007/s11104-008-9712-3.
- Cobbett C, Goldsbrough P. Phytochelatins and metallothionein: roles in heavy metal detoxification and homeostasis. Annu Rev Plant Biol. 2002;53:159–182. doi:https://doi.org/10.1146/annurev.arplant.53.100301.135154.
- Sharma SS, Dietz K. The significance of amino acids and amino acid-derived molecules in plant responses and adaptation to heavy metal stress. J Exp Bot. 2006;57:711–726. doi:https://doi.org/10.1093/jxb/erj073.
- Cong M, Zhao JM, Lü JS, Ren ZM, Wu HM. Homologous cloning, characterization and expression of a new halophyte phytochelatin synthase gene in Suaeda salsa. Chin J Oceanol Limnol. 2016;34:1034–1043. doi:https://doi.org/10.1007/s00343-016-4382-0.
- Jin SM, Xu C, Li GL, Sun D, Li Y, Wang XW, Liu SK. Functional characterization of a type 2 metallothionein gene, SsMT2, from alkaline-tolerant Suaeda salsa. Sci Rep. 2017;7:17914. doi:https://doi.org/10.1038/s41598-017-18263-4.
- Tong YP, Kneer R, Zhu YG. Vacuolar compartmentalization: a second-generation approach to engineering plants for phytoremediation. Trends Plant Sci. 2004;9:7–9. doi:https://doi.org/10.1016/j.tplants.2003.11.009.
- Martinoia E, Maeshima M, Neuhaus HE. Vacuolar transporters and their essential role in plant metabolism. J Exp Bot. 2006;58:83–102. doi:https://doi.org/10.1093/jxb/erl183.
- Slama I, Abdelly C, Bouchereau A, Flowers T, Savoure´ A. Diversity, distribution and roles of osmoprotective compounds accumulated in halophytes under abiotic stress. Ann Bot. 2015;115:433–447. doi:https://doi.org/10.1093/aob/mcu239.
- Cao SY, Xie QY, Yi K, Liu L. Research progress on salt tolerance mechanisms of Suaeda heteroptera. Mod Agric Sci Technol. 2018;5:169–171. [ in Chinese].
- Wang PP, Ma CL, Zhao KF, Zhao YX, Zhang H. Isolation and characterizing of a D1-pyrroline-5-carboxylate synthase gene in Suaeda salsa under salinity stress. J Shandong Normal Univ (Nat Sci). 2002;17:59–62. [ in Chinese].
- Wang FJ, Zeng B, Sun ZX, Zhu C. Relationship between proline and Hg2+-induced oxidative stress in a tolerant rice mutant. Arch Environ Con Tox. 2009;56:723–731. doi:https://doi.org/10.1007/s00244-008-9226-2.
- Khan MIR, Jahan B, AlAjmi MF, Rehman MT, Khan NA. Ethephon mitigates nickel stress by modulating antioxidant system, glyoxalase system and proline metabolism in Indian mustard. Physiol Mol Biol Pla. 2020;26:1201–1213. doi:https://doi.org/10.1007/s12298-020-00806-1.
- Bohnert HJ, Shen B. Transformation and compatible solutes. Sci Hortic-Amsterdam. 1998;78:237–260. doi:https://doi.org/10.1016/S0304-4238(98)00195-2.
- Majumder AL, Johnson MD, Henry SA. 1L-myo-inositol-1-phosphate synthase. Biochim Biophys Acta. 1997;1348:245–256. doi:https://doi.org/10.1016/S0005-2760(97)00122-7.
- Li H, Wang H, Wen WJ, Yang GW. The antioxidant system in Suaeda salsa under salt stress. Plant Signal Behav. 2020;15:7. doi:https://doi.org/10.1080/15592324.2020.1771939.
- Zhou XT, Wang F, Ma YP, Jia LJ, Liu N, Wang HY, Zhao P, Xia GX, Zhong NQ. Ectopic expression of SsPETE2, a plastocyanin from Suaeda salsa, improves plant tolerance to oxidative stress. Plant Sci. 2018;268:1–10. doi:https://doi.org/10.1016/j.plantsci.2017.12.006.
- Wang BS, Lüttge U, Ratajczak R. Specific regulation of SOD isoforms by NaCl and osmotic stress in leaves of the C3 halophyte Suaeda salsa L. J Plant Physiol. 2004;161:285–293. doi:https://doi.org/10.1078/0176-1617-01123.
- Wang F, Zhong NQ, Gao P, Wang GL, Wang HY, Xia GX. SsTypA1, a chloroplast-specific TypA/BipA-type GTPase from the halophytic plant Suaeda salsa, plays a role in oxidative stress tolerance. Plant Cell Environ. 2008;31:982–994. doi:https://doi.org/10.1111/j.1365-3040.2008.01810.x.
- Qi YC, Zhang SM, Wang LP, Wang MD, Zhang H. Overexpression of GST gene accelerates the growth of transgenic Arabidopsis under salt stress. J Plant Physiol Mol Biol. 2004;30:517–522. [ in Chinese].
- Ma CL, Wang PP, Cao ZY, Zhao YX, Zhang H. Cloning and differential gene expression of two catalases in Suaeda salsa in response to salt stress. Acta Botanica Sicina. 2003;45:93–97.
- Guo XL, Cao YR, Cao ZY, Zhao YX, Zhang H. Molecular cloning and characterization of a stress-induced peroxiredoxin Q gene in halophyte Suaeda salsa. Plant Sci. 2004;167:969–975. doi:https://doi.org/10.1016/j.plantsci.2004.05.004.
- Ma CL, Wang PP, Cao ZY, Zhao YX, Zhang H. cDNA cloning and gene expression of APX in Suaeda salsa in response to salt stress. J Plant Physiol Mol Biol. 2002;28:261–266. [ in Chinese].
- Shu WS, Liu W, Lan CY. Viola baoshanensis Shu, Liu et Lan, a new species of Violaceae from Hunan Province, China. Acta Sci Nat Univ Sunyatseni. 2003;42:118–119. [ in Chinese].
- Yi LP, Wang ZW. Effects of different types of halophytes on the concentration of cadmium in coastal saline soil. Acta Ecologica Sin. 2017;37:4656–4662. [ in Chinese].
- Jiang L, Wang L, Zhang K, Tian CY. Copper-induced similar changes in growth and physiological responses of plants grown from dimorphic seeds of Suaeda salsa. Pak J Bot. 2018;50:871–877. doi:https://doi.org/10.5897/AJB2014.14279.
- Li CF, Ge BM, Jiang SH, Tang BP. Review on remedial effect of Suaeda salsa on saline and polluted soils. Chin J Soil Sci. 2014;45:1014–1019. [ in Chinese].
- Xie QY, Cao SY, Zhao CY, Yi K, Dai F, Qin HF, Qin YX, Fang L. Study on the molecular mechanisms of Suaeda heteroptera in response to high salt stress. J Dalian Ocean Univ. 2019;34:160–167. [ in Chinese].
- Dong JZ, Yin CH, Wang HX, Wang QL, Wang XY, Tian CY. Comparison of two species of halophyte in removing and reducing soil salinity from saline soil. Xinjiang Agric Sci. 2014;51:124–128. [ in Chinese].
- Huang J, Tian CY, Bian WG, Yin HL, Ren J, Chen CX. Response of growth of four halophyte species in oil-contaminated soil. Arid Zone Res. 2014;31:100–104. [ in Chinese].
- Yang XE, Long XX, Ye HB, He ZL, Calvert DV, Stoffella PJ. Cadmium tolerance and hyperaccumulation in a new Zn-hyperaccumulating plant species (Sedum alfredii Hance). Plant Soil. 2004;259:181–189. doi:https://doi.org/10.1023/b:plso.0000020956.24027.f2.
- Sun RL, Zhou QX, Sun FH, Jin CX. Antioxidative defense and proline/phytochelatin accumulation in a newly discovered Cd-hyperaccumulator, Solanum nigrum L. Environ Exp Bot. 2007;60:468–476. doi:https://doi.org/10.1016/j.envexpbot.2007.01.004.
- Hu PJ, Zhou XY, Qiu RL, Tang YT, Ying RR. Cadmium tolerance and accumulation features of Zn-hyperaccumulator Potentilla griffithii var. velutina. J Agro Environ Sci. 2007;26:2221–2224. [ in Chinese].
- Peng HY, Yang XE, Tan SK. Accumulation and ultrastructural distribution of copper in Elsholtzia splendens. J Zhejiang Univ Sci. 2005;6:311–318.
- Liao B, Deng DM, Yang B, Shu WS, Lin L, Lan CY. Cu tolerance and accumulation in Commelina communis. Acta Scientiae Circumstantiae. 2003;6:797–801. doi:https://doi.org/10.13671/j.hjkxxb.2003.06.018. [in Chinese].
- Huang JW, Cunningham SD. Lead phytoextraction: species variation in lead uptake and translocation. New Phytol. 1996;134:75–84. doi:https://doi.org/10.1111/j.1469-8137.1996.tb01147.x.
- Wang HQ, Li H, Lu SJ. Bidens maximowicziana’s adsorption ability and remediation potential to lead in soils. Environ Sci. 2005;6:145–149. doi:https://doi.org/10.13227/j.hjkx.2005.06.030. [ in Chinese].
- Xue SG, Chen YX, Lin Q, Xu SY, Wang YP. Phytolacca acinosa Roxb. (Phytolaccaceae): a new manganese hyperaccumulator plant from southern China. Acta Ecologica Sin. 2003;5:935–937. [ in Chinese].
- Mizuno T, Asahina R, Hosono A, Tanaka A, Senoo K, Obata H. Age-dependent manganese hyperaccumulation in Chengiopanax sciadophylloides (Araliaceae). J Plant Nutr. 2008;31(10):1811–1819. doi:https://doi.org/10.1080/01904160802325396.
- Chen TB, Lei M, Wan XM, Yang J, Zhou XY. Arsenic hyperaccumulator Pteris vittata L. and its application to the field. In: Luo YM, Tu C, editors. Twenty years of research and development on soil pollution and remediation in China. Singapore: Springer; 2018. p. 465–476. doi:https://doi.org/10.1007/978-981-10-6029-8_27.
- Francesconi KA, Visoottiviseth P, Sridokchan W, Goessler W. Arsenic species in an arsenic hyperaccumulating fern, Pityrogramma calomelanos: a potential phytoremediator of arsenic-contaminated soils. Sci Total Environ. 2002;284(1–3):27–35. doi:https://doi.org/10.1016/s0048-9697(01)00854-3.
- Li XX, Zhang X, Wang XL, Yang XY, Cui ZJ. Bioaugmentation-assisted phytoremediation of lead and salinity co-contaminated soil by Suaeda salsa and Trichoderma asperellum. Chemosphere. 2019;224:716–725. doi:https://doi.org/10.1016/j.chemosphere.2019.02.184.
- Rascio N, Navari-Izzo F. Heavy metal hyperaccumulating plants: how and why do they do it? and what makes them so interesting? Plant Sci. 2011;180:169–181. doi:https://doi.org/10.1016/j.plantsci.2010.08.016.