ABSTRACT
We have previously described that laboratory strains of Ustilago maydis, a fungal pathogen of maize and its ancestor teosinte, harbor an intracellular bacterium that enables the fungus to fix nitrogen. However, it is not clear whether other strains isolated from nature also harbor endosymbiotic bacteria, and whether these fix nitrogen for its host. In the present study, we isolated U. maydis strains from naturally infected maize. All the isolated strains harbored intracellular bacteria as determined by PCR amplification of the 16S rRNA gene, and some of them showed capacity to fix nitrogen. That these are truly bacterial endosymbionts were shown by the fact that, after thorough treatments with CuSO4 followed by serial incubations with antibiotics, the aforementioned bacterial gene was still amplified in treated fungi. In all, these data support the notion that U. maydis-bacterium endosymbiosis is a general phenomenon in this species.
There is growing evidence that endosymbiotic bacteria are associated with the most major fungal lineages.Citation1–11 These endobacteria may have a role in its host’s pathogenesis, Citation5 sexual reproduction, Citation6 vegetative growth, Citation12 or even primary or secondary metabolism.Citation13,Citation14 Even though the benefit of these endobacteria to its host is apparent, it is not clear how extended is their presence in fungal species. For example, in Rhizopus microsporus all analyzed strains harbor an endosymbiotic bacterium closely related to Burkholderia sp.Citation15 On the other hand, an endobacterium also related also to Burkholderia is present only in some strains within the Gigasporaceae family, except for Gigaspora rosea .Citation16 In other taxa, there is widespread distribution of endosymbionts, for example in Mortierella spp. 53 out of 238 isolates representing 22 species harbored Burkholderia-related endobacteria, which fall into three phylogenetically related groups.Citation7,Citation14,Citation17
Another important question is whether the symbiosis is facultative or obligate, or if it increases the fitness of the host and/or the endosymbiont. Evolutionary analysis of the Glomeromycota-Glomeribacter interaction showed that, in this particular case, this interaction is non-essential for the fungus, as inferred from their pattern of codivergence, and by the fact that not all fungal isolates harbor this endosymbiont.Citation18 A clear advantage provided by bacterial endosymbionts in some instances would be reflected in a widespread presence in the host population.
Ustilago maydis, a fungus within the phylum Basidiomycota, is a pathogen-infecting maize (Zea mays) and its ancestor teosinte (Zea mays ssp parviglumis and mexicana), and is the causal agent of common smut or “huitlacoche.” U. maydis is also a model for the study of plant fungal pathogenicity, Citation19,Citation20 cellular differentiation, Citation21–24 gene regulation, Citation25,Citation26 and fungal sexual reproduction .Citation27 Recently, it was observed that U. maydis harbors a bacterial endosymbiont that likely provides the fungus with the capacity to fix nitrogen.Citation28 This endosymbiont is closely related to Bacillus pumilus, a plant-growth-promoting bacterium that associates with the rhizosphere of plants and is able to fix nitrogen.Citation29,Citation30
All laboratory strains of U. maydis, are derived from teliospores collected in a cornfield near St. Paul, Minnesota [see Supplementary Methods in reference 31]. After propagation, these gave rise to the broadly used FB1, FB2 and ½ strainsCitation21,Citation31,Citation32 (). The B. pumilus endosymbiont described above was shown to be present in the FB1, FB2 and ½ strains.Citation28 However, it is not clear whether strains collected in the wild harbor the same endosymbiont, if any, and whether these are also able to fix nitrogen.
Figure 1. A. Origin of the laboratory strains of U. maydis. Schematic description of the origin of the U. maydis strains used in most laboratories. Teliospores originally collected by J.J. Christensen (for more data see the text of supplementary methods inCitation33). B. Detection of endobacteria in diverse wild U. maydis isolates after treatment of teliospores to eliminate exogenous bacteria (see text). PCR was carried out using F21 and R1494 primers. The products were separated by agarose gel electrophoresis. Left to right: 1, W29 (Y. lipolytica); 1kb plus DNA ladder (m); 2, Bucaramanga (CO), 3, Tehuacán (PU); San Juan Teltipac, Oaxaca (OA); 4, Chiapas (CH); 5, Llera (TA); 7, Atlacomulco (AT); 8, Santa Maria (SA); 9, Irapuato (IR); 10, Tlaxcala (TL); 11, Washington (US); 12, Estación Ruiz (ER); 13, Las Adelitas (AD); 14, La Cruz Elota (LCE); 15, Acaponeta (AC); 16, and FB2 satrin. C. Detection of 16S rDNA in representative isolates of wild-collected U. maydis isolates by PCR after successive treatments with antibiotics (see text). 1, M (1kb plus DNA Ladder); 2, IR; 3, US; 4, CO; 5, FB1 strain; 6, FB2 strain; 7. FB2 strain after serial antibiotic treatment (experiment 1); 8, FB2 strain after serial antibiotic treatment (experiment 2); 9, Burkholderia vietnamensis (positive control); 10, Non-template control
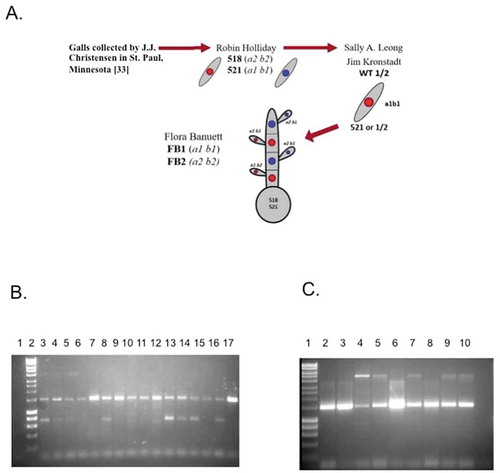
The present study was carried out as a first approximation to determine how widespread is the presence of bacterial endosymbionts in wild strains of U. maydis, (i.e., within a species) as well as to analyze the ability of these wild strains to fix nitrogen. To this end, we isolated several strains in 12 states in México. In addition, we obtained a strain from an infected cob from a cornfield from Washington State (United States), and another strain from a cornfield in Bucaramanga, Colombia.
From the germinated teliospores we isolated at least 10 or more haploid yeast colonies, to be used for subsequent analyses. From these sets, one single colony was randomly selected from each location. The other individuals were stored at −70°C for further analysis.
All the Mexican strains were isolated from infected cobs existing in small fields where different “criollo” landraces had been constantly grown and avoided the material from commercial cultivars. The laboratory strains FB1 (a1b1) and FB2 (a2b2)Citation21 were used as positive controls for the presence of endobacteria, and Yarrowia lipolytica W29 strain (kindly provided by Prof. Angel Domínguez, University of Salamanca, Spain) was used as negative control, since no endobacteria have been found in this fungus. Once isolated, all strains were propagated in complete medium (CM)Citation31 and kept at −70°C in a 50% glycerol solution. Tumors from infected cobs were crushed and ground with mortar and pestle under sterile conditions, filtered through sterile gauze, and the released teliospores separated by centrifugation at 5000 g and washed with sterile-distilled water by centrifugation. Subsequently, the teliospores were incubated overnight in a 1% CuSO4 solution and centrifuged and washed with sterile-distilled water, at least five times. The sedimented teliospores were recovered and inoculated in liquid minimal medium (MM), pH 7.Citation31 Briefly, 10 ml of complete medium with 1 mg/ml ampicillin was inoculated with 106 cells/ml and incubated at 25°C with shaking (150 rpm) for 24 hours to discard contamination from exogenous bacteria. Cells were then washed with sterile water. describes phenotypic characteristics of the aforementioned isolates. Genomic DNA was extracted from these and subjected to PCR for 16S rRNA as previously described using specific primers (Table S1).Citation28 Potential endobacteria were detected in all U. maydis wild isolates ()). To discard that the amplified product originated from exogenous contaminating bacteria, liquid cultures of U. maydis isolates were successively incubated with various antibiotics at 25°C with shaking (150 rpm) for 24 hours; initially, with 1 mg/ml ampicillin; and followed by 0.5 mg/ml kanamycin, 1 mg/ml trimethoprim, and 1 mg/ml cefalexin. Cells were washed three times with sterile water after each treatment. After the treatment mentioned above, amplification of 16S rDNA was observed in the Irapuato (IR), US, and Colombia (CO) isolates, along with the FB1 and FB2 strains ()). Thus, contamination from exogenous bacteria (i.e., adhered to the fungal cell surface) can be ruled out. The PCR product may correspond actually to different bacteria; this must be established through metagenomic analysis, currently underway by our group. However, some PCR products were cloned and sequenced from colonies obtained from fungal isolates, corresponding to Bacillus, Burkholderia and other potential nitrogen-fixing bacteria (Genbank submission No. SUB8004302). A more detailed analysis will help determine the precise identity of these bacteria, and whether there is more than one bacterial taxon associated with the fungal host.
Table 1. Phenotypic characterization of wild collected strains U. maydis.
The initial phenotypic trait determined in the strains isolated from the wild was their capacity to grow in a medium without nitrogen in order to select strains carrying a nitrogen-fixing bacterium. Accordingly, nitrogen starved cells of each one of the initially isolated strains of U. maydis, the positive control strains FB1 and FB2, and the negative Y. lipolytica negative control, were inoculated in 125 ml flasks containing 30 ml of pH 7 MM with or without KNO3, essentially as described.Citation28 Initially and at 24 h time periods, a sample to measure OD600 was withdrawn. All strains were able to grow in the medium containing KNO3 (not shown). More importantly, all strains were able to grow in medium with no added nitrogen source, except for Y. lipolytica, (Supporting Table 3; )).
Figure 2. A, Representative data of the growth of U. maydis strains in a medium without fixed nitrogen. Growth kinetics were measured as absorbance at 600 nm (OD600) in minimal media pH 7 without nitrogen [MM7-N]. FB2, Laboratory strain; TL, a representative wild collected strain from Tlaxcala; IR, wild collected strain from Irapuato. n = 3. B. PCR detection of nifH in independent U. maydis isolates. Lanes: 1, 1 kb Plus marker; 2, AC; 3, ER; 4; LCE; 5, SM; 6, OA;7, PU;8, (-); 9, FB2; 10, FB2 Feα; 11, 1 kb Plus. C. PCR detection of nifH in independent U. maydis isolates. Lanes: 1, 1 kb Plus marker; 2, IR; 3, IR; 4, IR; 5, IR; 6, TL; 7, TL; 8, FB2
![Figure 2. A, Representative data of the growth of U. maydis strains in a medium without fixed nitrogen. Growth kinetics were measured as absorbance at 600 nm (OD600) in minimal media pH 7 without nitrogen [MM7-N]. FB2, Laboratory strain; TL, a representative wild collected strain from Tlaxcala; IR, wild collected strain from Irapuato. n = 3. B. PCR detection of nifH in independent U. maydis isolates. Lanes: 1, 1 kb Plus marker; 2, AC; 3, ER; 4; LCE; 5, SM; 6, OA;7, PU;8, (-); 9, FB2; 10, FB2 Feα; 11, 1 kb Plus. C. PCR detection of nifH in independent U. maydis isolates. Lanes: 1, 1 kb Plus marker; 2, IR; 3, IR; 4, IR; 5, IR; 6, TL; 7, TL; 8, FB2](/cms/asset/20f688a0-ed31-4467-af85-df37b569ed86/kpsb_a_1855016_f0002_b.gif)
To analyze whether the bacterium was a nitrogen-fixer, we analyzed the presence of the nifH gene also by PCR using a pair of oligonucleotides that are specific for alpha, beta, and delta-proteobacteria, and firmicutes (Table S1). PCR conditions were as described.Citation28 A product of the expected size was obtained in some, but not all isolates (Table S2 and S3; )). Colonies from the IR isolate were obtained, and the presence of the nifH gene confirmed also by PCR ()). These results suggest that some isolates might harbor different bacteria (i.e., lacking nif genes) relative to the other isolates.
Acetylene reduction is an indirect but reliable assay to determine nitrogenase activity, which was performed essentially as described previously.Citation34–36 Using this analysis in the wild isolated U. maydis strains variable results were obtained (). While some strains consistently showed such activity in independent tests, other strains (IR, TL, PU, and SM) displayed nitrogenase activity only in some cases. In yet other strains (AD, TA, CH, and CO), acetylene reduction was not observed.
15N2 isotopic experiments were carried out to unequivocally confirm diazotrophy (see Table S3) as described in a previous work .Citation28 In these assays Yarrowia lipolityca was included as negative control, and Burkholderia vietnamiensis (collected in the wild by our group) as positive control. The results obtained are shown in Table S3. It is important to observe that not all U. maydis strains showed a higher rate of nitrogen fixation than Y. lipolytica (a non-diazotrophic fungus). These strains were SM, ER, OA, and CE; these results would suggest that either the capacity to fix nitrogen in the strains from these locations is reduced, or harbor different endobacteria. In contrast, in other strains: AC, TA, and US, fixation of isotopic nitrogen was remarkable. Another point to remark is that in some strains where nitrogen-fixing capacity was lower, such as OA and CE, their activity for acetylene reduction was normal. Indeed, AO presents an elevated activity, just below the bacterial control, whereas strain SM was considered a presumptive acetylene reducer since no activity was detected in any of the experimental triplicates (Table S3). These results suggest that not all endobacteria found in different U. maydis isolates fix nitrogen, and thus their populations are heterogeneous. Metagenomic analysis of such isolates will help solve this issue.
U. maydis protoplasts from different isolates strains were prepared as described.Citation37 Protoplasts were subjected to osmotic lysis and fixed with 2% glutaraldehyde. Samples were placed on copper grids, and negatively stained with 2.5% uranyl acetate.Citation38 After this treatment samples were visualized in a Morgagni M-268 Phillips/FEI electron microscope (Amsterdam, NL). Disrupted protoplasts of the laboratory strain FB2, and the wild isolated strains PU, OA, and TA were negatively stained with uranyl acetate and observed under the electron microscope. In all cases stained spherical structures 0.2–0.5 μm in diameter were observed (, red arrows) in the cell debris. These structures are similar to those observed previously in the laboratory strain FB2.Citation28
Figure 3. Determination of the presence of bacteria-like structures in U. maydis protoplasts. Electron microscopy of negatively stained broken protoplasts: A) FB2, B) TA (Llera, Tamaulipas), C) OA (San Juan Teltipac, Oaxaca), D) PU (Tehuacán, Puebla). Scale bar for electron microscopy: A and C 200 nm; B and E 500 nm
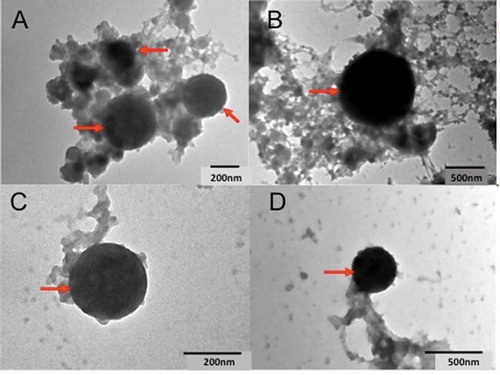
The results shown here are evidence that all the strains isolated from the naturally infected maize plants harbor endobacteria, and in most cases, this probably conferred the fungus the capacity to live without fixed nitrogen, which would have obvious competitive advantages. Different assays to detect the capacity to fix N2, i.e. i) amplification the nifH gene, ii) nitrogenase activity measured by acetylene reduction, and iii) fixation of 15N2, did not yield always the same results, possibly due to technical reasons. Accordingly, it is necessary to consider that the number of copies of both 16S rDNA and nifH genes in bacteria varies widely. For example, some bacteria species have up to 10–12 copies of the 16S rRNA gene, Citation39 whereas nif genes are localized in a single operon within a plasmid, at least in many Rhizobium species.Citation40 Furthermore, the large amount of fungal DNA could make difficult the detection of bacterial DNA and thus the 16S rRNA gene. Regarding the acetylene reduction assay, and the fixation of 15N2, it is important to remark that U. maydis respiratory activity is very high, and that oxygen is a known inhibitor of nitrogenase. Accordingly, most diazotrophic bacteria generate different responses to avoid dissolved oxygenCitation41. At the present time, the mechanisms employed by the fungus to avoid oxygen toxicity to the endobacterial nitrogenase are unknown. Accordingly, the conditions that lead to acetylene reduction in vitro may be different from in vivo conditions, and variable in the different strains.
One point that must be considered is that, currently, it is not known whether all the isolated U. maydis strains harbor the same bacterium species, and even if there exists one or various endobacteria species in all the isolated U. maydis strains. This last event is rare but not impossible.Citation42–43 Metagenomic analyses to resolve this question is, underway in our group.
Presently, the benefit of harboring endobacteria in fungi, at least in the case of U. maydis, is not clear. Preliminary experiments of isotopic dilution and direct isotopic nitrogen fixation suggest that nitrogen fixation in U. maydis does not occur during the pathogenic cycle in maize (data not shown). In nature, teliospore germination probably starts until direct contact with maize leaves. It is at this stage of the life cycle that the nitrogen-fixing capacity would be an advantageous trait. Further analysis is necessary to understand this genetic and metabolic relationship.
It must be stressed that this work also opens the basis for important aspects regarding the origin and role of the symbiosis; mainly i) was this association initiated with U. maydis on maize, or did it occur previously with the maize ancestor, teosinte (Zea mays ssp parviglumis and mexicana, and how stable it is?; ii) does the only role of the endobacterium is that of nitrogen fixation, or is it involved in other aspects of U. maydis physiology?; iii) is there only a single endobacterium species, or there are several bacterial species associated with fungus?, if so, do they play different roles?; iv) is this association strictly necessary for U. maydis survival, or can the bacterium be eliminated without any harm to the fungus? All these important aspects are presently under analysis by our research group.
Abbreviation
Disclosure of potential conflicts of interest
The authors declare none.
Supplemental Material
Download MS Word (16.1 KB)Acknowledgments
This work was partially supported by Consejo Nacional de Ciencia y Tecnología CONACYT, México. Isotopic analyses of 15N were carried out with support from IAEA grant No. ARCAL/05/78.
Dr. Lino Sánchez-Segura’s help with TEM techniques is appreciated. We also thank the members of our laboratories for their technical help.
FPR is a PhD student supported by a fellowship from CONACYT.
Supplementary material
Supplemental data for this article can be accessed on the publisher’s website.
Additional information
Funding
References
- Arendt KR, Hockett KL, Araldi-Brondolo SJ, Baltrus DA, Arnold AE, Cullen D. Isolation of endohyphal bacteria from foliar ascomycota and in vitro establishment of their symbiotic associations. Appl Environ Microbiol. 2016;82:1–6. doi:10.1128/AEM.00452-16.
- Bertaux J, Schmid M, Hutzler P, Hartmann A, Garbaye J, Frey-Klett P. Occurrence and distribution of endobacteria in the plant-associated mycelium of the ectomycorrhizal fungus Laccaria bicolor S238N. Environ Microbiol. 2005;7(11):1786–1795. doi:10.1111/j.1462-2920.2005.00867.x.
- Bianciotto V, Bandi C, Minerdi D, Sironi M, Tichy HV, Bonfante P. An obligately endosymbiotic mycorrhizal fungus itself harbors obligately intracellular bacteria. Appl Environ Microbiol. 1996;62(8):3005–3010. doi:10.1128/AEM.62.8.3005-3010.1996.
- Desirò A, Salvioli A, Ngonkeu EL, Mondo SJ, Epis S, Faccio A, Kaech A, Pawlowska TE, Bonfante P. Detection of a novel intracellular microbiome hosted in arbuscular mycorrhizal fungi. Isme J. 2014;8:257–270. doi:10.1038/ismej.2013.151.
- Fitzpatrick EE First bacterial endosymbionts found in the phylum Ascomycota. Dissertations and Theses. 2013; paper 675.
- Partida-Martínez LP, Monajembashi S, Greulich KO, Hertweck C. Endosymbiont-dependent host reproduction maintains bacterial-fungal mutualism. Curr Bio. 2007;17:773–777. doi:10.1016/j.cub.2007.03.039.
- Sato Y, Narisawa K, Tsuruta K, Umezu M, Nishizawa T, Tanaka K, Yamaguchi K, Komatsuzaki M, Ohta H. Detection of betaproteobacteria inside the mycelium of the fungus Mortierella elongate. Microbes Environ. 2010;25:321–324. doi:10.1264/jsme2.ME10134.
- Schüßmer A, Mollenhauer D, Schnepf E, Kluge M. Geosiphon pyriforme, an endosymbiotic association of fungus and cyanobacteria: the spore structure resembles that of arbuscular mycorrhizal [AM] fungi. Bot Acta. 1994;107:36–45. doi:10.1111/j.1438-8677.1994.tb00406.x.
- Sharma M, Schmid M, Rothballer M, Hause G, Zuccaro A, Imani J, Kämpfer P, Domann E, Schäfer P, Hartmann A, et al. Detection and identification of bacteria intimately associated with fungi of the order Sebacinales. Cell Microbiol. 2008;10:2235–2246. doi:10.1111/j.1462-5822.2008.01202.x.
- Bonfante P, Desirò A. Who lives in a fungus? The diversity, origins and functions of fungal endobacteria living in Mucoromycota. Isme J. 2017;11:1727–1735.
- Deveau A, Bonito G, Uehling J, Paoletti M, Becker M, Bindschedler S, Hacquard S, Hervé V, Labbé J, Lastovetsky OA, et al. Bacterial–fungal interactions: ecology, mechanisms and challenges. FEMS Microbiol Rev. 2018;42:335–352.
- Bianciotto V, Genre A, Jargeat P, Lumini E, Bécard G, Bonfante P. Vertical transmission of endobacteria in the arbuscular mycorrhizal fungus Gigaspora margarita through generation of vegetative spores. Appl Environ Microbiol. 2004;70(6):3600–3608. doi:10.1128/AEM.70.6.3600-3608.2004.
- Hoffman MT, Gunatilaka MK, Wijeratne K, Gunatilaka L, Arnold AE, Corradi N. Endohyphal bacterium enhances production of indole-3-acetic acid by a foliar fungal endophyte. PLoS ONE. 2013;8:1–8. doi:10.1371/journal.pone.0073132.
- Uehling J, Gryganskyi A, Hameed K, Tschaplinski T, Misztal PK, Wu S, Desirò A, Vande Pol N, Du Z, Zienkiewicz A, et al. Comparative genomics of Mortierella elongata and its bacterial endosymbiont Mycoavidus cysteinexigens. Environ Microbiol. 2017;19(8):2964–2983. doi:10.1111/1462-2920.13669.
- Lackner G, Möbius N, Scherlach K, Partida-Martinez LP, Winkler R, Schmitt I, Hertweck C. Global distribution and evolution of a toxinogenic Burkholderia-Rhizopus symbiosis. MBio. 2009;75:2982–2986.
- Bianciotto V, Lumini E, Lanfranco L, Minerdi D, Bonfante P, Perotto S. Detection and identification of bacterial endosymbionts in arbuscular mycorrhizal fungi belonging to the family Gigasporaceae. MBio. 2000;66:4503–4509.
- Desirò A, Faccio A, Kaech A, Bidartondo MI, Bonfante P. Endogone, one of the oldest plant-associated fungi, host unique Mollicutes-related endobacteria. New Phytol. 2015;205:1464–1472. doi:10.1111/nph.13136.
- Mondo SJ, Toomer KH, Morton JB, Lekberg Y, Pawlowska TE. Evolutionary stability in a 400-million-year-old heritable facultative mutualism. Evolution. 2012;66(8):2564–2576. doi:10.1111/j.1558-5646.2012.01611.x.
- Bölker M, Urban M, Kahmann R. The a mating type locus of U. maydis specifies cell signaling components. Cell. 1992;68:441–450. doi:10.1016/0092-8674(92)90182-C.
- Müller P, Weinzierl G, Brachmann A, Feldbrügge M, Kahmann R. Mating and pathogenic development of the smut fungus Ustilago maydis are regulated by one mitogen-activated protein kinase cascade. Eukaryot Cell. 2003;2:1187–1199. doi:10.1128/EC.2.6.1187-1199.2003.
- Banuett F, Different HI. a alleles of Ustilago maydis are necessary for maintenance of filamentous growth but not for meiosis. Proc Natl Acad Sci USA. 1989;86:5878–5882. doi:10.1073/pnas.86.15.5878.
- Banuett F, Herskowitz I. Discrete developmental stages during teliospore formation in the corn smut fungus, Ustilago maydis. Development. 1996;122:2965–2976.
- Cabrera-Ponce JL, León-Ramírez CG, Verver-Vargas A, Palma-Tirado L, Ruiz- Herrera J. Metamorphosis of the Basidiomycota Ustilago maydis: transformation of yeast-like cells into basidiocarps. Fungal Genet Biol. 2012;49:765–771. doi:10.1016/j.fgb.2012.07.005.
- Ruiz-Herrera J, León-Ramírez CG, Guevara-Olvera L, Cárabez-Trejo A. Yeast-mycelial dimorphism of haploid and diploid strains of Ustilago maydis. Microbiology. 1995;141:695–703. doi:10.1099/13500872-141-3-695.
- Feldbrügge M, Bölker M, Steinberg G, Kämper J, Kahmann R. Regulatory and structural networks orchestrating mating, dimorphism, cell shape, and pathogenesis in Ustilago maydis. In: Kües U, Fischer R, editors. The mycota i growth, differentiation and sexuality. Heidelberg, GermanyBerlin: Heidelberg Springer-Verlag; 2006. p. 375–391.
- Heimel K, Scherer M, Schuler D, The KJ. Ustilago maydis Clp1 protein orchestrates pheromone and b-dependent signaling pathways to coordinate the cell cycle and pathogenic development. Plant Cell. 2010;22:2908–2922. doi:10.1105/tpc.110.076265.
- Banuett F. History of the mating types in Ustilago maydis. In: Heitman J, JW K, JW T, LA C, editors. Sex in fungi: molecular determination and evolutionary implications. Washington DC, USA; ASM Press: 2007. p. 351–375.
- Ruiz-Herrera J, León-Ramírez C, Vera-Núñez A, Sánchez‐Arreguín A, Ruiz‐Medrano R, Salgado‐Lugo H, Sánchez‐Segura L, Peña‐Cabriales JJ. A novel intracellular nitrogen-fixing symbiosis made by Ustilago maydis and Bacillus spp. New Phytol. 2015;207:769–777. doi:10.1111/nph.13359.
- Li W, Lee SY, Cho YJ, Ghim SY, Jung HY. Mediation of induced systemic resistance by the plant growth-promoting rhizobacteria Bacillus pumilus S2-3-2. Mol Biol Rep. 2020 Oct 10;47:8429–8438. PMID: 33037963. doi:10.1007/s11033-020-05883-9.
- Masood S, Zhao XQ, Shen RF. Bacillus pumilus promotes the growth and nitrogen uptake of tomato plants under nitrogen fertilization. Sci Hortic (Amsterdam). 2020;272:109581. doi:10.1016/j.scienta.2020.109581.
- Holliday R. The genetics of Ustilago maydis. Genet Res. 1961;2(2):204–230. doi:10.1017/S0016672300000719.
- Kronstad JW, Leong SA. Isolation of two alleles of the b locus of Ustilago maydis. Proc Natl Acad Sci USA. 1989;86:978–982. doi:10.1073/pnas.86.3.978.
- Kämper J, Kahmann R, Bölker M, Ma LJ, Brefort T, Saville BJ, Banuett F, Kronstad JW, Gold SE, Müller O, et al. Insights from the genome of the biotrophic fungal plant pathogen Ustilago maydis. Nature. 2006;444:97–101. doi:10.1038/nature05248.
- Burris RH. Methodology. In: Quispel A, editor. Biology of nitrogen fixation. Amsterdam, Netherlands: North-Holland Publishing Co Amsterdam; 1974. p. 3–42.
- Cavalcante VA, Dobereiner J. A new acid-tolerant nitrogen-fixing bacterium associated with sugarcane. Plant Soil. 1988;108:23–31. doi:10.1007/BF02370096.
- Estrada-de Los Santos P, Bustillos-Cristales Y, Caballero-Mellado J. Burkholderia, a genus rich in plant-associated nitrogen fixers with wide environmental and geographic distribution. Appl Environ Microbiol. 2001;67:2790–2798. doi:10.1128/AEM.67.6.2790-2798.2001.
- Tsukuda T, Carleton S, Fotheringham S, Holloman WK. Isolation and characterization of an autonomously replicating sequence from Ustilago maydis. Mol Cell Biol. 1988;8:3703–3709. doi:10.1128/MCB.8.9.3703.
- Bracker CE, Ruiz-Herrera J, Bartnicki-Garcia S. Structure and transformation of chitin synthetase particles (chitosomes) during microfibril synthesis in vitro. Proc Natl Acad Sci USA. 1976;73(12):4570–4574. doi:10.1073/pnas.73.12.4570.
- Klappenbach JA, Saxman PR, Cole JR, Schmidt TM. rrndb: the ribosomal RNA operon copy number database. Nucleic Acids Res. 2001;29:181–184.
- Palacios R, Brom S, Davila G, Flores M, Girard ML, Romero D, Stepkowski T. Gene amplification in Rhizobium. In: Palacios R, Mora J, Newton WE, editors. New horizons in nitrogen fixation. Dordrecht, The Netherlands; Kluwer Academic Publishers: 1993. p. 581–585.
- FJ B. Biological nitrogen fixation. In: Lugtenberg B editor. Principles of plant-microbe interactions. Hoboken, New Jersey, USA: Springer, Cham; 2015. p. 215–224.
- Gomez NC, Kosheleva IA, Abraham WR, Smalla K. Effect of the inoculant strain Pseudomonas putida KT2442 (pNF142) and of naphthalene contamination on soil bacterial community. FEMS Microbiol Ecol. 2005;54:21–33. doi:10.1016/j.femsec.2005.02.005.
- Heuer H, Krsek M, Baker P, Smalla K, Wellington EM. Analysis of actinomycete communities by specific amplification of genes encoding 16sRNA and gel-electrophoretic separation in denaturing gradients. Appl Environ Microbiol. 1997;63:3233–3241. doi:10.1128/AEM.63.8.3233-3241.1997.