ABSTRACT
Inhibition of primary root (PR) growth is a typical developmental response of Arabidopsis to phosphate (Pi) deficiency. Functional disruption of SIZ1, a SUMO E3 ligase, is known to enhance the Pi deficiency-induced inhibition of PR growth. The molecular mechanism of how SIZ1 regulates PR growth under Pi deficiency, however, remains unknown. SIZ1 was recently reported to partially SUMOylate STOP1, a transcription factor that functions in plant tolerance to aluminum toxicity and in plant responses to Pi deficiency by regulating the expression of ALMT1. ALMT1 encodes an aluminum-activated malate transporter, and its expression is induced by Pi deficiency. In siz1, the expression of ALMT1 is enhanced and the removal of Fe from Pi-deficient medium suppressed the siz1 mutant phenotype. In this report, we show that siz1 overaccumulates Fe in its root apoplasts, and consequently, produces more hydroxyl radicals, which are detrimental to root growth. Such physiological changes in siz1 can be completely suppressed by the mutation of STOP1 or ALMT1. Based on previously published work and the results of the current study, we propose that SIZ1 regulates Pi deficiency-mediated PR growth through modulating the accumulation of Fe and the production of hydroxyl radicals by controlling ALMT1 expression.
When plants encounter phosphate (Pi) deficiency in the environment, their roots exhibit an array of biochemical, physiological, and developmental responses.Citation1 These responses include a remodeling of root system architecture (RSA), an increase in the activity of Pi transporters located in the plasma membranes of root cells, and the enhanced synthesis and secretion of acid phosphatases, nucleases, and organic acids (such as malate and citrate). Such adaptive responses enable plants to better survive and grow under Pi deficiency.
RSA, i.e., “the spatial configuration of the root system”, has a profound effect on a plant’s ability to acquire water and nutrients.Citation2 The pattern of remodeling of RSA for a given species depends on many factors and varies from species to species.Citation3 In the past decades, the model plant Arabidopsis has been extensively used to investigate the molecular mechanisms that regulate the remodeling of RSA. When grown in a Pi-deficient (–Pi) medium in Petri dishes, Arabidopsis seedlings show an inhibition of primary root (PR) growth and an enhanced production of lateral roots.Citation3 Pi deficiency also causes plants to accumulate high levels of Fe in roots,Citation4,Citation5 and the presence of Fe in the – Pi medium is essential for the inhibition of PR growth.Citation6,Citation7 Many Arabidopsis mutants that display enhanced or reduced sensitivity to Pi deficiency in terms of their PR growth have been isolated, and the genes responsible for the mutant phenotypes have been identified.Citation3 By studying a group of mutants that affect Fe accumulation in root apoplasts and the secretion of malate into the rhizosphere, researchers have elucidated the molecular mechanism of Pi deficiency-induced inhibition of PR growth of Arabidopsis. LPR1 encodes a ferroxidase that converts Fe2+ to Fe3+.Citation8 Mutation of LPR1 and its close homolog LPR2 reduces the accumulation of Fe3+ in root apoplasts and makes plants insensitive to Pi deficiency-induced inhibition of PR growth.Citation7,Citation8 Similarly, mutations of ALMT1 (an aluminum-activated malate transporter) and STOP1 (a transcription factor that directly regulates the transcription of ALMT1) also reduces the accumulation of Fe3+ in root apoplasts and causes PR growth to be insensitive to Pi deficiency.Citation9–11 In contrast, the als3 or star1 mutant overaccumulates Fe3+ in the root apoplasts and is hypersensitive to Pi deficiency-induced inhibition of PR growth.Citation12 ALS3 and STAR1 encode a transmembrane domain and nucleotide-
binding domain, respectively, of a putative ABC transporter complex located in tonoplasts. Researchers further demonstrated that Pi deficiency-induced inhibition of Arabidopsis PR growth is caused by a blue light-mediated Photo-Fenton reaction and by a canonical Fenton reaction in root apoplasts.Citation13 In these reactions, malate reacts with Fe to produce hydroxyl radicals (a type of reactive oxygen species, ROS), which is detrimental to cell activity, therefore inhibiting PR growth. This mechanism was also supported by two recent publications which showed a positive correlation between the levels of Fe accumulation in roots and the degree of Pi deficiency-induced inhibition of PR growth using the Arabidopsis knockout mutants mpk6 and mediator16 .Citation14,Citation15
SUMOylation is an important protein modification process. It transfers a small peptide (small ubiquitin-related modifier, SUMO) to its target proteins, which alters protein stability and function. The SUMO E3 ligase catalyzes the last step of attaching the SUMO peptide to the target proteins.Citation16 SIZ1 is a SUMO E3 ligase which, when mutated, enhances Pi deficiency-induced inhibition of Arabidopsis PR growth.Citation17 In vitro biochemical study indicated that SIZ1 could SUMOylate the transcription factor PHR1, which is a master regulator of plant transcriptional responses to Pi deficiency.Citation18,Citation19 However, the PR growth of phr1 was similar to that of the WT under Pi deficiency and the transcription of STOP1 and ALMT1 was not affected in phr1phl1 double mutant (PHL1 is a close homolog of PHR1 in Arabidopsis),Citation9,Citation19 indicating that it is unlikely that SIZ1 regulates Pi deficiency-induced inhibition of PR growth by SUMOylating PHR1.
Recently, Xu et al. (2021) and Fang et al. (2021) independently reported that SIZ1 could SUMOylate STOP1 and that the siz1 mutant had an enhanced expression of ALMT1.Citation20,Citation21 These researchers further showed that the enhanced inhibition of PR growth of siz1 could be suppressed by the mutation in STOP1 or ALMT120 or by the removal of Fe from the – Pi medium.Citation21 These findings suggested that SIZ1 acts through STOP1 to regulate PR growth under Pi deficiency by affecting the expression of ALMT1 and that the hypersensitive PR growth phenotype of siz1 is linked to Fe in – Pi media.
In the current study, we first reexamined the PR growth phenotype of the WT, siz1, stop1, almt1, siz1stop1, and siz1almt1 on both +Pi and – Pi media (). The results obtained were consistent with those previously reported.Citation17,Citation20 We then analyzed the patterns of Fe accumulation in roots in the WT and the various mutants using Perls staining and Perls/DAB (diaminobenzidine) staining. The Perls method mainly stains Fe3+, and the Perls/DAB method stains both Fe2+ and Fe3+. The two methods revealed similar staining patterns. On +Pi medium, the level of Fe accumulation in roots did not differ between the WT and various mutants; on – Pi medium, however, siz1 roots accumulated more Fe and stop1 and almt1 roots accumulated less Fe than WT roots (). Moreover, the levels of Fe accumulation in siz1stop1 and siz1almt1 double mutants were similar to those in stop1 and almt1. Finally, we found that under Pi deficiency, the levels of hydroxyl radicals (a type of reactive oxygen species, ROS) produced in different genotypes were positively correlated with the levels of Fe accumulation in roots (). Such physiological changes in siz1 could be completely suppressed by the mutation of STOP1 or ALMT1. Considering that the overexpression of STOP1 or ALMT1 caused overaccumulation of Fe and enhanced inhibition of PR growth induced by Pi deficiency,Citation11 as well as the results from Xu et al. (2021)Citation20 and Fang et al. (2021)Citation21 and the current research, we conclude that the mutation of SIZ1 enhances the expression of ALMT1, which in turn increases Fe accumulation in root apoplasts. The overaccumulation of Fe results in an overproduction of hydroxyl radicals and, consequently, an increased inhibition of PR growth. We therefore propose that SIZ1 regulates Pi deficiency-induced inhibition of PR growth by SUMOylating STOP1, which alters expression of ALMT1 and the accumulation of Fe and ROS in roots.
Figure 1. Growth phenotypes and Fe accumulation patterns of the WT and various mutants. (a) Morphologies of 8-day-old seedlings of the WT and various mutants grown in +Pi or – Pi medium. Scale bar = 1 cm. (b and c) Perls staining (b) and Perls/DAB staining (c) of Fe accumulation patterns of the WT and various mutants corresponding to the seedlings shown in (a). Ten seedlings for each genotype were analyzed and the roots for representative seedlings were shown. The experiments were repeated three time with similar results. Scale bar = 100 μm
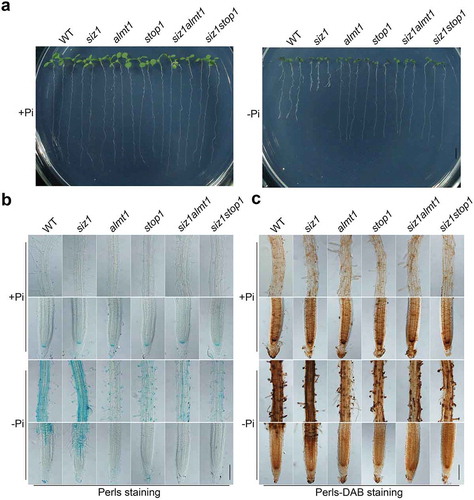
Figure 2. Hydroxyl radical accumulation in roots of the WT and various mutants. (a) The levels of hydroxyl radicals in roots of 8-day-old seedlings of the WT and various mutants grown in +Pi or – Pi medium assessed by HPF staining. (b) Quantification of HPF fluorescent signals in the root meristematic and maturation zones shown in (a). Values are means with SD (n = 6 roots). The experiments were repeated three time with similar results. Asterisks indicate significant differences from the WT (t-test, p < .05)
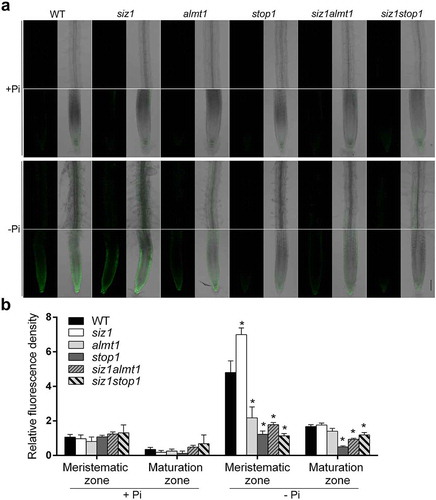
Materials and methods
Plant materials and growth conditions
All Arabidopsis plants used in this study were in the Columbia-0 background. The T-DNA insertion lines siz1 (SALK_065397), stop1 (SALK_114108), and almt1 (SALK_ 009629) and the double mutants derived from genetic crossing were previously describeCitation20 and kindly provided by Dr. Huiyu Tian.
Arabidopsis seeds were surface sterilized in 20% (v/v) bleach for 10 min and were then washed three times with sterile-distilled water (ddH2O). After being stratified at 4°C for 2 days, the seeds were sown on Petri plates containing a Pi-sufficient (+Pi) medium or a Pi-deficient (-Pi) medium. The standard +Pi medium was half-strength Murashige and Skoog (MS) medium with 1% (w/v) sucrose, 0.1% (w/v) MES, and 1.2% (w/v) agar (Sigma-Aldrich, catalog no. A1269). The +Pi medium contained 625 μM KH2PO4 and 100 μM Fe-EDTA per liter. For the -Pi medium, KH2PO4 in the +Pi medium was replaced with K2SO4. The pH was adjusted to 5.8 for both +Pi and -Pi media. The plates with seeds were placed vertically in a growth room with a photoperiod of 16 h of light and 8 h of dark at 22–24°C. The light intensity was 100 µmol m–2 s–1.
Perl and Perls/DAB staining assays
Perls staining was performed as previously described with minor modifications.Citation22 Briefly, the roots were excised from 8-day-old seedlings and directly submerged into the staining solution of a Perls stain kit (Solarbio). After 30 min in the staining solution, the samples were rinsed with ddH2O three times.
Perls/DAB staining was carried out as described by Balzergue et al (2017).Citation9 Perls-stained samples were incubated for 60 min in methanol containing 0.01 M NaN3 and 0.3% H2O2 (30%). The samples were then washed with 0.1 M Na-phosphate buffer (pH 7.2) and incubated for 5 min in the same buffer containing 0.025% (w/v) DAB (Sigma-Aldrich) and 0.005% H2O2. The samples were washed three times with phosphate buffer to stop the reaction.
The roots stained by both Perls and Perls/DAB methods were stored in 0.1 M Na-phosphate buffer (pH 7.4) before being photographed. All stained samples were cleared on glass slides with a 80% HCG clearing solution (80% (v/v) chloral hydrate glycerol
diluted with 0.1 M Na-phosphate buffer, pH 7.4). The Fe staining patterns in roots were examined using a 20x objective with a differential interference contrast microscope (Olympus BX51) equipped with a camera (Olympus DP71).
Histochemical detection of OH in roots
·OH in roots was detected using the HPF (hydroxylphenyl fluorescein) staining method as previously described.Citation13 Roots were excised from 8-d-old seedlings and were pre-incubated in a 100 mM phosphate buffer (pH 6.1) for 20 min. The samples were then transferred to the same buffer containing 10 µM HPF, and the preparation was incubated for another 30 min. The fluorescent signals representing ·OH were observed with an LSM710 confocal microscope (Zeiss). The excitation and emission wavelengths used for detection of the signals were 490 nm and 520 nm, respectively. The images were processed and analyzed using Zen Blue software.
Acknowledgments
We thank Dr. Huiyu Tian of Shandong University for kindly providing the mutant seeds. This work was supported by the funds from the Ministry of Science and Technology of China (grant no. 2016YFD0100700) and the National Natural Science Foundation of China (grant no. 32070298).
Disclosure statement
No potential conflict of interest was reported by the author(s).
Additional information
Funding
References
- López‐Arredondo DL, Leyva‐González MA, González‐Morales SI, López‐Bucio J, Herrera‐Estrella L Phosphate nutrition: improving low‐phosphate tolerance in crops. Annu Rev. Biol P. 2014;65:1–5. doi:10.1146/annurev-arplant-050213-035949
- Lynch J. Root architecture and plant productivity. Plant Physiol. 1995;109(1):7–13. doi:10.1104/pp.109.1.7.
- Liu D. Root developmental responses to phosphorus nutrition. J Integr Plant Biol. 2021 Mar 12;63(6):1065–1090. Online ahead of print. doi:10.1111/jipb.13090.
- Hirsch J, Marin E, Floriani M, Chiarenza S, Richaud P, Nussaume L, Thibaud MC. Phosphate deficiency promotes modification of iron distribution in Arabidopsis plants. Biochimie. 2006;88(11):1767–1771. doi:10.1016/j.biochi.2006.05.007.
- Misson J, Raghothama KG, Jain A, Jouhet J, Block MA, Bligny R, Ortet P, Creff A, Somerville S, Rolland N, et al. A genome-wide transcriptional analysis using Arabidopsis thaliana Affymetrix gene chips determined plant responses to phosphate deprivation. Proc Natl Acad Sci USA. 2005;102(33):11934–11939. doi:10.1073/pnas.0505266102.
- Ward JT, Lahner B, Yakubova E, Salt DE, Raghothama KG. The effect of iron on the primary root elongation of Arabidopsis during phosphate deficiency. Plant Physiol. 2008;147(3):1181–1191. doi:10.1104/pp.108.118562.
- Svistoonoff S, Creff A, Reymond M, Sigoillot‐Claude C, Ricaud L, Blanchet A, Nussaume L, Desnos T. Root tip contact with low‐phosphate media reprograms plant root architecture. Nat Genet. 2007;39(6):792–796. doi:10.1038/ng2041.
- Müller J, Toev T, Heisters M, Teller J, Moore KL, Hause G, Dinesh DC, Bürstenbinder K, Abel S. Iron‐dependent callose deposition adjusts root meristem maintenance to phosphate availability. Dev Cell. 2015;33(2):216–230. doi:10.1016/j.devcel.2015.02.007.
- Balzergue C, Dartevelle T, Godon C, Laugier E, Meisrimler C, Teulon JM, Creff A, Bissler M, Brouchoud C, Hagège A, et al. Low phosphate activates STOP1‐ALMT1 to rapidly inhibit root cell elongation. Nat Commun. 2017;8(1):15300. doi:10.1038/ncomms15300.
- Mora‐Macías J, Ojeda‐Rivera JO, Gutiérrez‐Alanís D, Yong‐Villalobos L, Oropeza‐Aburto A, Raya‐González J, Jiménez‐ Domínguez G, Chávez‐Calvillo G, Rellán‐Álvarez R, Malate‐dependent HL. Fe accumulation is a critical checkpoint in the root developmental response to low phosphate. Proc Natl Acad Sci USA. 2017;114(17):E3563–E3572. doi:10.1073/pnas.1701952114.
- Wang X, Wang Z, Zheng Z, Dong J, Song L, Sui L, Nussaume L, Desnos T, Liu D. Liu D Genetic Dissection of Fe-Dependent Signaling in Root Developmental Responses to Phosphate Deficiency. Plant Physiol. 2019;179(1):300–316. doi:10.1104/pp.18.00907.
- Dong J, Piñeros MA, Li X, Yang H, Liu Y, Murphy AS, Kochian LV, Liu D. An Arabidopsis ABC transporter mediates phosphate deficiency‐induced remodeling of root architecture by modulating iron homeostasis in roots. Mol Plant. 2017;10(2):244–259. doi:10.1016/j.molp.2016.11.001.
- Zheng Z, Wang Z, Wang X, Liu D. Blue light‐triggered chemical reactions underlie phosphate deficiency‐induced inhibition of root elongation of Arabidopsis seedlings grown in Petri dishes. Mol Plant. 2019;12(11):1515–1523. doi:10.1016/j.molp.2019.08.001.
- López-Bucio JS, Salmerón-Barrera GJ, Ravelo-Ortega G, Raya-González J, León P, De La Cruz HR, Campos-García J, López-Bucio J, Guevara-García ÁA. Mitogen-activated protein kinase 6 integrates phosphate and iron responses for indeterminate root growth in Arabidopsis thaliana. Planta. 2019;250(4):1177–1189. doi:10.1007/s00425-019-03212-4.
- Raya-González J, Ojeda-Rivera JO, Mora-Macias J, Oropeza-Aburto A, Ruiz-Herrera LF, López-Bucio J, Herrera-Estrella L. MEDIATOR16 orchestrates local and systemic responses to phosphate scarcity in Arabidopsis roots. New Phytol. 2021;229(3):1278–1288. doi:10.1111/nph.16989.
- Jmii S, Cappadocia L. Plant SUMO E3 ligases: function, structural organization, and connection with DNA. Front Plant Sci. 2021:12. doi:10.3389/fpls.2021.652170.
- Miura K, Rus A, Sharkhuu A, Yokoi S, Karthikeyan AS, Raghothama KG, Baek D, Koo YD, Jin JB, Bressan RA, et al. The Arabidopsis SUMO E3 ligase SIZ1 controls phosphate deficiency responses. Proc Natl Acad Sci USA. 2005;102(21):7760–7765. doi:10.1073/pnas.0500778102.
- Rubio V, Linhares F, Solano R, Martín AC, Iglesias J, Leyva A, Paz‐Ares J. A conserved MYB transcription factor involved in phosphate starvation signaling both in vascular plants and in unicellular algae. Genes Dev. 2001;15(16):2122–2133. doi:10.1101/gad.204401.
- Bustos R, Castrillo G, Linhares F, Puga MI, Rubio V, Pérez‐Pérez J, Solano R, Leyva A, Paz‐Ares J, Ecker JR. A central regulatory system largely controls transcriptional activation and repression responses to phosphate starvation in Arabidopsis. PLoS Genet. 2010;6(9):e1001102. doi:10.1371/journal.pgen.1001102.
- Xu J, Zhu J, Liu J, Wang J, Ding Z, Tian H. SIZ1 negatively regulates aluminum resistance by mediating the STOP1–ALMT1 pathway in Arabidopsis. J Integr Plant Biol. 2021;63(6):1147–1160. doi:10.1111/jipb.13091.
- Fang Q, Zhang J, Yang DL, Huang CF. The SUMO E3 ligase SIZ1 partially regulates STOP1 SUMOylation and stability in Arabidopsis thaliana. Plant Signal Behav. 2021;16(5):1899487. doi:10.1080/15592324.2021.1899487.
- Roschzttardtz H, Conejero G, Curie C, Mari S. Identification of the endodermal vacuole as the iron storage compartment in the Arabidopsis embryo. Plant Physiol. 2019;51:1329–1338.