ABSTRACT
To determine the effect of the serine/threonine protein kinase (STPK) gene on leaf lettuce bolting, we utilized virus-induced gene silencing (VIGS) using the TRV vector to silence the target gene. The ‘GB30ʹ leaf lettuce cultivar was the test material, and the methods included gene cloning, bioinformatics analysis, quantitative real-time PCR (qRT-PCR) and VIGS. LsSTPK, was cloned from the ‘GB30ʹ leaf lettuce cultivar via reverse transcription-polymerase chain reaction (RT-PCR). qRT-PCR analysis showed that the expression of LsSTPK in the stem of leaf lettuce was significantly greater than that in the roots and leaves, and after high-temperature treatment, the gene expression in the stems in the experimental group was markedly lower than that in the control groups. Following LsSTPK silencing via the VIGS method, the stem length in the treatment group was significantly greater than that in the blank and negative control groups, and the contents of auxin (IAA), GA3 and abscisic acid (ABA) in the treatment group were greater than those in the other two groups. Flower bud differentiation occurred in the treatment group but not in the control group. The above findings suggested that LsSTPK inhibits the bolting of leaf lettuce under high-temperature conditions.
Introduction
Leaf lettuce (Lactuca sativa L.), a leafy vegetable species originating from the Mediterranean coast, Citation2 belongs to the Lactuca genus in the Compositae family. Leaf lettuce has a crispy texture and is rich in nutrients, including folic acid, protein, Citation3 vitamin C and iron .Citation4 As a widely grown and consumed vegetable, leaf lettuce grows best under temperatures from 7°C to 24°C. When the temperature exceeds 30°C, lettuce grows poorly and bolts easily, resulting in poor quality .Citation5 Because the mechanisms underlying the bolting of leaf lettuce under high–temperature conditions remain unknown, it is important to investigate this phenomenon and establish scientific techniques for its prevention.
Bolting represents the beginning of flowering and is a distinct feature of the transition of plants from vegetative to reproductive growth, and it plays an important role in plant growth and development .Citation6 Bolting is regulated by a variety of environmental and endogenous factors, such as temperature, light signals, day length, developmental stage and plant hormones .Citation7 Researchers have studied vegetable bolting in several species, such as cabbage, Citation8 onion Citation1 and radish, Citation9 by analyzing the characteristics, genetic laws and molecular mechanisms of these species. However, few reports about the bolting of leaf lettuce exist.
Similar to ubiquitination, methylation and acetylation, protein phosphorylation is a posttranslational protein modification that widely occurs in organisms .Citation10 Protein phosphorylation plays a molecular role in enzyme activity regulation and cell signal transduction, and it is a critical step in metabolic regulation in prokaryotes and eukaryotes .Citation10 Protein phosphorylation is achieved by protein kinases, which are involved in the regulation of multiple biological processes, including metabolism, Citation11,Citation12 the cell cycle, Citation13 cytokinesis Citation14 and hormone responses .Citation15,Citation16
Serine/threonine protein kinases (STPKs) are the most important type of protein kinase because they regulate various cellular activities by catalyzing the phosphorylation of functional proteins (for example, enzymes, transport proteins, receptors, nuclear proteins and regulatory proteins) .Citation17–19 PRK1, a STPK gene in Petunia inflata, plays an important role in pollen development and signal transduction during pollination .Citation20 Similarly, AtSK3-2 in Arabidopsis thaliana modulates cell elongation in flower development, Citation21 and NPK1, a plant mitogen-activated protein kinase kinase kinase (MAPKKK), acts as a negative modulator of auxin (IAA) signal transduction .Citation22
Plant PIN-formed (PIN) polarity and the resulting IAA flow directionality are mediated by the antagonistic actions of PINOID kinase and protein phosphatase 2A .Citation23 Gibberellin (GA) increases the activity of calcium-dependent protein kinase (CDPK) in rice, and GA biosynthesis inhibitors significantly reduce the activity of CDPK, which catalyzes the phosphorylation of the GA-binding protein .Citation24 However, the role of other hormones and metabolism or major genes/proteins in bolting is unclear, and the molecular mechanisms also are unclear.
Our previous proteomic research of different phosphorylation processes during the bolting of leaf lettuce under high-temperature conditions revealed reductions in STPK protein abundance. However, the mechanism of action of the STPK gene in leaf lettuce and its relationship with bolting remain unknown. In the present study, we cloned and performed a bioinformatics study of LsSTPK. Further, using qRT-PCR, we analyzed the relative expression of LsSTPK at different stages of treatment under high-temperature conditions and investigated its functionality using VIGS. The present study provides a basis for future research into the mechanism of action of LsSTPK during leaf lettuce bolting.
Materials and methods
Plant material and growth conditions
Seeds of the leaf lettuce (L. sativa L.) cultivar ‘GB30ʹ, which had been stored in our laboratory, were sown in a mixture of sand:soil:peat (1:1:1 v/v) and grown in a greenhouse at Beijing University of Agriculture. The greenhouse conditions were as follows: 14 h of light and 20 ± 2°C during the day; 10 h of darkness and 13 ± 2°C during the night; and 50%-70% relative humidity. When the seedlings had three leaves, they were transplanted to a 10-cm diameter pot. Lettuce plants with six leaves were transferred to a growth chamber for 2 d of acclimatization. The growth conditions included a 20/13°C (day/night) temperature, a 14/10 h (day/night) photoperiod and 60% relative humidity. After 2 d, 5 plants that displayed consistent growth were selected and constituted one replication. The roots, stems and leaves were collected, frozen in liquid nitrogen and stored at −80°C until further analysis of the gene expression in each tissue. The remaining plants were divided into two groups as follows: a control group and a high-temperature treatment group. The conditions of the control group were consistent with the abovementioned growth conditions. Plants in the high-temperature treatment group were moved to another growth chamber with a temperature of 33/25°C (day/night), and the other environmental conditions were unchanged. The plants were treated for 3 h, 6 h, 12 h, 24 h, 48 h, 8 d, 16 d, 24 d or 32 d. For cloning and qRT-PCR, 5 healthy plants that displayed consistent growth were selected, which constituted one replication. The roots, leaves and stems were collected, frozen in liquid nitrogen and stored at −80°C until later use. Each sample was sampled three times (as independent repeats).
Total RNA extraction and first-strand cDNA synthesis
A Spin Column Plant Total RNA Purification Kit (Aidlab Biotechnologies, Ltd., Beijing, China) and First Strand cDNA Synthesis SuperMix (TransGen Biotech, Beijing, China) were used to extract the total RNA from lettuce and synthesize cDNA.
Cloning of the LsSTPK gene
Based on the sequence information obtained from the pretranscriptome sequencing in our laboratory, specific primers for the coding region of LsSTPK were designed. The primer sequences used are shown in and were synthesized by Sangon Biotech Co., Ltd. (Shanghai, China).
Table 1. Primer sequences
The coding sequence (CDS) cDNA of the LsSTPK gene was cloned from the lettuce stem by RT-PCR. PCR amplification was performed using PrimeSTAR HS DNA Polymerase (Takara Biomedical Technology Co., Ltd., Beijing) with a total volume of 20 μL. PCR without a template was used as a negative control. The reaction conditions used were as follows: initial denaturation at 98°C for 5 min; 35 cycles of denaturation at 98°C for 10 s, annealing at 56°C for 15 s and extension at 72°C for 1 min; and a final extension of 72°C for 8 min. The PCR products were electrophoresed on a 1% agarose gel, and the target bands were purified by an EasyPure Quick Gel Extraction Kit (TransGen Biotech, Beijing, China), subcloned into a pTOPO-Blunt vector (Aidlab Biotechnologies Co., Ltd., Beijing, China) and then sequenced by Sangon Biotech Co., Ltd. (Shanghai, China).
Sequence analysis of the LsSTPK gene
The protein parameters of LsSTPK were analyzed and predicted by ProtParam Citation25 (http://web.expasy.org/protparam), and the conserved domains of LsSTPK were analyzed via NCBI CD Search Citation26 (https://www.ncbi.nlm.nih.gov/Structure/cdd/wrpsb.cgi). The subcellular localization was predicted by Cell-PLoc 2.0 (http://www.csbio.sjtu.edu.cn/bioinf/Cell-PLoc-2/) .Citation27 The amino acid sequences of STPK from nine additional species were downloaded from the NCBI database and subjected to multiple sequence alignment using DNAMAN. The neighbor-joining algorithm of MEGA 7.0 was used to construct a phylogenetic tree of the STPK proteins .Citation28
The secondary structure of the LsSTPK protein was predicted by SOPMA, and the three-dimensional structure was predicted using SWISS-MODEL software.
Expression analysis of the LsSTPK gene
qRT-PCR was performed using cDNA templates to detect the expression level of the LsSTPK gene in lettuce. The lettuce 18S rRNA gene (GenBank accession number HM047292.1) was used as a loading control. The specific STPK-Q-F and STPK-Q-R primer sequences were used for qPCR analysis. The reaction system (10 μL) consisted of 2× SYBR qPCR Mix (5 μL), cDNA template (1 μL), 10 μM forward primer (0.5 μL), 10 μM reverse primer (0.5 μL) and ddH2O (3 μL). The program involved predenaturation at 94°C for 3 min followed by 39 cycles of 94°C for 10 s and 55°C for 30 s. The 2-ΔΔCT relative quantitation method was used to quantify the specific mRNA level.
VIGS
Two designed primers, STPK-V-F and STPK-V–R, targeting a 300-500 bp fragment in the open reading frame of LsSTPK were selected. The fragment was then cloned from the ‘GB30ʹ leaf lettuce cultivar. The pTRV2 empty vector was digested with the EcoRI and BamHI restriction enzymes. The sequence fragment obtained from cloning was inserted into the pTRV2 vector using T4 ligase followed by transformation into Escherichia coli for positive colony screening. PCR identification and sequencing were then performed. The identified recombinant plasmid was subsequently transferred into Agrobacterium GV3101, which was then prepared in infection solution.
‘GB30ʹ plants that had four true leaves were selected for the experiment and divided into the following three groups: 1) a blank control group, in which the plants received no injection; 2) a negative control group, in which pTRV2 and pTRV1 empty vectors were mixed at a 1:1 ratio and injected into the plants; and 3) an experimental group, in which LsSTPK-pTRV2 and pTRV1 empty vectors were mixed at a 1:1 ratio and injected into the bottoms of leaves using a sterilized syringe. The plants were then cultivated in an intelligent growth chamber with the following conditions: 20/13°C (day/night) temperature, 14/10 h (day/night) photoperiod and 60% relative humidity. At three weeks after injection, the plants were transferred to a new growth chamber (day/night temperatures of 33/25°C) for high-temperature treatment, and the other growth conditions were unchanged. Plant morphological changes were monitored, including weekly measurements of stem length. After 1 week of high-temperature treatment, new leaves that grew after the injection were randomly selected and then tested using the characteristic TRV primers () to determine whether the TRV virus had been transferred into the plants. Three plants were then tested to determine the relative expression of LsSTPK using qPCR and to determine the contents of GA3, IAA and abscisic acid (ABA) in new leaves using an enzyme-linked immunosorbent assay (ELISA). The testing kit was provided by China Agricultural University. At the same time, the flower buds were observed via paraffin methods .Citation29
Statistical analysis
All tests were performed in three replicates. For expression analyses, three different roots, stems or leaves were pooled together as one biological sample, and this was done three times to produce three independent biological replicates (of three pooled roots, stems or leaves) for expression analysis. For the observation of flower bud differentiation, each biological replicate had five samples from five plants. The data represent the means ± SD of three replications. Microsoft Office Excel 2016 was used for data processing. The data were analyzed by one-way ANOVA and Duncan’s multiple comparison analysis using SPSS 22.0 software (International Business Machine, Chicago, IL, USA). OriginPro 9.0 software (Origin Lab, Northampton, MA, USA) was used to generate the graphs.
Results
Cloning and sequence analysis of LsSTPK
The complete open reading frame sequence of the STPK gene (GeneID: 111,903,985) was cloned from lettuce stems by RT-PCR. The gene, which we defined as LsSTPK, had the highest homology with Helianthus annuus STPK (XP_022036020.1). LsSTPK has a complete open reading frame that comprises 1095 bp () and encodes a protein of 365 amino acids ().
Figure 1. Bioinformatics analysis of the LsSTPK gene. (a) 1, 2 and 4:LsSTPK; 3, no template negative control. (b) Nucleotide and amino acid sequences of LsSTPK. (c) The model of the secondary structure of the LsSTPK protein. H, α-Helix; e, Extended strand; t, β turn; c, Random coil (d) Tertiary structure prediction of LsSTPK. (e) Phylogenetic analysis of LsSTPK. (f) Conserved domain of the LsSTPK protein. (g) Multiple sequence alignment of STPK
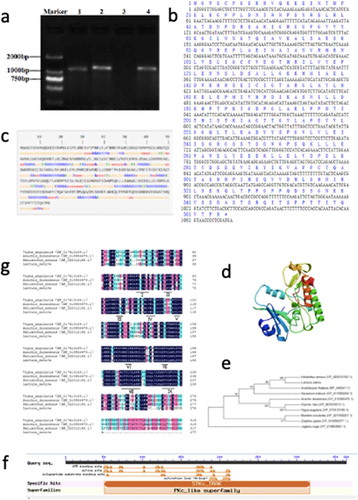
The deduced molecular weight of LsSTPK is 40.76 kDa, and the isoelectric point (pI) is 8.16. LsSTPK has an instability index of 42.99, which indicates that the protein is an unstable protein, and has an average hydrophilicity coefficient (GRAVY) of −0.231, which indicates that the protein is predicted to be hydrophilic. The subcellular structure prediction results showed that the LsSTPK protein may be located in the nucleus. The LsSTPK protein mainly consists of 163 random coils, 121 α-helixes, 59 extended strands and 21 β-turns (). The three-dimensional structure of LsSTPK was predicted by SWISS-MODEL software ().
Phylogenetic domains and conservation analysis of LsSTPK
The LsSTPK amino acid sequence obtained was aligned with other STPK sequences. LsMAPK4 exhibited a high degree of homology with the STPK protein from 9 plant species, including Helianthus annuus, Vigna angularis and Arabidopsis thaliana. Both lettuce and Helianthus annuus are members of the Asteraceae family with a close degree of homology followed by Arabidopsis and Sesamum indicum ().
By using NCBI CD Search to analyze the conserved structure of the LsSTPK protein, we found that the protein had a complete conserved plant protein kinase domain (). The kinase activity sites of this protein include an ATP-binding site and a substrate-binding site. In addition, the protein also contains an activating loop (A loop).
LsSTPK proteins are highly similar to STPK proteins of other species. LsSTPK has 9 conserved catalytic domains of STPKs ().
Analysis of LsSTPK expression in leaf lettuce
shows that the LsSTPK expression levels in the roots and leaves were similar, whereas the expression level in the stem was significantly greater than that in the roots and leaves. Therefore, we speculated that LsSTPK might play a role in stem development.
Figure 2. Expression pattern of LsSTPK. (a) Expression of LsSTPK in different tissues detected by qRT-PCR. (b-c) Expression analysis of LsSTPK in lettuce after high-temperature treatment. Error bars represent ±SD. Different small letters indicate significant difference at P < .05, and different capital letters indicate significant difference at P < .01 (n = 3)
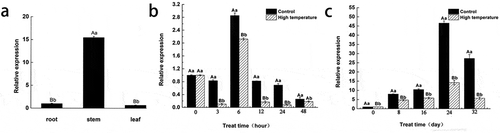
shows the variations in the relative expression of LsSTPK in leaf lettuce at different treatment times. The relative expression of LsSTPK in all three test groups sequentially decreased, increased and then decreased within the first 48 h of treatment. The relative expression of LsSTPK peaked after 6 h of treatment, and the relative expression in the experimental group was significantly lower than that in the other two groups.
The relative expression of LsSTPK in all three groups first increased and then decreased from day 8 to day 32 of treatment. The relative expression of LsSTPK peaked on day 24 of treatment, and the relative expression in the experimental group was significantly lower than that in the other two groups (). In summary, the high-temperature treatment significantly changed the relative expression of LsSTPK in leaf lettuce, which was lower in the experimental group than in the other two groups.
VIGS
Vector construction and Agrobacterium transformation
A 379-bp fragment was amplified using the characteristic LsSTPK primers and the cDNA of leaf lettuce as a template. The sequencing result and the sequence of LsSTPK were 100% homologous. The amplified fragment was the only fragment required for vector construction. The plasmid obtained from cloning and a pTRV2 empty vector were treated separately using the double-digest method and fused by T4 ligase. The EcoRI and BamHI sites were verified using the double-digest method to confirm that the recombinant plasmid contained the LsSTPK fragment. The positive plasmid was subsequently transferred into Agrobacterium GV3101, and PCR verification was performed using the characteristic primers of the LsSTPK fragment. A fragment approximately 400 bp in length was obtained, indicating that the expression vector was successfully transferred into Agrobacterium GV3101.
Molecular assays
PCR amplification was performed using the characteristic TRV primers and cDNA of new leaves as a template from the three testing groups. Bands of approximately 200 and 600 bp were identified in plants infected with empty and recombinant vectors, respectively (). No band was detected in the leaves of plants that had not been infected with the TRV viruses. These findings indicated that the plants in the negative control and experimental groups were successfully infected with TRV viruses.
Figure 3. Functional analysis of LsSTPK associated with bolting. (a) RT-PCR detection of TRV RNA in upper leaves. 1, Negative control; 2, Positive. (b) Expression of LsSTPK in lettuce leaves after gene silencing. Different small letters indicate significant difference at P < .05, and different capital letters indicate significant difference at P < .01 (n = 3). (c) The stems of the three groups of lettuce were elongated after high-temperature treatment. Different small letters indicate significant difference at P < .05, and different capital letters indicate significant difference at P < .01 (n = 3). (d) Stem length of lettuce after 4 weeks of infection. A, Positive; B, Negative control; C, Blank control. (e) Paraffin section of lettuce shoot tips. A, Blank control; B, Negative control; C, Positive. (f) The contents of endogenous hormones. Different small letters indicate significant difference at P < .05, and different capital letters indicate significant difference at P < .01 (n = 3)
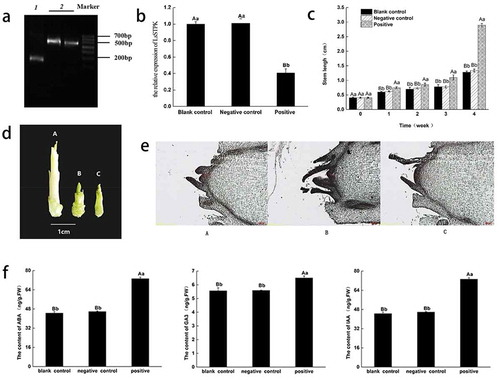
Analysis of LsSTPK expression in virus-infected leaf lettuce plants
The relative expression of LsSTPK in plants from the three test groups after 1 week of high-temperature treatment is shown in . The LsSTPK expression in the experimental group after 1 week of high-temperature treatment was 60% lower than that in the blank control and negative control groups.
Phenotypic characteristics and flower bud differentiation of virus-infected leaf lettuce plants
One week after infection, the stems in the negative control group were slightly longer than those in the blank control group with no significant difference between the two groups. Furthermore, the stems in the experimental group significantly differed from those in the other two groups (). One week after the high-temperature treatment, the stems in all three groups were elongated, and the difference in stem length between the experimental group and the other two groups was significant. Therefore, we speculated that the gene silencing treatment inhibited the expression of LsSTPK, resulting in elongation of the stems of leaf lettuce (). Thus, LsSTPK may play an important role in the bolting of leaf lettuce under high-temperature conditions. shows that the stem apical meristem of the two control groups was conical, while the stem apical meristem of the positive plants was flat and hemispherical, indicating that the buds of the positive plants had begun to differentiate.
Determination of endogenous hormone contents
shows that there was no significant difference in the contents of IAA, GA3 or ABA between the plants in the blank control group and the negative control group, but the contents of IAA, GA3 and ABA in the experimental group were significantly greater than those in the other two groups. These findings suggested that LsSTPK silencing increases the contents of endogenous hormones (IAA, GA3 and ABA) in leaf lettuce leaves.
Discussion
Plants need to adapt to changes in the external environment to maintain normal growth and development, and they have developed integrated regulatory mechanisms as a result of evolution. Protein phosphorylation is an important mechanism for plants to regulate their response to external signals. By catalyzing functional proteins, STPK, an important type of protein kinase, is involved in the biological activities of organisms. For example, STPK in peanut (Arachis hypogaea) is involved in the transduction of environmental stress signals, Citation30,Citation31 and mitogen-activated protein kinase (MAPK) cascade transduction pathways have important roles in growth factor regulation, hormone responses, cell proliferation and cell differentiation .Citation32
In the present study, the specific STPK gene, named LsSTPK, was obtained from leaf lettuce by cloning and verified by sequence analysis. We found that the LsSTPK protein retains a conservative structure in many plant species and has a complete STPK motif. According to subcellular location prediction, this protein is located in the nucleus. Analysis of LsSTPK gene expression in different organs suggested that the gene plays a role in stem development, and the qRT-PCR results showed that temperature affected the bolting of leaf lettuce and significantly affected the expression of LsSTPK ().
When LsSTPK was silenced via VIGS, the leaf lettuce stems in the experimental group were longer than those in the other two groups, and flower bud differentiation began sooner. Moreover, the IAA content in the leaves after LsSTPK gene silencing was greater than that in the other two groups. These results were consistent with those in the literature, in which NPK1, a specific STPK, is suggested to play a role in the negative modulation of IAA signal transduction .Citation22 After LsSTPK silencing, the contents of GA3 and ABA in the leaves of leaf lettuce in the experimental group were greater than those in the other two groups. Previous studies have shown that IAA is beneficial to flower bud differentiation and bolting. Suggested that ABA could inhibit Arabidopsis flowering. Later, some studies showed that abscisic acid sprayed on leaves of strawberry could make it bloom, but ABA could inhibit the flowering of spinach .Citation33 Therefore, LsSTPK may be related to the bolting of leaf lettuce under high-temperature conditions, and silencing of the LsSTPK gene promotes bolting. This phenomenon may be explained by the following mechanism: under high-temperature conditions, LsSTPK expression decreases to regulate the phosphorylation of relevant proteins, thereby regulating the levels of hormones (IAA, GA3 and ABA) in plants, which in turn results in leaf lettuce bolting.
Conclusions
In the present paper, the LsSTPK gene was isolated from leaf lettuce, and its expression was inhibited by high temperature. Following LsSTPK silencing using the VIGS method, the stem length of the treated group was significantly longer than that in the blank and negative control groups, and the contents of IAA, GA3 and ABA in the treated group were higher than those in the other two groups. Flower bud differentiation appeared in the treatment group but not in the control group. The above findings suggested that LsSTPK inhibits the bolting of leaf lettuce under high-temperature conditions.
Author contributions
Conceptualization, J.H. and L.W.; methodology and formal analysis, L.W., Y.W. and W.D.; investigation and resources, L.W., Y.W., Z.Y., Z.Q. and W.T.; writing—original draft preparation, L.W.; writing—review and editing, Y.H. and C.L.; project administration and funding acquisition, S.F. and J.H. All authors have read and agreed to the published version of the manuscript.
Acknowledgments
This study was supported by the National Natural Science Foundation of China (Grant No. 31972400); the 2018 Joint Funding Project of Beijing Natural Science Foundation-the Municipal Education Commission (KZ201810020027); Beijing Leafy Vegetables Innovation Team of Modern Agro-industry Technology Research System (BAIC07-2021); and the Construction of Beijing Science and Technology Innovation and Service Capacity in Top Subjects (CEFF-PXM2019_014207_000032).
Disclosure statement
No potential conflict of interest was reported by the author(s).
Additional information
Funding
References
- Atsushi Y, Kazuo T, Kazuo T, Hiroyuki M. 2000. Effects of day and night temperatures on flower-bud formation and bolting of Japanese bunching onion(Allium fistulosum L.). Engei Gakkai Zasshi. 69(1): 1–8. doi:10.2503/jjshs.69.40.
- Han YY, Fan SX, Zhang Q, Wang YN. 2013. Effect of heat stress on the MDA, proline and soluble sugar content in leaf lettuce seedlings. Agric Sci. 4: 112–115.
- Santamaria P. 2006. Nitrate in vegetables: toxicity, content, intake and EC regulation. J Sci Food Agric. 86(1): 10–17. doi:10.1002/jsfa.2351.
- Kim MJ, Tou JC, Mou B, Waterland NL, Waterland NL. 2016. Nutritional value, bioactive compounds and health benefits of lettuce (Lactuca sativa L.). J Food Compos Anal. 49: 10–34. doi:10.1016/j.jfca.2016.03.004.
- Jenni S, Truco MJ, Michelmore RW. 2013. Quantitative trait loci associated with tipburn, heat stress-induced physiological disorders, and maturity traits in crisphead lettuce. Theor Appl Genet. 126(12): 3065–3079. doi:10.1007/s00122-013-2193-7.
- Liu XY, Liu R, Huang Q, Liu CJ, Fan SX, Han YY (2018) Effects of morphological characterization in different bolting period of lettuce, International Conference on Biological Sciences and Technology, 316–319. doi:10.2991/bst-17.2018.50
- Pajoro A, Biewers S, Dougali E, Leal Valentim F, Mendes MA, Porri A, Coupland G, Van De Peer Y, Van Dijk AD, Colombo L, et al. 2014. The revolution of gene regulatory networks controlling Arabidopsis plant reproduction: a two-decade history. J Exp Bot. 65(17): 4731–4745. doi:10.1093/jxb/eru233.
- Naoko K, Susumu Y, Kazuhiro N, Yoshihito T, Shuji Y. 2014. A naturally occurring long insertion in the first intron in the Brassica rapa FLC2 gene causes delayed bolting. Euphytica1. 96:213–223. doi:10.1007/s10681-013-1025-9
- Lim SH, Song JH, Kim DH, Kim JK, Lee JY, Kim YM, Ha SH. 2016. Activation of anthocyanin biosynthesis by expression of the radish R2R3-MYB transcription factor gene RsMYB1. Plant Cell Rep. 35(3): 641–653. doi:10.1007/s00299-015-1909-3.
- Kempa S, Rozhon W, Samaj J, Erban A, Baluska F, Becker T, Haselmayer J, Schleiff E, Kopka J, Hirt H, et al. 2007. A plastid-localized glycogen synthase kinase 3 modulates stress tolerance and carbohydrate metabolism. Plant J. 49(6): 1076–1090. doi:10.1111/j.1365-313X.2006.03025.x.
- Bentemde SDLFV, Hirt H. 2007. Using phosphoproteomics to reveal signalling dynamics in plants. Trends Plant Sci. 9: 404–411.
- Polge C, Thomas M. 2007. SNF1/AMPK/SnRK1 kinases, global regulators at the heart of energy control? Trends Plant Sci. 12: 20–28. doi:10.1016/j.tplants.2006.11.005.
- Inzé D, De VL. 2006. Cell cycle regulation in plant development. Annu Rev Genet. 40(1): 77–105. doi:10.1146/annurev.genet.40.110405.090431.
- Sasabe M, Machida Y. 2006. MAP65: a bridge linking a MAP kinase to microtubule turnover. Curr Opin Plant Biol. 9(6): 563–570. doi:10.1016/j.pbi.2006.09.010.
- Harper JF, Harmon A. 2005. Plants, symbiosis and parasites: a calcium signalling connection. Nat Rev Mol Cell Biol. 6(7): 555–566. doi:10.1038/nrm1679.
- Takahashi F, Yoshida R, Ichimura K, Mizoguchi T, Seo S, Yonezawa M, Maruyama K, Yamaguchi-Shinozaki K, Shinozaki K. 2007. The mitogen-activated protein kinase cascade MKK3–MPK6 is an important part of the jasmonate signal transduction pathway in Arabidopsis. Plant Cell. 19(3): 805–818. doi:10.1105/tpc.106.046581.
- Alderwick LJ, Molle V, Kremer L, Cozzone AJ, Dafforn TR, Besra GS, Fütterer K. 2006. Molecular structure of EmbR, a response element of Ser/Thr kinase signaling in mycobacterium tuberculosis. Proc Natl Acad Sci U S A. 103: 2558–2563.
- Faucher SP, Viau C, Gros PP, Daigle F, Le MH. 2008. The prpZ gene cluster encoding eukaryotic-type Ser/Thr protein kinases and phosphatases is repressed by oxidative stress and involved in Salmonella enterica serovar Typhi survival in human macrophages. FEMS Microbiol Lett. 281(2): 160–166. doi:10.1111/j.1574-6968.2008.01094.x.
- Pereira SF, Goss L, Dworkin J. 2011. Eukaryote-like serine/threonine kinases and phosphatases in bacteria. Microbiol Mol Biol R. 75(1): 192–212. doi:10.1128/MMBR.00042-10.
- Mu JH, Lee HS, Kao TH. 1994. Characterization of a pollen-expressed receptor-like kinase gene of Petunia inflata and the activity of its encoded kinase. Plant Cell. 6(5): 709–721. doi:10.1105/tpc.6.5.709.
- Claisse G, Charrier B, Kreis M. 2007. The Arabidopsis thaliana GSK3/Shaggy like kinase AtSK3-2 modulates floral cell expansion. Plant Mol Biol. 64(1–2): 113–124. doi:10.1007/s11103-007-9138-y.
- Kovtun Y, Chiu WL, Zeng W, Sheen J. 1998. Suppression of auxin signal transduction by a MAPK cascade in higher plants. J Sheen Nature. 395(6703): 716–720. doi:10.1038/27240.
- Zhang J, Nodzynski T, Pencík A, Rolcík J, Friml J. 2010. PIN phosphorylation is sufficient to mediate PIN polarity and direct auxin transport. Natl Acad Sci U S A. 107(2): 918–922. doi:10.1073/pnas.0909460107.
- Sharma A, Komatsu S. 2002. Involvement of a Ca2+-dependent protein kinase component downstream to the gibberellin-binding Phosphoprotein, RuBisCO activase, in rice. Biochem Biophys Res Commun. 290(2): 690–695. doi:10.1006/bbrc.2001.6269.
- Wilkins MR, Gasteiger E, Bairoch A, Sanchez JC, Williams KL, Appel RD, Hochstrasser DF. 1999. Protein identification and analysis tools in the ExPASy server. Methods Mol Biol. 112: 531–552. doi:10.1385/1-59259-584-7:531.
- Marchler-Bauer A, Bo Y, Han L, He J, Lanczycki CJ, Lu S, Chitsaz F, Derbyshire MK, Geer RC, Gonzales NR, et al. 2017. CDD/SPARCLE: functional classification of proteins via subfamily domain architectures. Nucleic Acids Res. 45(D1): D200–D203. doi:10.1093/nar/gkw1129.
- Chou KC, Shen HB. 2008. Cell-PLoc: a package of web servers for predicting subcellular localization of proteins in various organisms. Nat Protoc. 3(2): 153–162. doi:10.1038/nprot.2007.494.
- Kumar S, Stecher G, Tamura K. 2016. MEGA7: molecular evolutionary genetics analysis version 7.0 for bigger datasets. Mol Biol Evol. 33(7): 1870–1874. doi:10.1093/molbev/msw054.
- Zhang YM, Ma HL, Calderón-Urrea A, Tian CX, Bai XM, Wei JM. 2016. Anatomical changes to protect organelle integrity account for tolerance to alkali and salt stresses in Melilotus officinalis. Plant Soil. 406(1–2): 327–340. doi:10.1007/s11104-016-2875-4.
- Rudrabhatla P, Rajasekharan R. 2002. Developmentally regulated dual-specificity kinase from peanut that is induced by abiotic stresses. Plant Physiol. Vol. 130: 380–390. doi:10.1104/pp.005173
- Rudrabhatla P, Rajasekharan R. 2003. Mutational analysis of stress-responsive peanut dual specificity protein kinase. Identification of tyrosine residues involved in regulation of protein kinase activity. J Biol Chem. 278(19): 17328–17335. doi:10.1074/jbc.M300024200.
- Mizoguchi T, Ichimura K, Shinozaki K. 1997. Environmental stress response in plants: the role of mitogen-activated protein kinases. Trends Biotechnol. 15(1): 15–19. doi:10.1016/S0167-7799(96)10074-3.
- Bernier G, Havelange A, Houssa C, Petitjean A, Lejeune P. 1993. Physiological signals that induce flowering. Plant Cell. 5(10): 1147–1155. doi:10.2307/3869768.