ABSTRACT
Brassicaceae plants, including Arabidopsis thaliana, develop endoplasmic reticulum (ER)-derived structures called ER bodies, which are involved in chemical defense against herbivores. NAI1 is a basic helix-loop-helix (bHLH) type transcription factor that regulates two downstream genes, NAI2 and BGLU23, that are responsible for the ER body formation and function. Here, we examined the transcription factor function of NAI1, and found that NAI1 binds to the promoter region of NAI2 and activates the NAI2 promoter. The recombinant NAI1 protein recognizes the canonical and non-canonical G-box motifs in the NAI2 promoter. Furthermore, we examined the DNA binding activity of NAI1 toward several E-box motifs in the NAI2 and BGLU23 promoters and found that NAI1 binds to a DNA fragment that includes an E-box motif from the BGLU23 promoter. Subcellular localization of NAI1 was evident in the nucleus, which is consistent with its transcription factor function. Transient expression experiments in Nicotiana benthamiana leaves showed that GFP-NAI1 protein activated the NAI2 promoter by binding to the two G-boxes of the promoter. Disruption of the G-boxes abolished the NAI1-dependent activation of the NAI2 promoter. These results indicate that NAI1 has a DNA binding activity in a motif-dependent manner and suggest that NAI1 regulates NAI2 and BGLU23 gene expressions through binding to these DNA motifs in their promoters.
KEYWORDS:
Brassicaceae plants such as Arabidopsis thaliana produce an endoplasmic reticulum (ER) derived organelle called the ER body.Citation1–3 ER bodies specifically accumulate β-glucosidases (BGLUs) that possess an ER-retention signal.Citation4 In Arabidopsis, roots and epidermal cells of seedlings constitutively accumulate a high number of ER bodies.Citation2 The giant epidermal cells of leaves also accumulate ER bodies constitutively,Citation5,Citation6 but wounding or the wounding hormone jasmonate further induces the accumulation of ER bodies throughout entire leaves.Citation7,Citation8 We have recently found that ER bodies in seedlings and leaves are responsible for the chemical defense against herbivory with Brassicaceae specific glucosides, namely glucosinolates.Citation6,Citation9 The BGLUs in ER bodies hydrolyze glucosinolates to produce pungent repellents; ER bodies represent a defense system through an intracellular compartmentalization of glucosinolates and BGLUs.Citation6,Citation9–12
The Arabidopsis nai1 mutant does not accumulate ER bodies in seedlings and roots.Citation13 NAI1/bHLH020 encodes a basic helix-loop-helix (bHLH) type transcription factor and regulates the expression of genes encoding proteins that are important for ER body formation and function, such as NAI2 and PYK10/BGLU23.Citation4,Citation13,Citation14 NAI2 encodes a unique ER body protein that is responsible for the formation of ER bodies.Citation14 Brassicaceae plants have a NAI2-paralogous TSA1 gene, which expression is activated by jasmonate treatment.Citation7 This suggests that TSA1 is involved in the formation of ER bodies in leaves under stressed conditions.Citation7,Citation15
Since NAI1 is the central regulatory factor for the ER body-related genes, its molecular function is a great interest. bHLH proteins were first identified as transcription factors that bind a variation of an E-box element (CANNTG) in regulatory regions.Citation16,Citation17 Plant bHLH transcription factors regulate developmental processes, the response to environmental cues, and modulation of secondary metabolisms.Citation18–22 Most plant bHLH proteins have evolved from group B of the bHLH proteins, whose typical DNA binding sequence has been shown as a G-box (CACGTG) element.Citation23 In Arabidopsis, bHLH proteins are divided into 12 subfamilies and NAI1 is classified as type IVa along with three other homologs (bHLH018, bHLH019, and bHLH025).Citation24 The NAI1 homologs, namely bHLH proteins of the type IVa subfamily, are characterized in Catharanthus roseus (BIS1 and BIS2),Citation25,Citation26 Medicago truncatula (TSAR1 and TSAR2),Citation27 and Glycyrrhiza uralensis (GubHLH3).Citation28 Although these plants do not produce ER bodies these NAI1 homologs are involved in the regulation of terpene-derived metabolites that have a potential defense function in these species.Citation25–28 It has been shown that TSAR1 and TSAR2 recognize the G-box motif.Citation27 Furthermore, a DNA affinity purification sequence (DAP-seq) analysis revealed that a NAI1 homolog in Arabidopsis, bHLH018, binds to a consensus sequence including the G-box.Citation29 The data suggest a strong possibility that NAI1 binds to the G-box motif, but there was no direct evidence.
To address the DNA binding activity of NAI1, we expressed the NAI1 protein as a thioredoxin (TRX)-His-tag fusion protein in Escherichia coli and purified it with Ni2+-affinity chromatography. The molecular mass of the purified protein was a bit higher than expected in comparison to the theoretical value predicted for the recombinant protein, which was around 65 kDa ()). Recently we found that NAI1 has the potential to recognize canonical (CACGTG) and non-canonical (CACGTT) G-boxes in the NAI2 promoter.Citation7 Therefore, we examined its ability to bind to canonical and non-canonical G-boxes (G1 and G2) in the NAI2 promoter ()). We generated a biotinylated DNA probe including G1 and G2 (pNAI2 probe, )) and subjected it to electrophoretic mobility shift assay (EMSA). Protein-DNA complexes were detected when the TRX-NAI1 fusion proteins were incubated with the pNAI2 probe (). Excess unlabeled pNAI2-G1/G2 was able to compete for the DNA binding activity of NAI1 ()). The possibility of nonspecific binding of the TRX protein was eliminated as no shifted bands were observed when the TRX protein was incubated with the probe ()). These results indicate the specific interaction between NAI1 and the pNAI2 probe. To elaborate the DNA binding of NAI1 in a sequence-specific manner, we evaluated the binding activities of the TRX-NAI1 fusion proteins to the pNAI2 probe with competition from three mutant versions in the DNA sequence of the pNAI2 probe: (i) mutation of canonical G-box (pNAI2-mG1/G2); (ii) mutation of the non-canonical G-box (pNAI2-G1/mG2) and (iii) mutation of both G-boxes (pNAI2-mG1/mG2) (). Interestingly, the binding activity of NAI1 to the pNAI2 probe was inhibited either by the excess of unlabeled pNAI2-mG1/G2 or by pNAI2-G1/mG2 ()). However, pNAI2-mG1/mG2 had no competitive ability ()), indicating that the presence of either of G-boxes is crucial for the binding of NAI1 to the pNAI2 probe.
Table 1. Probe and competitor sequences
Figure 1. In vitro DNA binding activity of NAI1. (a) A coomassie brilliant blue-stained gel image of purified thioredoxin (TRX) and recombinant TRX-NAI1 fusion protein (arrowhead). (b) Diagrams of G-box and E-box positions in the NAI2 and BGLU23 promoters. The numbers indicate the nucleotide base pairs from the starting codon (ATG). (c) EMSA showing NAI1 binding to the pNAI2 probe in a G-box-dependent manner. (d) EMSA of NAI1 and the pNAI2 probe with the various competitive DNA fragment as indicated. The sequences of the competitive DNA fragments are shown in Table 1
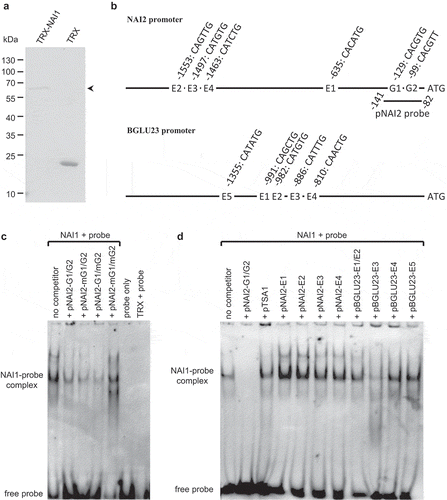
bHLH transcription factors have the potential to bind to a variety of E-box sequences in the regulatory regions.Citation16,Citation17 Further, we searched for any G-boxes or E-boxes in the NAI2 and BGLU23 promoters, where NAI1 is proposed to be a direct regulator of these genes. We found that the promoters of NAI2 and BGLU23 have four and five E-boxes, respectively ()). We examined the binding ability of NAI1 to these E-boxes by accomplishing an EMSA with the NAI1 protein and the pNAI2 probe in the presence of excess unlabeled DNA, including the E-box sequences (). Competitors involving the E-box motifs in the NAI2 promoter were not able to inhibit the binding of NAI1 to the pNAI2 probe ()). This result demonstrates that the two G-box motifs from the NAI2 promoter are critical for the NAI1 binding to DNA. Additionally, the binding of NAI1 protein to the pNAI2 probe was inhibited by the excess of unlabeled pBGLU23-E3, unlike other competitors from the BGLU23 promoter sequence ()), which indicates that NAI1 has a potential to bind to this region in the BGLU23 promoter, as has been hinted to by Matsushima et al.Citation13
Next, we examined the binding ability of NAI1 to a G-box like motif (CACGTTT) in the Arabidopsis TSA1 promoter, which is suggested as a regulatory region for TSA1 through competition analysis of unlabeled oligonucleotide (pTSA1, ) in the presence of the pNAI2 probe. We found that pTSA1 had no competitive ability toward the pNAI2 probe, suggesting that the NAI1 protein is unable to bind to this region in the TSA1 promoter ()).
To understand the NAI1 function in the plant we expressed the green fluorescent protein (GFP)-NAI1 fusion protein under the control of the NAI1 promoter and analyzed its localization in Arabidopsis stable transgenic lines. We found the fluorescence of GFP-NAI1 in the nucleus of epidermis cells of the hypocotyl and roots, indicating that NAI1 is a nuclear-localized protein ()). The subcellular localization of NAI1 was also analyzed by transient expression of a GFP-NAI1 fusion protein in Nicotiana benthamiana leaves with agroinfiltration. GFP-NAI1 was observed in the nucleus when the expression was driven by the constitutively expressing CaMV35S promoter. The nuclear localization of NAI1 is consistent with its function as a transcription factor. GFP-NAI1 did not express under the NAI1 promoter ()), suggesting that the NAI1 promoter is not active in the leaves of N. benthamiana. The expression of GFP alone exhibited the fluorescent signals in both the cytoplasm and the nuclei.
Figure 2. NAI1 localization in the plant cells. Confocal laser scanning microscope (GFP) and bright-field image of 7-day-old seedlings of Arabidopsis transgenic plants (a), and N. benthamiana leaves that are infiltrated with Agrobacterium harboring respective constructs (b). Scale bars are 50 µm
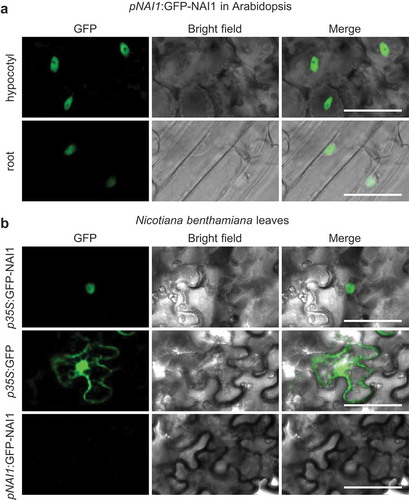
To test whether NAI1 activates the NAI2 promoter in planta, we performed an effector-reporter assay ()). When we co-introduced CaMV35S promoter-driven GFP-NAI1 (p35S:GFP-NAI1) and the NAI2 promoter-driven β-glucuronidase reporter (pNAI2:GUS) constructs into N. benthamiana by agroinfiltration, the dark blue signal of GUS staining was detected ()). The GUS staining was not detected when we introduced p35S:GFP instead of p35S:GFP-NAI1. The results demonstrated that the GFP-NAI1 protein can activate the NAI2 promoter to induce GUS expression. In contrast, the GUS staining was weakly detectable with a construct having a mutation in the two G-box elements in the NAI2 promoter (mut-pNAI2:GUS), indicating that GFP-NAI1 cannot activate the mutated NAI2 promoter ()). This result demonstrates that NAI1 activates the NAI2 promoter by interacting with the G-box elements in plant cells. When we used a construct of GFP-NAI1 driven by the NAI1 promoter (pNAI1:GFP-NAI1), the expression of the GUS gene was not observed ()). These results also validate the idea that the NAI1 promoter is not enough by itself to induce NAI1-GFP in the leaves of N. benthamiana.
Figure 3. Activation of NAI2 promoter by NAI1. (a) Schematic model of DNA construct used in the experiment. (b) Images of GUS staining in the N. benthamiana leaves. The Agrobacterium harboring the effector construct is co-infiltrated with the Agrobacterium harboring the reporter construct, followed by the GUS staining. Scale bar is 0.5 cm
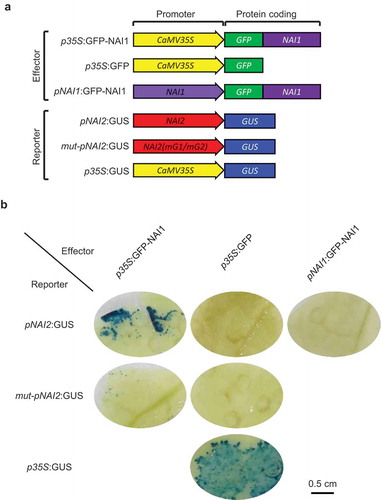
Here we verified the binding ability of NAI1 to the G1 and G2 motifs in the NAI2 promoter. Furthermore, we confirmed the in vivo activation of the NAI2 promoter by NAI1. A nuclear-localized NAI1 can activate the NAI2 promoter as a transcription factor through its two G-box elements. The NAI1 protein recognizes G-box (CACGTG) and G-box like (CACGTT) sequences in the NAI2 promoter, suggesting that NAI1 is a direct regulator of the NAI2 gene. This is consistent with the fact that the NAI1 gene regulates the expression of NAI2 in Arabidopsis.Citation14 In contrast, NAI1 did not bind to a DNA sequence from the TSA1 promoter, which also contains a G-box like (CACGTTT) motif. The finding suggests that flanking sequences spanning the G-box like motifs might affect the recognition by NAI1, in addition to the presence of the G-box like motif. The finding is still intriguing and it is consistent with the in planta situation because there is no evidence that the NAI1 gene regulates the expression of TSA1 in Arabidopsis.Citation7
A competitor experiment in EMSA showed that NAI1 binds to pBGLU23-E3, suggesting that it recognizes the E-box motif (CATTTG) in the pBGLU23-E3. Because NAI1 regulates the expression of BGLU23 in Arabidopsis,Citation13 the motif will be a regulatory element in the BGLU23 promoter. The binding of NAI1 to the G-box and to one of the E-box motifs suggests that NAI1 could regulate other genes that have these motifs. Because NAI1 is a central regulator for the ER body formation,Citation13 and ER bodies are involved in plant chemical defense against herbivory,Citation6,Citation9 our analysis will provide information about how NAI1 recognizes the target genes to raise the plant defense systems.
Materials and methods
NAI1 protein preparation
We obtained a plasmid DNA harboring NAI1 cDNA in the Gateway entry vector pDONR201 (clone no. REGIo476B122D) from Source BioScience (Nottingham, UK). The NAI1 coding region in pDONR201 was transferred to pET32a-GW. The pET32a-GW was derived from pET32a (Novagen, Madison, Wisconsin, USA), which had been modified into a Gateway vector by using the Gateway Vector Conversion System (Invitrogen).Citation30 For bacterial expression of the recombinant protein (TRX-NAI1-His-Tag), the plasmid was introduced into BL21(DE3) pLysS Rosetta competent cells (Novagen). Expression of the recombinant protein was induced by culturing the cells in 150 mL lysogeny broth (LB) medium containing 0.4 mM isopropyl β-D-1-thiogalactopyranoside (IPTG) for 18 h at 18°C. For protein purification, cells were harvested from the induction culture and resuspended in CelLytic B lysis buffer (Sigma). The bacterial cells were lysed by sonication, then centrifuged and the supernatant was mixed in equal proportion with an equilibration buffer (50 mM sodium phosphate, 300 mM NaCl, 10 mM imidazole, pH 8.0) before being loaded into pre-equilibrated Ni-NTA Agarose resin (Qiagen). Following three rounds of washing with a batch of imidazole that ranged from 20 to 100 mM, His-tagged TRX-NAI1 protein was eluted using 500 mM imidazole. The protein solvent was replaced with PBS (10 mM sodium phosphate, pH 7.2, and 130 mM NaCl) by dialysis and further concentrated by an Amicon Ultra Centrifugal device (Thermo Fisher).
Electrophoretic mobility shift assay
A biotinylated DNA double-stranded probe of pNAI2-G1/G2 was made by annealing a 50-μm mixture of complementary oligonucleotides in 10 mM Tris-HCl, pH 8.0, 50 mM NaCl, and 1 mM EDTA by heating to 95°C for 5 min, followed by slowly cooling to room temperature. Binding reactions were induced by incubation of THX-NAI1 (1 µg in , 0.3 µg in ) and probe (5 µM) on ice for 30 min and then resolved by 6% PAGE. The competitor oligonucleotides were added to the binding reaction mixture 15 min before the addition of the probe. After electrophoresis, the products were transferred to a positively charged nylon membrane (Thermo-Fisher). DNA–protein interactions were visualized by the same method as western blotting, except the membrane was blocked with 3% skimmed milk and probed with Pierce Avidin horseradish peroxidase conjugated (Thermo-Fisher). Competition assays were performed using 5x () and 20x () excess unlabeled probes in the binding reactions.
Subcellular localization analysis of NAI1
Arabidopsis Columbia accession was used to generate transgenic plants that expressed GFP-NAI1 under control of the NAI1 promoter (pNAI1:GFP-NAI1). We amplified four DNA fragments by PCR; promoter region of the NAI1 gene, coding and terminator regions of the NAI1 gene, sGFP, and the vector backbone of pENTR1AxeCitation6 with the respective primer sets (Supplemental Table 1). The amplified DNA fragments were fused by an In-Fusion reaction (Takara, Japan), resulting in a Gateway entry vector pENTR1Axe/ProNAI1:sGFP-NAI1g:TerNAI1. Then the entire region was transferred to a vector pGWB501 (a gift from T. Nakagawa) to generate pGWB501/ProNAI1:sGFP-NAI1g:TerNAI1. The vector was then introduced into the Agrobacterium tumefaciens strain GV3101, then transformed into Arabidopsis.
The Gateway binary vector pGWB406 (a gift from T. Nakagawa) was used for the generation of a p35S:GFP-NAI1 overexpression construct, which consisted of NAI1 ORF cDNA bearing a N‐terminal fusion to GFP under the control of the Cauliflower mosaic virus (CaMV) 35S promoter.Citation31 The resulting plasmid, pGWB406/NAI1, was transformed into the A. tumefaciens strain GV3101(pMP90RK) by electroporation. pGWB406, which expresses GFP under the control of the CaMV 35S promoter,Citation31 served as the control. To suppress gene silencing, we used A. tumefaciens containing the p19 silencing suppressor (a gift from W. Strzałka) of Tomato busy shunt virus (TBSV).Citation32 The bacteria were cultured in LB medium-containing rifampicin, kanamycin, and spectinomycin at 28°C for 2 days, harvested, and resuspended in an infiltration medium (10 mM MES, 10 mM MgCl2, and 150 µM acetosyringone). The bacteria harboring a gene of interest were diluted in the infiltration medium as OD600 = 1.0, and the bacteria containing the p19 silencing suppressor were diluted in the infiltration medium as OD600 = 0.5, subsequently, equal volumes of these bacteria solutions are mixed. The bacteria mixture is incubated for 4 h at 28°C, and then infiltrated with a 1-mL syringe without a needle into the abaxial air spaces of 4-week-old leaves of N. benthamiana. After 36 to 40 h, GFP fluorescence was observed in a laser scanning confocal microscope (LSM 880, Zeiss).
GUS expression analysis
The 635-bp long promoter region of the NAI2 gene was amplified by PCR from Arabidopsis (Col-0 accession) genomic DNA with the primers of pNAI2HindIIIF and pNAI2BamHIR (Supplemental Table 1). The sequence of the NAI2 promoter was verified and subsequently, it was cloned into pBI121 (Clontech) at the HindIII and BamHI sites, replacing the CaMV35S promoter to generate pBI121/ProNAI2:GUS. To generate the pBI121/mut-ProNAI2:GUS, overlap extension PCRCitation33 was conducted to substitute the core sequence of canonical and non-canonical G-box elements (G1/G2-boxes) in the NAI2 promoter. Briefly, two rounds of PCR reactions were conducted using Arabidopsis genomic DNA as a template and primers (pNAI2HindIIIF and complement strand for pNAI2-mG1/mG2p; NAI2BamHIR and forward strand for pNAI2-mG1/mG2p). The products obtained from the above PCR reactions were mixed in a 1:1 ratio and subsequently a third PCR was performed using primers (pNAI2HindIIIF and pNAI2BamHIR) to amplify the mutated G1/G2-boxes containing NAI2 promoter fragment. The mutated sequence in the NAI2 promoter was verified and subsequently, it was cloned into the pBI121 at the HindIII and BamHI sites. The resulting plasmids were introduced into the A. tumefaciens strain EHA105 by electroporation. The bacteria-harboring effector (p35S:GFP, p35S:GFP-NAI1, or pNAI1:GFP-NAI1) or reporter (pNAI2:GUS, mut-pNAI2:GUS, or p35S:GUS) constructs were diluted in the infiltration medium as OD600 = 0.6, and the bacteria-harboring p19 silencing suppressor were diluted in the infiltration medium as OD600 = 0.3. Equal volumes of these bacteria solutions were mixed, and then co-infiltrated into leaves of N. benthamiana at the 5-leaf stage using a 1-mL syringe. After infiltration, the plants were maintained for 3 days in a growth chamber at 28°C under a photoperiod of 16 h light.
GUS staining was performed as described previouslyCitation34 with minor modifications. The infiltrated leaves were cut into pieces and soaked in the GUS staining buffer (100 mM sodium phosphate, 1 mM 5-bromo-4-chloro-3-indolyl-β-D-glucuronide, 0.5 mM potassium ferrocyanide, 0.5 mM potassium ferricyanide, 10 mM EDTA, pH 7.0) and then vacuum infiltrated for 5 min and incubated at 37°C overnight. After the leaf samples were stained for GUS, they were treated with 70% (v/v) ethanol until pigments, such as chlorophylls, were completely cleared and then washed with sterilized water for decolorization.
Disclosure of potential conflicts of interest
No potential conflicts of interest were disclosed.
Supplemental Material
Download MS Excel (9.8 KB)Acknowledgments
We are grateful to Tsuyoshi Nakagawa (Shimane University), Wojciech Strzałka (Jagiellonian University), Haruko Ueda (Konan University), and Shoji Mano (NIBB) for their donation of the vectors and Arabidopsis plants. We thank Yumi Konnai (Konan University) for her helpful assistance in vector construction. This work is supported; by the OPUS grant from the National Science Centre of Poland to K.Y. and N.S. [grant number; UMO-2016/23/B/NA1/01847]; by the TEAM grant from the Foundation for Polish Science to S.S. [grant number; TEAM/2017-4/41]; by the institutional support from the Małopolska Centre of Biotechnology, Jagiellonian University; by Grants-in-Aid for Scientific Research to I.H.-N. (no. 15H05776 and 22000014) from the Japan Society for the Promotion of Science (JSPS); and by the Hirao Taro Foundation of KONAN GAKUEN for Academic Research to I.H.-N.
Supplementary material
Supplemental data for this article can be accessed on the publisher’s website.
Additional information
Funding
References
- Iversen TH. The morphology, occurrence, and distribution of dilated cisternae of the endoplasmic reticulum in tissues of plants of the Cruciferae. Protoplasma. 1970;71:1–7. doi:10.1007/BF01279689.
- Matsushima R, Hayashi Y, Yamada K, Shimada T, Nishimura M, Hara-Nishimura I. The ER body, a novel endoplasmic reticulum-derived structure in Arabidopsis. Plant Cell Physiol. 2003;44:661–666. doi:10.1093/pcp/pcg089.
- Gunning BES. The identity of mystery organelles in Arabidopsis plants expressing GFP. Trends Plant Sci. 1998;3:417.
- Matsushima R, Kondo M, Nishimura M, Hara-Nishimura I. A novel ER-derived compartment, the ER body, selectively accumulates a β-glucosidase with an ER-retention signal in Arabidopsis. Plant J. 2003;33:493–502. doi:10.1046/j.1365-313X.2003.01636.x.
- Nakazaki A, Yamada K, Kunieda T, Tamura K, Hara-Nishimura I, Shimada T. Biogenesis of leaf endiolasmic reticulum body is regulated by both jasmonate-dependent and independent pathways. Plant Signal Behav. 2019;14:e1622982. doi:10.1080/15592324.2019.1622982.
- Nakazaki A, Yamada K, Kunieda T, Sugiyama R, Hirai YM, Tamura K, Hara-Nishimura I, Shimada T. Leaf endoplasmic reticulum bodies identified in Arabidopsis rosette leaves are involved in defense against herbivory. Plant Physiol. 2019;179:1515–1524. doi:10.1104/pp.18.00984.
- Stefanik N, Bizan J, Wilkens A, Tarnawska-Glatt K, Goto-Yamada S, Strzałka K, Nishimura M, Hara-Nishimura I, Yamada K. NAI2 and TSA1 drive differentiation of constitutive and inducible ER body formation in Brassicaceae. Plant Cell Physiol. 2020;61(4):722–734. doi:10.1093/pcp/pcz236.
- Matsushima R, Hayashi Y, Kondo M, Shimada T, Nishimura M, Hara-Nishimura I. An endoplasmic reticulum-derived structure that is induced under stress conditions in Arabidopsis. Plant Physiol. 2002;130(4):1807–1814. doi:10.1104/pp.009464.
- Yamada K, Goto-Yamada S, Nakazaki A, Kunieda T, Kuwata K, Nagano AJ, Nishimura M, Hara-Nishimura I. Endoplasmic reticulum-derived bodies enable a single-cell chemical defense in Brassicaceae plants. Commun Biol. 2020;3(1):21. doi:10.1038/s42003-019-0739-1.
- Nakano RT, Piślewska-Bednarek M, Yamada K, Edger PP, Miyahara M, Kondo M, Böttcher C, Mori M, Nishimura M, Schulze-Lefert P, et al. PYK10 myrosinase reveals a functional coordination between ER bodies and glucosinolates in Arabidopsis thaliana. Plant J. 2017;89:204–220.
- Nakano RT, Yamada K, Bednarek P, Nishimura M, Hara-Nishimura I. ER bodies in plants of the Brassicales order: biogenesis and association with innate immunity. Front Plant Sci. 2014;5:73. doi:10.3389/fpls.2014.00073.
- Lüthy B, Matile P. The mustard oil bomb: rectified analysis of the subcellular organisation of the myrosinase system. Biochem Physiol Pflanzen. 1984;179:5–12. doi:10.1016/S0015-3796(84)80059-1.
- Matsushima R, Fukao Y, Nishimura M, Hara-Nishimura I. NAI1 gene encodes a basic-helix-loop-helix-type putative transcription factor that regulates the formation of an endoplasmic reticulum-derived structure, the ER body. Plant Cell. 2004;16:1536–1549. doi:10.1105/tpc.021154.
- Yamada K, Nagano AJ, Nishina M, Hara-Nishimura I, Nishimura M. NAI2 is an endoplasmic reticulum body component that enables ER body formation in Arabidopsis thaliana. Plant Cell. 2008;20:2529–2540. doi:10.1105/tpc.108.059345.
- Geem KR, Kim DH, Lee DW, Kwon Y, Lee J, Kim JH, Hwang I. Jasmonic acid-inducible TSA1 facilitates ER body formation. Plant J. 2019;97:267–280. doi:10.1111/tpj.14112.
- Voronova A, Baltimore D. Mutations that disrupt DNA binding and dimer formation in the E47 helix-loop-helix protein map to distinct domains. Proc Natl Acad Sci USA. 1990;87:4722–4726. doi:10.1073/pnas.87.12.4722.
- Swanson HI, Chan WK, Bradfield CA. DNA binding specificities and pairing rules of the Ah receptor, ARNT, and SIM proteins. J Biol Chem. 1995;270:26292–26302. doi:10.1074/jbc.270.44.26292.
- Bai M-Y, Fan M, Oh E, Wang Z-Y. A triple helix-loop-helix/basic helix- loop-helix cascade controls cell elongation downstream of multiple hormonal and environmental signaling pathways in Arabidopsis. Plant Cell. 2012;24:4917–4929. doi:10.1105/tpc.112.105163.
- MacAlister CA, Bergmann DC. Sequence and function of basic helix-loop- helix proteins required for stomatal development in Arabidopsis are deeply conserved in land plants. Evol Dev. 2011;13:182–192. doi:10.1111/j.1525-142X.2011.00468.x.
- Pires N, Dolan L. Origin and diversification of basic-helix-loop-helix proteins in plants. Mol Biol Evol. 2010;27:862–874. doi:10.1093/molbev/msp288.
- Yi K, Menand BB, Bell E, Dolan L. A basic helix-loop-helix transcription factor controls cell growth and size in root hairs. Nat Genet. 2010;42:264–267. doi:10.1038/ng.529.
- Toledo-Ortiz G, Huq E, Quail PH. The Arabidopsis basic/helix-loop-helix transcription factor family. Plant Cell. 2003;15:1749–1770. doi:10.1105/tpc.013839.
- Meshi T, Iwabuchi M. Plant transcription factors. Plant Cell Physiol. 1995;36:1405–1420.
- Heim MA, Jakoby M, Werber M, Martin C, Weisshaar B, Bailey PC. The basic helix-loop-helix transcription factor family in plants: a genome-wide study of protein structure and functional diversity. Mol Biol Evol. 2003;20:735–747. doi:10.1093/molbev/msg088.
- Van Moerkercke A, Steensma P, Gariboldi I, Espoz J, Purnama PC, Schweizer F, Miettinen K, Vanden Bossche R, De Clercq R, Memelink J, et al. The basic helix-loop-helix transcription factor BIS2 is essential for monoterpenoid indole alkaloid production in the medicinal plant Catharanthus roseus. Plant J. 2016;88:3–12. doi:10.1111/tpj.13230.
- Van Moerkercke A, Steensma P, Schweizer F, Pollier J, Gariboldi I, Payne R, Vanden Bossche R, Miettinen K, Espoz J, Purnama PC, et al. The bHLH transcription factor BIS1 controls the iridoid branch of the monoterpenoid indole alkaloid pathway in Catharanthus roseus. Proc Natl Acad Sci USA. 2015;112(26):8130–8135. doi:10.1073/pnas.1504951112.
- Mertens J, Pollier J, Vanden Bossche R, Lopez-Vidriero I, Franco-Zorrilla JM, Goossens A. The bHLH transcription factors TSAR1 and TSAR2 regulate triterpene saponin biosynthesis in Medicago truncatula. Plant Physiol. 2016;170(1):194–210. doi:10.1104/pp.15.01645.
- Tamura K, Yoshida K, Hiraoka Y, Sakaguchi D, Chikugo A, Mochida K, Kojoma M, Mitsuda N, Saito K, Muranaka T, et al. The basic Helix–Loop–Helix transcription factor GubHLH3 positively regulates soyasaponin biosynthetic genes in Glycyrrhiza uralensis. Plant Cell Physiol. 2018;59:783–796. doi:10.1093/pcp/pcy046.
- O’Malley RC, Huang SC, Song L, Lewsey MG, Bartlett A, Nery JR, Galli M, Gallavotti A, Ecker JR. Cistrome and epicistrome features shape the regulatory DNA landscape. Cell. 2016;165:1280–1292.
- Yamada K, Fukao Y, Hayashi M, Fukazawa M, Suzuki I, Nishimura M. Cytosolic HSP90 regulates the heat shock response that is responsible for heat acclimation in Arabidopsis thaliana. J Biol Chem. 2007;282:37794–37804.
- Nakagawa T, Suzuki T, Murata S, Nakamura S, Hino T, Maeo K, Tabata R, Kawai T, Tanaka K, Niwa Y, et al. Improved Gateway binary vectors: high-performance vectors for creation of fusion constructs in transgenic analysis of plants. Biosci Biotechnol Biochem. 2007;71:2095–2100.
- Lindbo JA. High-efficiency protein expression in plants from agroinfection-compatible Tobacco mosaic virus expression vectors. BMC Biotechnol. 2007;7:52.
- Ho SN, Hunt HD, Horton RM, Pullen JK, Pease LR. Site-directed mutagenesis by overlap extension using the polymerase chain-reaction. Gene. 1989;77:51–59. doi:10.1016/0378-1119(89)90358-2.
- Jefferson RA, Kavanagh TA, Bevan MW. GUS fusions: β-glucuronidase as a sensitive and versatile gene fusion marker in higher plants. Embo J. 1987;6:3901–3907. doi:10.1002/j.1460-2075.1987.tb02730.x.