ABSTRACT
Plant architecture and disease resistance are the key factors that control the production of yield. However, the mechanism behind these factors is largely unknown. In this study, we identified that indeterminate domain 3 (IDD3) was obviously induced by inoculation of Rhizoctonia solani AG1-IA. Plants that overexpressed IDD3 (IDD3 OX) were more susceptible, while idd3 mutants showed a similar response to sheath blight disease compared with wild-type plants. Interestingly, IDD3 OX plants developed a wider tiller angle and exhibited altered shoot gravitropism, while idd3 knock-out mutants showed no visible morphological differences compared with the wild-type plants. IDD3 is ubiquitously expressed in different tissues and stages, and the IDD3 transcript was induced by exogenously applied auxin. Expression of the PIN-FORMED (PIN) and Aux/IAA genes was altered in IDD3 OX compared with wild-type plants. Furthermore, IDD3 OX plants are sensitive to auxin and the polar auxin transporter inhibitor N-1-naphthylphalamic acid (NPA). Further yeast-one hybrid, chromatin immunoprecipitation (ChIP) and transient assays revealed that IDD3 directly represses PIN1b via promoter binding. Inoculation with R. solani indicated that PIN1b RNAi plants are more susceptible to sheath blight disease (ShB) compared with the wild-type. Taken together, our analyses suggest that IDD3 controls plant architecture and the resistance of rice to ShB via the regulation of PIN auxin transporter genes.
Introduction
Rice (Oryza sativa) is a cereal grain that is the most widely consumed staple food for a large part of the world’s human population; therefore, higher yield potential and yield stability are needed to meet the challenges of increasing demand for its production.Citation1 Alternatively, with the swift decrease in arable land area that follows quick economic development, food security has become a serious problem. Developing ideal plant architecture varieties that produce substantially more grain has become a critical endeavor for scientists. One of the important goals of crop breeding is yield improvement. Among the yield indices, tiller angle is tightly associated with the enhancement of photosynthetic efficiency and facilitation of enhanced planting density.Citation2,Citation3 Rice plants with erect tillers, leaves and panicles on high-density planting systems should be optimal for high yield. However, these factors will increase the occurrence of sheath blight disease (ShB) caused by Rhizoctonia solani that results in a reduction in yield. Therefore, the antagonistic relationship between crop yield and pathways of plant resistance renders crop breeding even more difficult.Citation4
Extensive studies have focused on the isolation of tiller angle regulators. Polar auxin transport was one of the aspects that was identified for its importance in tiller angle control. The suppression of PIN-FORMED1 (PIN1) or overexpression of PIN2, two auxin efflux transporters that alter auxin transport activity, lead to increased tiller angles in rice.Citation5,Citation6 Lazy1 plays a negative role in polar auxin transport, and the loss of Lazy1 function impaired endogenous auxin distribution, resulting in reduced shoot gravitropism and a tiller-spreading phenotype.Citation7 In addition, quantitative trait loci (QTLs) related to tiller angle have been identified.Citation8 Mutation at a major quantitative trait locus, TAC1 (Tiller Angle Control 1), narrows tiller angle, resulting in more efficient plant architecture.Citation9 The PROG1 (PROSTRATE GROWTH 1) gene controls a key transition from prostrate to erect growth in the domestication of rice.Citation10 ShB is one of the three major diseases that are caused by R. solani in rice.Citation11 ShB can reduce rice yield production up to 50% when the disease is severe.Citation12 In addition, our recent studies observed that lazy1 mutants promote the disease,Citation13 while pin1a mutants reduce the resistance of rice to ShB,Citation14 respectively, suggesting that a complex regulatory mechanism occurs between plant architecture and defense regulation.
The indeterminate domain (IDD) consists of two C2H2 and two C2HC zinc finger motifs, and the IDD genes have diverse biological functions in plants. Extensive studies were performed to analyze IDD protein functions in plants; ID1 mutants showed delay of flowering time in maize and rice;Citation15,Citation16 Magpie (MAG)/AtIDD3 and Jackdaw (JKD)/AtIDD10 regulate the fate of root cells;Citation17 Enhydrous (ENY)/AtIDD1 regulates seed maturation;Citation18 AtIDD8 modulates plant development and sugar metabolism;Citation19 AtIDD14, AtIDD15 and AtIDD16 cooperatively regulate lateral organ morphogenesis and gravitropism by promoting auxin biosynthesis and transport in Arabidopsis;Citation20 Loose plant architecture1 (LPA1)/IDD14 regulates shoot gravitropism and the lamina joint angle;Citation21,Citation22 the regulator of CBF1 (ROC1)/IDD3 activates DREB1B/CBF1 to regulate chilling tolerance in rice;Citation23 IDD2 regulates secondary cell wall formation in rice,Citation24 suggesting that IDDs play the key roles in diverse aspect of plant biological processes. In addition, the AtIDD4 repressor was reported to constitutively induce immunity in Arabidopsis,Citation25 and LPA1 promotes the resistance of rice to ShB via activation of PIN1a,Citation14 suggesting that IDDs also function in plant defense. In addition, the binding motifs of the transcription factor IDD have been identified in maize (ID1, 5ʹ-TTTGTCG/CTTTT-3ʹ), Arabidopsis (AtIDD8, 5ʹ-TTTTGTCC-3ʹ), and rice (IDD10, 5ʹ-TTTGTCC/G);Citation19,Citation26,Citation27 however, IDD targets and the regulatory mechanism in regulation of plant architecture and defense are largely unknown.
In this study, we elucidated that IDD3 expression and sensitivity to ShB, along with further genetic and molecular study, showed that plants that overexpress IDD3 modulate the resistance of rice to ShB, as well as changing the tiller angle. Further analyses identified that IDD3 is involved in auxin signaling and regulates the expression of PIN and AUX/IAA genes, which could enable IDD3 to regulate the tiller angle and rice defense.
Materials and methods
Mutant isolation and plant growth
T-DNA insertion lines idd3-1 (PFG_3A-09378) and idd3-2 (PFG_3A-14411) were isolated from a rice T-DNA population (http://signal.salk.edu/cgi-bin/RiceGE/).Citation28 The mutant lines were derived from the Japonica rice cultivar ‘Dongjin.’ Transgenic lines were generated from this rice cultivar. Experiments were conducted involving treatment with auxin, a brassinosteroid (BR) and abscisic acid (ABA). Seeds were germinated and grown for 7 days on liquid half-strength Morishige and Skoog (MS) medium, transferred to the same medium containing 1 µM 2,4-D (synthetic auxin), 0.1 µM 2,4-epiBL (BR) and 1 µM ABA for 3 and 6 h, and whole seedlings were sampled for RNA extraction. Seedling growth tests were conducted to determine the dependence of seedling growth on auxin using 2,4-D, NAA and the polar auxin transporter inhibitor NPA. Rice seeds were germinated on half-strength MS media containing auxin (0.5 and 1 µM NPA; 10 and 100 nM 2,4-D; 10 and 100 nM NAA) after being de-husked and surface sterilized. Five-day-old plants were photographed.
Plant growth and inoculation with R. solani AG1-IA
The wild-type (WT) control line (O. sativa Japonica, cultivar Dongjin), idd3-1 (PFG_3A-09378), idd3-2 (PFG_3A-14411) and IDD3-GFP overexpressor (IDD3 OX) plants were used. The plants were grown in a greenhouse at Shenyang Agricultural University, Shenyang, China, at a temperature of 23ºC–30°C. One-month-old rice plants were inoculated with R. solani AG1-IA.Citation29
Plasmid construction and cytological observation
To generate the IDD3-GFP overexpression transgenic plants, IDD3 ORF sequences were amplified and cloned into BglII and SpeI restriction enzyme sites of the pCAMBIA1302 binary vector, in which IDD3 coding sequences were N-terminally fused to the GFP coding sequences. The 3 kb IDD3 promoter region was fused to GUS in PCAMBIA1381 vector to create the GUS-fusion vector. A total of 300 bp of PIN1b cDNA sequences were fused separately from the GUS intron sequences in sense and antisense directions, respectively, and the construct was cloned into the BamHI and HindIII restriction enzyme sites of the pGA1611 binary vector. The following primers were used for cloning IDD3 ORF and its promoter or PIN1b cDNA: IDD3 cDNA-F: GATTCATACAAGCTTATGGCGGCCGCCTCGTCCGCACCCTTC, IDD3 cDNA-R: AGATCTGCGGCGGCCATGTTTGCCGGGTCCAGTGAGCCGAC, IDD3 PM-F: GAATTCAGGTTTGCTGTCTCCCTTTC, IDD3 PM-R: GAGCTCTCTCGCTGCTTACTTTGTTG, PIN1b C-F: ATGGCGCTGCAGCCGCGGATCATC, PIN1b C-R: GATGTAGTACACCAGCGTGATGGGCAGCG. Rice transformation and GUS detection were performed using standard methods.Citation28
RT-PCR analysis
Total cellular RNA was isolated using RNeasy Plant Mini Kits (Qiagen, http://www.qiagen.com/). The cDNA was synthesized using reverse transcriptase RNaseH (Toyobo, http://www.toyobo.co.jp/e/). qRT-PCR products were quantified using Illumina Research Quantity software (Eco 3.0) (Illumina, San Diego, CA, USA), and values were normalized against Actin and Ubiquitin cDNA from the same samples. The following primers were used for RT-PCR or qRT-PCR are shown in bellow: IDD3 RT-F: ACCGGGATCAAGAAGCACTACTG, IDD3 RT-R: GATCAAACTGAGAGGCGCCATTG, IAA3-F: GGGTTCTCCAAGACATGCAATC, IAA3-R: GGATGGAAATCAAAGCATTGAAGC, PIN1a-F: TCATCTGGTCGCTCGTCTGC, PIN1a-R: CGAACGTCGCCACCTTGTTC, PIN1b-F: TGCACCCTAGCATTCTCAGCA, PIN1b-R: CCCTCCTCCCAAATTCTACTT C, PIN2-F: CAGGGCTAGGAATGGCTATGT, PIN2-R: GCAAACACA AACGGGACAA, TAC1 F: GAGATGGCTCTAAAGGTGTTC, TAC1 R: CGTGCCAATTGCAAGTATACC, Lazy1 F: ACGGTGACGAAGAGCAAGTT, Lazy1 R: ACAGCACATTCAAGCCCTTC, Ubiquitin-F: GCACAAGCACAAGAAGGTGA, Ubiquitin-R: GCCTGCTGGTTGTAGACGTA, Actin-F: CTTCATAGGAATGGAAGCTGCGGGTA, Actin-R: CGACCACCTTGATCTTCATGCTGCTA.
Northern blot analysis
Formaldehyde gels (1.3%) were prepared in MOPS/EDTA buffer (0.5 M MOPS, pH 7.0; 0.01 M Na2EDTA, pH 7.5) to use for Northern hybridizations. Twenty micrograms of each RNA sample were dissolved and heat-denatured in formaldehyde/formamide buffer. After electrophoresis, the gels were washed with water and 10× SSC and blotted onto a Hybond N+ membrane (Amersham Pharmacia Biotech, Little Chalfont, UK) prewetted in 10× SSC. rRNA was stained with ethidium bromide (EtBr) and used as a loading control. Hybridization was performed at 65°C in Church buffer (1% bovine serum albumin [BSA], 200 µM EDTA, 0.5 M sodium phosphate, and 7% SDS) containing a 32P-labeled probe. The membranes were autoradiographed using Fuji X-ray film. An IDD3 cDNA fragment (637–1608 bp) was used as a probe.
Gravitropism assay
The gravity response was analyzed as previously described.Citation7 Rice seeds were grown on half-strength liquid Morishige and Skoog (MS) medium after surface sterilization. Six-day-old plants were placed horizontally under 16 h dim-light/8 h dark at 26°C to examine the gravitropic responses. The gravity response was determined by measuring the curved angle after reorientation by 90°.
Yeast one-hybrid analysis
Yeast-one hybrid assays were performed using a 1.5-kb section of the PIN1b promoter that was amplified by PCR and cloned into a pLacZi vector. Screening of the interaction clones was conducted via mating using Matchmaker Gold Systems (Clontech, Takara Bio USA, USA). The ORF sequences of IDD3 were cloned into the pGAD424 vector. The constructed pGAD424-IDD3 or empty vector pGAD424 were transformed into the yeast one-hybrid bait strain (YM4271), and the growth of the yeast cells was monitored on synthetic dropout media lacking Leu and Ura. The cells were transferred to 3 M paper and incubated with X-gal at 37°C for a chromogenic reaction. The following primers were used to cloning PIN1b promoter: PIN1b PF: GAATTC GAATAGGTCAACTCATTCC, PIN1b PR: CTTGCAGCCAAGAAAAACCACGACTTC.
Chromatin-immunoprecipitation (ChIP) assay
Eight grams of rice calli were collected from transgenic plants that expressed 35S:IDD3-GFP for the ChIP assay. The ChIP assay and subsequent ChIP-PCR assays were performed as previously described.Citation30 The primers used for the ChIP-PCR are shown in bellow: P1 F: AAAATATTGATTAAAGGGTG, P1 R: CTCACTAAGAATATCACCAGC, P2 F: AGAAGATGTTTGGTTGATGC, P2 R: TAATTTTACCTGATCGAAAG.
Transient expression assay
The effector plasmid (35S:IDD3) and reporter (pPIN1b or mutated promoter, mpPIN1b), as well as an internal control plasmid (35S:LUC), were co-transformed into protoplast cells for the transient expression assay.Citation31 The GUS activity analyses were performed as previously described.Citation27 The luciferase assay was performed using a Luciferase Assay Kit (Promega Bio Sciences, LLC, San Luis Obispo, CA, USA), and PEG-mediated transformation and luciferase activity assays were performed as previously described.Citation32 The primers used for the transient assay are shown in bellow: PIN1b PF: GAATTC GAATAGGTCAACTCATTCC, PIN1b PR: CTTGCAGCCAAGAAAAACCACGACTTC, mP-F: ATTCCAAAAAAATTCAAACC, mP-R: GGTTTGAATTTTTTTGGAAT.
Statistical analysis
Statistical calculations were performed using Prism 5 (GraphPad, San Diego, CA, USA). All data were expressed as the mean ± SE. Comparisons between the groups were performed using a one way analysis of variance (ANOVA) and at least P > .05 was considered as a significant difference.
Results
Patterns of expression of IDD3
To test whether the expression of IDD3 responds to ShB, qRT-PCR was performed using sheaths inoculated with R. solani. The results showed that IDD3 was obviously induced after 24 and 72 hours of inoculation, while it was suppressed after 48 hours of inoculation ()). Transgenic plants in which GUS was driven by a 3.0 kb endogenous IDD3 promoter were generated to confirm the patterns of expression of IDD3 in plants. GUS expression was obviously induced upon R. solani inoculation in the sheath ()). In addition, the patterns of expression of IDD3 were analyzed in leaves, roots, sheath, shoot apices and flowers. The results indicated that IDD3 was detected in all the tissues, and the lowest level of expression was detected in flowers ()).
Figure 1. Patterns of expression of IDD3 in rice
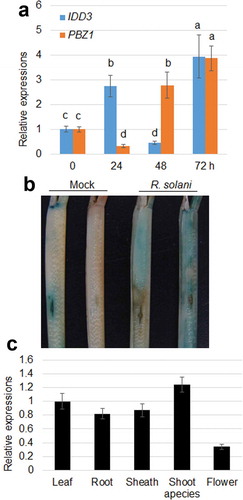
IDD3 overexpressors exhibit an enlarged tiller angle and reduced resistance to ShB
To elucidate the function of IDD3 in the resistance of rice to ShB, two independent mutants were isolated from a T-DNA population using PCR and target-specific primers. The T-DNAs were inserted into the second intron of the IDD3 locus. RT-PCR showed a lack of IDD3 transcripts in the IDD3 mutants (idd3-1 and idd3-2) ()). In parallel, IDD3 overexpressors (OXs) were generated. The levels of expression of IDD3 are significantly higher in OX lines than in the wild-type ()). idd3 mutants did not exhibit any visible morphological differences, but IDD3 OXs displayed increased tiller angles compared with the wild-type plants, and the tiller angles corresponded with the levels of expression of IDD3 in OX lines ()).
Figure 2. Phenotypic expression of IDD3 mutants and overexpression plants
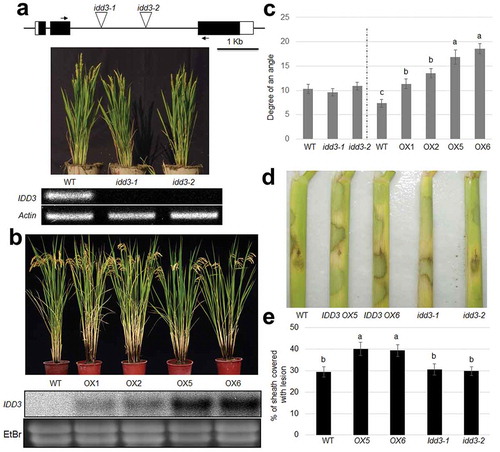
The results of inoculation with R. solani showed that the idd3 mutants exhibited a response to ShB similar to that of the wild-type, while IDD3 OX exhibited enhanced susceptibility to infection with R. solani compared with the wild-type plants ()). The percentage of the sheath area covered with lesions was 29.3% in WT, 30.3% in idd3-1, 29.5% in idd3-2, 39.2% in IDD3 OX #5 and 39.1% in IDD3 OX #6 plants ()).
Overexpression of IDD3 resulted in an altered response to auxin
Since IDD3 OX lines exhibited an enlarged tiller angle, the 2.0 kb promoter of IDD3 was analyzed via the PLACE database. Interestingly, one AuRE (auxin-responsive element), four E-box (BR signal transcription factor RAVL1 binding motif), and two ABRE (ABA-responsive element) appeared within a 2.0 kb promoter ()). In addition, phytohormone-mediated patterns of expression of IDD3 were examined in more detail. After treatment with 2,4-D (an auxin mimic), 2,4-epiBL (BR) and ABA, IDD3 was specifically induced by 2,4-D but not by 2,4-epiBL and ABA after 3 and 6 hours of accumulation ()). Additionally, the levels of expression of the IAA3 and IDD3 genes were sensitive to auxin treatment in a time-dependent manner (,)).
Figure 3. Auxin-dependent levels of IDD3 and IDD3 overexpression phenotype
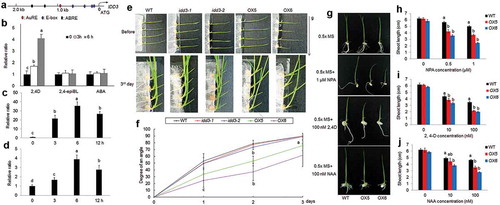
Tiller angle changes have been explained as effects of gravitropism.Citation5 Therefore, the shoot gravity responses between wild-type, idd3 mutants and IDD3 OX plants were compared. Five-day-old wild-type, idd3 mutants and IDD3 OX plants were gravistimulated by horizontal placement, and their responses to gravity were measured for up to 3 days ()). The curvature of wild-type and idd3 mutants reached approximately 90° on day 3. However, IDD3 ox plants exhibited weak gravitropism compared with the wild-type and mutant plants, and the curvature reached approximately 60–70° after 5 days of gravistimulation ()).
Auxin-dependent plant growth was analyzed in more detail using wild-type and IDD3 OX plants. 2,4-D, NAA and NPA were used to analyze the response of each line. The results showed that IDD3 OX seedlings exhibited a growth pattern similar to that of the wild-type plants. A high concentration of exogenously supplied NPA, 2,4-D and NAA inhibited the growth of shoots of wild-type plants, and two independent IDD3 OX lines (OX5 and OX6) exhibited a sensitive response to NPA, 2,4-D and NAA compared with the wild-type plants ().
IDD3 directly represses PIN1b
PIN-FORMED (PIN), IAA3 and Lazy1 are involved in auxin transport or auxin response, as well as in the control of tiller angle and gravitropism. TAC1 serves as a QTL locus control for tiller angles in rice.Citation9 The expression of PIN1a, PIN1b, PIN2, IAA3, Lazy1 and TAC1 was compared in wild-type and IDD3 OX plants. Two-week-old wild-type and two independent OX plants (OX5 and OX6) were used for qRT-PCR assays. The levels of PIN1a and PIN1b are ~25% and ~75% lower, while those of PIN2 and IAA3 are ~70% higher in OX lines than in the wild-type plants ()). However, no significant differences in the expression of Lazy1 and TAC1 were observed ()). Analysis of the promoter sequence identified that one IDD-binding motif appeared within 1.5 kb of PIN1b promoter ()). The results of this binding assay were confirmed using a yeast-one hybrid assay, which indicated that IDD3 can bind to the putative IDD-binding motif within the 1.5 kb PIN1b promoter ()). The ChIP assay using 35S:IDD3:GFP transgenic plant calli showed that the precipitation of IDD3 enriched the P2 region, but not the P1 region, of the PIN1b promoter ()). To verify if these cis-elements were responsible for the transcriptional activation of the PIN1b promoter via IDD3 in vivo, we performed a transactivation assay by transient expression in Arabidopsis thaliana protoplasts. The results suggested that IDD3 transrepresses the P2 promoter, but not the IDD-binding motif mutated promoter, mP2 ()), indicating that IDD3 could repress the expression of PIN1b via promoter binding.
Figure 4. IDD3 directly regulates the expression of PIN1b.
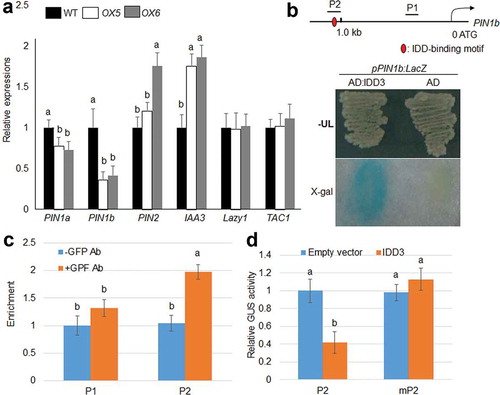
PIN1b positively regulates the resistance of rice to ShB
RNAi plants were generated to analyze the function of PIN1b in defense of rice to sheath blight. qRT-PCR confirmed the levels of expression of PIN1b in wild-type and PIN1b RNAi lines (#1-#4). The results indicated that the level of expression of PIN1b was significantly lower in PIN1b RNAi lines than in the wild-type plants ()). The results from inoculation with R. solani showed that the PIN1b RNAi lines were more susceptible to infection with R. solani than the wild-type plants ()). The percentage of the sheath area covered with lesions was 30.8% in WT, 40.8% in PIN1b RNAi #1, 39.3% in PIN1b RNAi #2, 40.2% in PIN1b RNAi #3, and 38.7% in PIN1b RNAi #4 plants ()).
Figure 5. The response of PIN1b RNAi plants to sheath blight
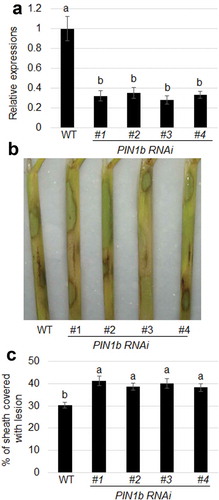
Discussion
Sheath blight disease caused by R. solani is a major disease of rice and severely reduces grain yield. However, the resistance mechanisms of the host remain elusive. Previously, we elucidated that LPA1/IDD14 promoted the defense of rice against ShB via the activation of PIN1a,Citation14 and interestingly, we identified the additional IDD member IDD3 that was significantly induced by R. solani infection, which was confirmed by qRT-PCR and an examination of the pattern of GUS expression in a transgenic plant. Inoculation with R. solani revealed that the two idd3 mutant lines did not differ significantly from the wild-type plants in their response to ShB. To further verify IDD3 function, IDD3 overexpressors were generated, and their response to ShB was analyzed to provide additional evidence. The results indicated that IDD3 overexpressors are more susceptible to ShB compared with wild-type plants. These results suggest that IDD3 negatively regulates rice defense to ShB.
Tiller angle is one of the traits that significantly contributes to plant architecture. A larger tiller angle results in lower humidity and can reduce the incidence of disease. However, it also consumes too much space and causes shading by reducing the time available to capture light. In contrast, erect plants increase photosynthesis and planting number, but they are more susceptible to insects and pathogens.Citation6 Therefore, an exploration of the regulatory mechanism that underlies tiller development and disease resistance is important for rice breeding. The use, distribution and transport of auxin play an important role in the alteration of plant architecture.Citation33,Citation34 PIN1a and PIN2 are members of the PIN-FORMED family of genes, and the suppression of PIN1a and overexpression of PIN2 lead to increased tiller angles in rice.Citation5,Citation6 The IDD3 promoter contains AuRE, E-box and ABRE motifs, but the IDD3 transcript is only regulated by auxin. It is possible that IDD3 is one of the auxin signaling proteins that is involved in the developmental regulation of plants mediated by auxin. The overexpression of IDD3 in transgenic rice plants showed that IDD3 OX greatly increased tiller angles. Furthermore, the levels of expression of PIN1a and PIN1b were lower, while that of PIN2 was higher in IDD3 OX than in wild-type plants, implying that IDD3 OX may increase the tiller angle via regulation of the expression of PIN1a and PIN2. The overexpression of IDD3 increased the tiller angle, but idd3 knock-out mutants exhibited normal tiller growth. In A. thaliana, IDD14, 15 and 16 cooperatively regulate organ development and gravitropism by the direct regulation of TAA1, YUCCA5 and PIN1 genes,Citation20 which provided strong evidence that IDD proteins act as a complex on certain types of gene regulation. Further genetic studies are required to understand the role of IDDs in tiller development. The overexpression of IDD3 affects shoot gravitropism, which is a response similar to those of the LPA1/IDD14 mutants.Citation22 Our study identified the role of IDD3 on the regulation of polar auxin transporters that control the tiller angle of rice and gravitropism, suggesting that IDD3 might control polar auxin transport to modulate plant architecture.
In addition, PIN1b was suppressed in IDD3 OX plants, and ChIP and yeast-one hybrid assays confirmed that IDD3 directly bound to the PIN1b promoter. The suppression of PIN1b also increased the susceptibility of rice plants to ShB. Recently, we also identified the function of IDD3 in directly repressing PIN1a to regulate resistance to ShB,Citation35 suggesting that IDD3 might function via the repression of both PIN1a and PIN1b to modulate resistance to ShB. The suppression of PIN1a and overexpression of PIN2 enlarged the tiller angle,Citation5,Citation6 suggesting that IDD3 might control the expression of multiple PIN genes to regulate tiller angle development and the resistance of rice to ShB. In conclusion, our analyses indicated that IDD3 functions as a transcriptional repressor to regulate the expression of PIN genes to modulate auxin distribution, which significantly contributes to the modulation of resistance to ShB in rice.
Disclosure of Potential Conflicts of Interest
The authors declare no conflict of interest.
Additional information
Funding
References
- Khush GS. Origin, dispersal, cultivation and variation of rice. Plant Mol Biol. 1997;35(1–2):1–8.. doi:https://doi.org/10.1023/A:1005810616885.
- Wang Y, Li J. Rice, rising. Nat Genet. 2008;40(11):1273–1275.doi:https://doi.org/10.1038/ng1108-1273.
- Sakamoto T, Morinaka Y, Ohnishi T, Sunohara H, Fujioka S, Ueguchi-Tanaka M, Mizutani M, Sakata K, Takatsuto S, Yoshida S, et al. Erect leaves caused by brassinosteroid deficiency increase biomass production and grain yield in rice. Nat Biotechnol. 2006;24(1):105–109. doi:https://doi.org/10.1038/nbt1173.
- Ning Y, Liu W, Wang GL. Balancing immunity and yield in crop plants. Trends Plant Sci. 2017;22(12):1069–1079. doi:https://doi.org/10.1016/j.tplants.2017.09.010.
- Chen Y, Fan X, Song W, Zhang Y, Xu G. Over-expression of OsPIN2 leads to increased tiller numbers, angle and shorter plant height through suppression of OsLAZY1. Plant Biotechnol J. 2012;10(2):139–149. doi:https://doi.org/10.1111/j.1467-7652.2011.00637.x.
- Xu M, Zhu L, Shou H, Wu P. A PIN1 family gene, OsPIN1, involved in auxin-dependent adventitious root emergence and tillering in rice. Plant Cell Physiol. 2005;46(10):1674–1681.doi:https://doi.org/10.1093/pcp/pci183.
- Li P, Wang Y, Qian Q, Fu Z, Wang M, Zeng D, Li B, Wang X, Li J. LAZY1 controls rice shoot gravitropism through regulating polar auxin transport. Cell Res. 2007;17(5):402–410. doi:https://doi.org/10.1038/cr.2007.38.
- Li ZKPA, Pinson SRM, Stansel JW. RFLP facilitated analysis of tiller and leaf angles in rice (Oryza sativa L.). Euphytica. 1999;109:79–84. doi:https://doi.org/10.1023/A:1003533001014.
- Yu B, Lin Z, Li H, Li X, Li J, Wang Y, Zhang X, Zhu Z, Zhai W, Wang X, et al. TAC1, a major quantitative trait locus controlling tiller angle in rice. Plant J. 2007;52(5):891–898. doi:https://doi.org/10.1111/j.1365-313X.2007.03284.x.
- Tan L, Li X, Liu F, Sun X, Li C, Zhu Z, Fu Y, Cai H, Wang X, Xie D, et al. Control of a key transition from prostrate to erect growth in rice domestication. Nat Genet. 2008;40(11):1360–1364. doi:https://doi.org/10.1038/ng.197.
- Savary S, Castilla NP, Elazegui FA, McLaren CG, Ynalvez MA, Teng PS. Direct and indirect effects of nitrogen supply and disease source structure on rice sheath blight spread. Phytopathology. 1995;85:959–965. doi:https://doi.org/10.1094/Phyto-85-959.
- Savary S, Willocquet L, Elazegui FA, Castilla NP, Teng PS. Rice pest constraints in Tropical Asia: quantification of yield losses due to rice pests in a range of production situations. Plant Dis. 2000;84(3):357–369.doi:https://doi.org/10.1094/pdis.2000.84.3.357.
- Sun Q, Liu Y, Wang ZY, Li S, Ye L, Xie JX, Zhao GQ, Wang HN, Wang Y, Li S, et al. Isolation and characterization of genes related to sheath blight resistance via the tagging of mutants in rice. Plant Gene. 2019;19:10020. doi:https://doi.org/10.1016/j.plgene.2019.100200.
- Sun Q, Li TY, Li DD, Wang ZY, Li S, Li DP, Han X, Liu JM, Xuan YH. Overexpression of loose plant architecture 1 increases planting density and resistance to sheath blight disease via activation of PIN-FORMED 1a in rice. Plant Biotechnol J. 2019;17(5):855–857. doi:https://doi.org/10.1111/pbi.13072.
- Colasanti J, Yuan Z, Sundaresan V. The indeterminate gene encodes a zinc finger protein and regulates a leaf-generated signal required for the transition to flowering in maize. Cell. 1998;93(4):593–603. doi:https://doi.org/10.1016/S0092-8674(00)81188-5.
- Park SJ, Kim SL, Lee S, Je BI, Piao HL, Park SH, Kim CM, Ryu C-H, Park SH, Xuan Y-H, et al. Rice Indeterminate 1 (OsId1) is necessary for the expression of Ehd1 (Early heading date 1) regardless of photoperiod. Plant J. 2008;56(6):1018–1029. doi:https://doi.org/10.1111/j.1365-313X.2008.03667.x.
- Welch D, Hassan H, Blilou I, Immink R, Heidstra R, Scheres B. Arabidopsis JACKDAW and MAGPIE zinc finger proteins delimit asymmetric cell division and stabilize tissue boundaries by restricting SHORT-ROOT action. Genes Dev. 2007;21(17):2196–2204. doi:https://doi.org/10.1101/gad.440307.
- Feurtado JA, Huang D, Wicki-Stordeur L, Hemstock LE, Potentier MS, Tsang EW, Cutler AJ. The Arabidopsis C2H2 zinc finger INDETERMINATE DOMAIN1/ENHYDROUS promotes the transition to germination by regulating light and hormonal signaling during seed maturation. Plant Cell. 2011;23(5):1772–1794.doi:https://doi.org/10.1105/tpc.111.085134.
- Seo PJ, Ryu J, Kang SK, Park CM. Modulation of sugar metabolism by an INDETERMINATE DOMAIN transcription factor contributes to photoperiodic flowering in Arabidopsis. Plant J. 2011;65(3):418–429. doi:https://doi.org/10.1111/j.1365-313X.2010.04432.x.
- Cui D, Zhao J, Jing Y, Fan M, Liu J, Wang Z, Xin W, Hu Y. The arabidopsis IDD14, IDD15, and IDD16 cooperatively regulate lateral organ morphogenesis and gravitropism by promoting auxin biosynthesis and transport. PLoS Genet. 2013;9(9):e1003759. doi:https://doi.org/10.1371/journal.pgen.1003759.
- Wu X, Tang D, Li M, Wang K, Cheng Z. Loose plant architecture1, an indeterminate domain protein involved in shoot gravitropism, regulates plant architecture in rice. Plant Physiol. 2013;161(1):317–329. doi:https://doi.org/10.1104/pp.112.208496.
- Liu JM, Park SJ, Huang J, Lee EJ, Xuan YH, Je BI, Kumar V, Priatama RA, Raj K V, Kim SH, et al. Loose Plant Architecture1 (LPA1) determines lamina joint bending by suppressing auxin signalling that interacts with C-22-hydroxylated and 6-deoxo brassinosteroids in rice. J Exp Bot. 2016;67(6):1883–1895. doi:https://doi.org/10.1093/jxb/erw002.
- Dou M, Cheng S, Zhao B, Xuan Y, Shao M. The indeterminate domain protein ROC1 regulates chilling tolerance via activation of DREB1B/CBF1 in rice. Int J Mol Sci. 2016;17(3):233.doi:https://doi.org/10.3390/ijms17030233.
- Huang P, Yoshida H, Yano K, Kinoshita S, Kawai K, Koketsu E, Hattori M, Takehara S, Huang J, Hirano K, et al. OsIDD2, a zinc finger and INDETERMINATE DOMAIN protein, regulates secondary cell wall formation. J Integr Plant Biol. 2018;60(2):130–143. doi:https://doi.org/10.1111/jipb.12557.
- Volz R, Kim SK, Mi J, Mariappan KG, Siodmak A, Al-Babili S, Hirt H. A chimeric IDD4 repressor constitutively induces immunity in Arabidopsis via the modulation of salicylic- and jasmonic acid homeostasis. Plant Cell Physiol. 2019;60:1536–1555. doi:https://doi.org/10.1093/pcp/pcz057.
- Kozaki A, Hake S, Colasanti J. The maize ID1 flowering time regulator is a zinc finger protein with novel DNA binding properties. Nucleic Acids Res. 2004;32(5):1710–1720. doi:https://doi.org/10.1093/nar/gkh337.
- Xuan YH, Priatama RA, Huang J, Je BI, Liu JM, Park SJ, Piao HL, Son DY, Lee JJ, Park SH, et al. Indeterminate domain 10 regulates ammonium-mediated gene expression in rice roots. New Phytol. 2013;197(3):791–804. doi:https://doi.org/10.1111/nph.12075.
- An S, Park S, Jeong DH, Lee DY, Kang HG, Yu JH, Hur J, Kim S-R, Kim Y-H, Lee M, et al. Generation and analysis of end sequence database for T-DNA tagging lines in rice. Plant Physiol. 2003;133(4):2040–2047. doi:https://doi.org/10.1104/pp.103.030478.
- Prasad B, Eizenga GC. Rice sheath blight disease resistance identified in oryza spp. accessions. Plant Dis. 2008;92(11):1503–1509. doi:https://doi.org/10.1094/pdis-92-11-1503.
- Je BI, Piao HL, Park SJ, Park SH, Kim CM, Xuan YH, Park SH, Huang J, Do Choi Y, An G, et al. RAV-Like1 maintains brassinosteroid homeostasis via the coordinated activation of BRI1 and biosynthetic genes in rice. Plant Cell. 2010;22(6):1777–1791. doi:https://doi.org/10.1105/tpc.109.069575.
- Yamaguchi M, Ohtani M, Mitsuda N, Kubo M, Ohme-Takagi M, Fukuda H, Demura T. VND-INTERACTING2, a NAC domain transcription factor, negatively regulates xylem vessel formation in Arabidopsis. Plant Cell. 2010;22(4):1249–1263. doi:https://doi.org/10.1105/tpc.108.064048.
- Yoo SD, Cho YH, Sheen J. Arabidopsis mesophyll protoplasts: a versatile cell system for transient gene expression analysis. Nat Protoc. 2007;2(7):1565–1572. doi:https://doi.org/10.1038/nprot.2007.199.
- Bennett MJ, Marchant A, Green HG, May ST, Ward SP, Millner PA, Walker AR, Schulz B, Feldmann KA. Arabidopsis AUX1 gene: a permease-like regulator of root gravitropism. Science (New York, NY). 1996;273(5277):948–950. doi:https://doi.org/10.1126/science.273.5277.948.
- Blilou I, Xu J, Wildwater M, Willemsen V, Paponov I, Friml J, Heidstra R, Aida M, Palme K, Scheres B, et al. The PIN auxin efflux facilitator network controls growth and patterning in Arabidopsis roots. Nature. 2005;433(7021):39–44.doi:https://doi.org/10.1038/nature03184.
- Sun Q, Li DD, Chu J, Yuan DP, Li S, Zhong LJ, Han X, Xuan YH. Indeterminate domain proteins regulate rice defense to sheath blight disease. Rice (New York, NY). 2020;13(1):15. Epub 2020/03/07. doi:https://doi.org/10.1186/s12284-020-0371-1.