ABSTRACT
Sunflower (Helianthus annuus L.) is one of the major oilseed crops cultivated world over for its high-quality oil rich in linoleic acid. It also has established applications in pharmaceutical and biotechnological industries, mainly through recombinant production of unique oil body (OB) membrane proteins-oleosins, which are used for producing a wide variety of vaccines, food products, cosmetics and nutraceuticals. The present review provides a critical analysis of the progress made in advancing our knowledge in sunflower biology, ranging from mechanisms of pollen-stigma interaction, seed development, physiology of seed germination and seedling growth under salt stress, and finally understanding the signaling routes associated with various biochemical pathways regulating seedling growth. Role of nitric oxide (NO) triggered post-translational modifications (PTMs), discovered in the recent past, have paved way for future research directions leading to further understanding of sunflower developmental physiology. Novel protocols recently developed to monitor temporal and spatial distributions of various biochemicals involved in above-stated developmental events in sunflower, will go a long way for similar applications in plant biology in future.
Introduction
Sunflower (Helianthus annuus L.) is commercially one of the four most important annual edible oilseed crops cultivated worldwide, others being soybean, peanut and canola. Oil bodies (OBs) and associated intrinsic proteins (oleosins) offer large number of pharmaceutical and biotechnological applications for human welfareCitation1. The research group of the corresponding author of this publication (S C Bhatla) began using sunflower seedlings as a model system 35 years back by investigating the role of auxin/ethylene interactions in adventitious rooting (AR) from hypocotyl explants, and monitored the associated changes in the expression of peroxidase isozymes.Citation2 The work was further extended to examine the role of calcium in auxin-induced AR formation.Citation3 Soon thereafter, focus was shifted to understanding the biochemistry of OB mobilization in seedling cotyledons during early phase of seed germination.Citation4,Citation5 While undertaking these investigations for the next decade or so, novel protocols were also developed simultaneously to temporally and spatially localize the key enzymes, namely lipase, lipoxygenase, thiol protease and phospholipase A2, which are crucial for regulated enzymatic degradation of OB membrane constituents, followed by the hydrolysis of triacylglycerides (TAGs) from within the OB matrix for providing energy to the growing seedlings.Citation6–9 Keeping in view the ever-increasing abiotic stress conditions being faced by crops world over, investigations were simultaneously undertaken to understand the physiological and biochemical aspects of sunflower seedling growth under salt stress, and mechanisms of their photomodulation.Citation10,Citation11 Present review provides an update on the major findings from the corresponding author’s laboratory with regard to new findings on the mechanisms of floral induction, seed development, seed germination and salt stress tolerance in sunflower. This review also discusses about the novel protocols developed in Delhi University to decipher sunflower seedling growth in normal and salt stress conditions.
Biomolecules associated with pollen-stigma interaction
Angiosperm flowers have developed a variety of complex structures for attracting pollinators, leading to multiple ways for pollen-stigma interaction. Among the members of the family Asteraceae, reproductive output is further enhanced by the condensing of inflorescence. Sunflower inflorescence is a disc-shaped capitulum consisting of ovate involucre bracts, which function as sepals and protect the capitulum during its development. Development of sunflower inflorescence can be considered under three phases, namely inflorescence initiation, floret development and anther formation. Floret primordia appear at the rim of the receptacle where ray or disc florets are generated. Sunflower stigma is semi-dry in nature and undergoes physiological maturity from bud, staminate and finally to pistillate stageCitation12,Citation13 . The production of extracellular lipid rich secretions on stigma surface is initiated at the staminate stage of stigma development and it increases at the receptive stage due to enhanced availability of elaioplasts and endoplasmic reticulum network in the basal region of the papillae. Receptive stage of stigma begins when it is able to support pollen adhesion on its surface, followed by pollen hydration and subsequent formation of pollen tube. A number of biomolecules are required to enable pollen adhesion, hydration and germination on the stigma surfaceCitation12. Non-specific esterase activity is evident at all stages of stigma development until maturity. Serine esterases are associated with cutinase complex formation needed for pollen tube penetration in dry stigma. Removal of stigma surface components by chemical treatment reduces the ability of the pollen tube to penetrate stigma.Citation12
During the course of stigma development, there is ample accumulation of reactive oxygen species (ROS), NO and enhanced expression of the activities of ROS scavenging enzymes, principally superoxide dismutase (SOD) and peroxidase (POD). Gradual increase in ROS and NO in the stigmatic papillae is likely to provide enhanced immunity to the developing stigma, and they also serve as signaling molecules. An increase in total SOD activity at the staminate stage is followed by a peak of POD activity during pistillate stage, indicating the sequential action of these ROS scavenging enzyme.Citation12 Stigmas are known to provide nutrients to the pollen grains which are highly desiccated and metabolically inactive. Pollen coat contains essential components required for adhesion and cell to cell interaction between pollen and stigma. Sunflower pollen coat exhibits lipase activity and, interestingly, lipid profile of pollen fractions shows the absence of TAGs. It is likely that lipases in pollen coat are activated when pollen grains reach the stigma surface.Citation12 Pollen coat lipases may be involved in the degradation of lipids present in the stigmatic tissue. There are also some other biochemical components of pollen grains and stigma which play a role in their interaction. These include glycoproteins in pollen grains and protease activity on stigma surface, leading to the activation of pollen cutinase necessary for degradation of cuticle. Calcium is another important constituent of in vitro germinating pollen and serves as a chemoattractant for guiding the growth of pollen tube. Once pollen grains are deposited on to the stigmatic surface, the process of adhesion is initiated. Pollen adhesion involves an interaction of proteins present in the pellicle and the pollen wall. After adhesion, various proteins from the pollen coat, including glycine-rich proteins, calcium-binding proteins, lipase and cutinase, facilitate the process of hydration by causing the breakdown of lipidic constituents at the pollen-stigma interface. It is possible that ROS plays a role in defense mechanisms by being toxic to pathogens. NO is also important in imparting immunity to the receptive stigma, and it also increases thermotolerance by activating ROS scavenging enzymes, thereby playing a role in ROS-mediated signaling processes. After pollination, interaction between pollen-localized NO and stigmatic ROS is likely to play a crucial role in pollen recognition, and signaling between stigmatic papillae and pollen grains. Thus, both the reproductive structures (pollen and stigma) undergo a complex series of biomolecular crosstalk between pollen and stigma to facilitate reproductive success in the form of seed set. Major molecular markers associated with stigma maturation have been summarized in .
Table 1. Molecular markers of stigma maturation in sunflowerCitation12–14.
Major biochemical events during seed development
Seed development in sunflower can be considered in three phases, namely cell division, cell proliferation and cell maturation.Citation15 During the initial phase up to 10 days after anthesis (DAA), fertilization and cell divisions take place and embryonic structures and pericarp are defined. During intermediate phase, there is an increase in cell size and degree of vacuolation. During seed maturation, seeds dehydrate and accumulate OBs. A rapid accumulation of proteins and lipids is evident up to 30 DAA, after which protein accumulation declines while lipids continue to accumulate. OB membrane in sunflower and few other oil seeds has oleosin proteins as an intrinsic constituent. Oleosins impart stability to OBs through steric hindrance and electronegative repulsion. The pattern of expression of oleosin isoforms is different in early forming oil bodies than those appearing in later stages of seed development.Citation16 This highlights the differential role of oleosin isoforms expressed at different stages of seed development. Differential expression of oleosin isoforms is thus considered as a determinant of oil body longevity in germinating seeds. Further analysis of the pattern of intrinsic protein constituents of oil body membranes has been undertaken in the author’s laboratory by rigorously removing extrinsic proteins from OB membranes by urea washing. Proteomic analysis of sunflower seeds has highlighted differences in the composition of seed proteins during the three phases of seed development.Citation17 There is a difference in protein expression at various stages of seed development, especially those associated with primary metabolism, with increased levels of glyceraldehydr-3-phosphate dehydrogenase (GAPDH), phosphoglycerate kinase and nucleoside diphosphate kinase. Furthermore, stage-specific expression of ROS metabolizing enzymes is evident and two SOD isoforms are expressed during 30 DAA stage of seed development. This could be the first line of defense against oxidative stress. Maximum ROS accumulation is evident in seeds at 20 DAA stage, coinciding with high metabolic activity, and it decreases gradually with seed maturation. Interestingly, at 20 DAA stage, developing seeds express glycine-rich RNA binding proteins, which are involved in the post-translational modification of proteins, thereby regulating various signaling processes. Significant variations in the expression of proteins associated with OB biogenesis and modification of fatty acid reserves are also observed during seed development. At 30 DAA stage, activation of cytosolic proteolytic pathways and novel expression of associated proteins participating in the proteolysis of unwanted proteins and protein turnover in the cell, are evident. Our observations have also indicated a probable crosstalk between ROS and protein kinase C (PKC) during seed maturation phase. ROS scavenging enzymes (principally SOD and POD) contribute to a reduction of ROS produced in developing seeds through a temporal distribution of their maximal activities during various stages of seed development. ROS and PKC interaction is likely to occur through a coordinated action of ROS and antioxidant enzymes on the regulatory and catalytic domains of PKC, with calcium ions regulating both PKC activity and ROS homeostasis through various scavenging mechanisms.Citation18
Signaling and enzymatic actions leading to oil body (OB) mobilization during seed germination
Oil bodies and TAGs stored within them exhibit far greater susceptibility to mobilization/degradation in light than in dark-grown seedlings.Citation4 OB mobilization is dependent on the collective action of several lipolytic and proteolytic enzymes. Germination of sunflower seeds and seedling growth are accompanied with mobilization of OB membrane proteins, majorly oleosin isoforms (16, 17.5, and 20 kDa). Interestingly, these three oleosin isoforms exhibit temporal differences in their mobilization patterns accompanying seedling growth. In vivo and in vitro analyses demonstrated that 17.5 kDa oleosin isoform exhibits maximum susceptibility to proteolytic degradation. Degradation of oleosins is chaperoned with the accumulation of a 11 kDa polypeptide fraction known as the ‘protease protected fraction’ (PPF).Citation5 PPF starts accumulating right after seed germination and continues to accumulate in seedling cotyledons with advancing age of seedlings. The extent of PPF accumulation is relatively higher during seedling growth in dark than in light. Nile red stained OBs from seedling cotyledons at different stages of growth display variable fluorescence, ranging from yellow to green and red, indicating that there is a constant change in lipid composition in the OB matrix with advancing seedling growth. A 65 kDa, thiol protease is one of the primary enzymes responsible for carrying out degradation of oleosins associated with OB membrane.Citation5,Citation7 Action of thiol protease is also responsible for rendering OBs susceptible to the action of other seed germination-associated enzymes, like phospholipase A2 (PLA2), lipase and lipoxygenase (LOX).Citation7,Citation8,Citation19 Cysteine protease-mediated mobilization of OB proteins is the first step of OB mobilization in sunflower seedling cotyledons.Citation7 Further evidence from zymographic analyses and in-situ localization confirm the role of cytosolic thiol protease in OB mobilization.Citation7 Fluorescein mercuric acetate (FMA) – binding at thiol protease-binding sites in protoplasts from sunflower seedling cotyledons is indicative of its expression on OB surface.Citation7 Thiol protease cleaves oleosin isoforms into small polypeptide fragments, making the OB matrix susceptible to TAG-mobilization enzymes. Protein storage vacuoles (PSVs), localized using BCEF-AM, display seedling development associated variations in size. With increasing age, small PSVs coalesce into larger ones.
Lipases are initially localized in PSVs prior to seed germination. Upon initiation of germination, they get transferred to OB surface for catalyzing hydrolysis of storage lipids.Citation6,Citation19 Using fluorescent glycerol-based resorufin-ester as substrate in OB-containing protoplasts isolated from sunflower seedling cotyledons, it has been demonstrated that lipase migration from PSVs to OB surface increases with advancing age and development of seedlings. Cytosolic PLA2 acts on catalysis of OB membrane phospholipids, thereby depleting phosphatidylcholine (PC) pool. A concomitant rise lyso-phosphatidylcholine (LPC) (the degradation product from phospholipids) level is evident with increasing age of sunflower seedling cotyledons. Its rise in the cytosol coincides with elevated levels of PLA2 as the seedlings grow. PLA2 action modulates OB membrane and makes it more prone to lipase action. A novel probe, PED6, was employed to visualize the expression of PLA2 on OB surface and cytosol.Citation8 CLSM imaging does indeed indicate a possible migration of these enzymes (PLA2 and lipase) from cytosol to OB surface so as to facilitate lipolysis and TAG hydrolysis. Although early action of PLA2 and its subsequent migration to OB surface seem evident but the mechanism behind its transfer remains to be explored. Sunflower seedlings raised in light exhibit higher activity of fatty acyl-ester hydrolase.Citation4 Its major isoforms (40–50 kDa) show high expression in light-grown seedlings. Expression of fatty acyl-ester hydrolase (responsible for MAG and DAG hydrolysis) is also photomodulated significantly and its activity increases several folds in light-grown seedling cotyledons compared to that in dark-grown ones. It is thus evident that seed germination and seedling growth in sunflower are regulated by a light-promoted sequential migration of a series of enzymes from cytoplasm to OB surface to facilitate systematic proteolytic degradation of oleosins, followed by TAG hydrolysis in the OB matrix to generate energy for the growing seedling.
Molecular switches regulating adventitious and lateral rooting
Adventitious roots (AR) are post-embryonic roots and their formation is stimulated by redifferentiation of predetermined mother cells destined for initiation of root primordia. The process of AR formation is accomplished in three physiologically interdependent phases: induction, initiation and extension. Various environmental and endogenous factors, such as temperature, light, hormones (particularly auxin), sugars and mineral salts, promote advancement of redifferentiation of the predetermined mother cells, resulting in AR induction. Nitric oxide (NO) plays a crucial role in root development. Work in the author’s laboratory has provided evidence for a significant interaction between auxin, NO, and actin during AR formation from sunflower seedling hypocotyls.Citation20 NO-dependent and NO-independent roles of auxin in AR development have been demonstrated using various pharmacological agents, such as 1-napthylphthalamic acid (NPA; a phytotropin which inhibits polar auxin transport), latrunculin B (Lat B; disrupts the organization of actin filaments), cyclosporin A (CsA; an inhibitor of cyclophilins), sodium nitroprusside (SNP; NO donor) and 2-phenyl-4,4,5,5-tetramethyllimidazoline-1-oxyl-3-oxide (PTIO; NO scavenger). Initial phase of AR development i.e. induction, is solely regulated by IAA, independent of NO.Citation20 Significant interaction between auxin and NO is associated with later phases of AR development, i.e., initiation and extension growth. Interaction between actin filaments and NO is also observed during auxin-induced AR formation during AR initiation and extension. Lat B inhibits IAA-induced AR response and NO accumulation in the interfascicular cells of the hypocotyl. CLSM imaging also revealed bundling of actin filaments with NPA and Lat B treatments. Investigations done using cyclosporin have demonstrated a probable involvement of cyclophilins in PIN protein (auxin efflux protein) localization, thus suggesting a role of cyclophilins as a potential signaling intermediate during AR formation.
Our attempts to look for novel signaling molecules in facilitating root development brought out interesting findings on strigolactones (SLs). Plants produce a variety of SLs and most of them are characterized from plant root exudates. SLs are plastidial in origin and are derived from carotenoid biosynthetic pathway, involving two carotenoid cleavage dioxygenases (CCDs). SL biosynthesis is known to be modulated by light. Attempts have been made in the author’s laboratory to detect and quantify endogenous SLs in roots, cotyledons and first pair of leaves derived from sunflower seedlings using analytical HPLC, FT-IR spectroscopy and ESI-MS.Citation21 Results also revealed the role of light in the regulation of SL accumulation in sunflower seedlings. Roots of light-grown seedlings exhibit higher CCD activity as compared to dark-grown ones during early stages of development, thereby highlighting photomodulation of CCD activity. These observations suggest migration and involvement of a yet to be discovered signaling molecule or SL precursors from light-exposed aerial parts of seedling to the roots that remain in dark. This work provides strong evidence for the presence of SLs in sunflower seedling roots and leaves and a positive correlation between photomodulated CCD activity and SL accumulation in sunflower seedlings. Lateral roots (LRs) emerge from pericycle cells in primary root through a series of cell divisions. Auxins act as central players both in LR and AR development. Auxin-evoked AR development is dose-dependent i.e. high concentration of auxin is required initially at the site of AR induction, followed by a decrease and again high during extension phase.Citation20,Citation22 SLs govern root development by altering polar transcellular transport of auxin. Thus, SLs modulate localized intracellular accumulation of auxin. Auxin and ethylene control different aspects of root development and exhibit differential sensitivity to SLs. An inverse correlation between SL-induced LR development and ACC synthase activity (key enzyme for ethylene biosynthesis) has also been established.Citation23 Endogenous NO inhibits CCD activity, thereby modulating the role of SLs during LR development. CCD is an iron-containing enzyme and NO is likely to have affinity to reversibly bind with Fe2+ present in its active site, as in the case of guanylate cyclase, cytochrome oxidase or catalase. On the basis of this affinity, it is expected that NO binds with Fe2+ of CCD molecule and inhibits its activity. These investigations highlight SL-modulated sensitization of auxin-ethylene co-action during LR development which is, in turn, controlled via regulation of CCD activity through reversible binding/release of NO with its Fe 2+ component (). Further work in this direction is likely to provide more insights into the molecular mechanisms of LR development through NO-SL crosstalk.
Figure 1. NO-modulated ethylene and SL biosynthesis and possible causes of their impact on lateral root formation
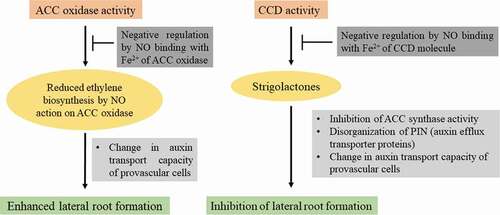
NO-ROS crosstalk associated with long distance sensing of salt stress in seedlings
Effect of NaCl stress on sunflower seedling growth is majorly concentration-dependent, with the effects of salinity visible upon treatment with 120 mM NaCl. NaCl stress manifests itself in sunflower seedlings as reduced hypocotyl length, unexpanded cotyledons and diminished root proliferation.Citation24 NaCl stress leads to accumulation of noxious Na+ ions in the roots as well as aerial tissue, thereby reducing its water potential. A significant increase in Na+/K+ ratio is noted in sunflower seedling roots. Rapid, long distance transport of sodium ions to cotyledons disrupts the ionic make-up of aerial tissue. Paucity of information on the mechanisms of uptake, accumulation and transport of Na+ and Cl− in various organs of sunflower prompted investigations in the author’s laboratory to decipher the relation between growth, water status and ion uptake.Citation25 The uptake, transport and accumulation of Cl− is more than that of Na+. Growth reduction during low salinity stress is mostly attributed to Cl− toxicity.Citation26 Plants subjected to salinity stress show reduction in growth, biomass and water content. NaCl exerts a concentration-dependent effect on the reduction in plant biomass. The findings revealed that NaCl concentration <40 mM does not exert noteworthy effects on growth reduction while 80 or 160 mM NaCl leads to its compartmentalization in roots. It is, therefore, proposed that sunflower (variety – Morden) is moderately tolerant to NaCl stress.
Excess Na+ alters the water relation of the plant, thus affecting its growth. In order to understand the regulation of ion transport across sunflower seedling root cells, patch clamp electrophysiology was performed on protoplasts derived from the roots of salt-tolerant sunflower (hybrid KBSH-53).Citation27 Ion conductance across the plasma membrane of sunflower root cells has been observed to be regulated by K+ outwardly rectifying channels (KORs) and nonselective cation channels (NSCCs). Sunflower seedling growth (hypocotyl elongation and primary root extension) improves in the presence of external Ca2+ (2 mM CaCl2), which correlates with reduced Na+ influx mediated by Ca2+-sensitive Na+-permeable NSCCs.Citation27 Furthermore, extracellular Ca2+ enhances Na+ sensitivity of the KORs, thus reducing K+ loss. This work is the first study using patch clamp and voltage-clamp electrophysiology in sunflower seedling roots and provides substantial evidence on the tolerance mechanism of sunflower to NaCl stress, wherein, ion conductance across the plasma membrane of root cells is found to be sensitive to extracellular Ca2+ and Na+. Long distance transport of ions appears to play a critical role in imparting salt tolerance to sunflower plants. Salt-sensitive sunflower plants exhibit poor root proliferation, reduced LR formation and early floral induction, accompanying steep rise in soil salinity and electrical conductivity.Citation28 This reflects the ability of salt-sensitive plants to restructure root architecture and flowering under salt stress. Long distance salt stress signaling is marked with massive accumulation of Na+, loss in storage lipids, downstream regulation of oleic and linoleic acid levels in sunflower achenes. Storage lipids (TAGs) of salt-tolerant plants exhibit up to 40% decline in U/S ratio (ratio of unsaturated to saturated fatty acids) under salt stress. Eicosanoic acid (22:0) seems to play a signaling role in salt-sensitive sunflower plants facing salt stress. Its elevated levels in salt-sensitive plants may affect membrane fluidity. Cis-vaccenic acid (18:1 ΔCitation9,Citation12,Citation16) is also likely to serve as a lipid marker/signal for salt sensitivity (). Future work in this direction is likely to provide new information on long distance ionic homeostasis and lipid signaling under salt stress.Citation29 Salt tolerant sunflower seedlings exposed to 120 mM NaCl exhibit high proteolytic degradation of OB membrane proteins, particularly oleosins, whereas salt-sensitive seedling cotyledons show negligible proteolytic activity, thereby retaining OB membrane integrity for a longer time.Citation28
Figure 2. Proposed model of fatty acid biosynthesis in sunflower achenes and modulation of its composition as a response to 120 mM NaCl
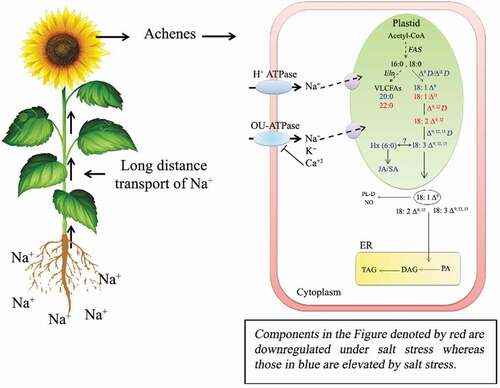
Endogenous nitric oxide (NO) has a possible role in NaCl-induced physiological and biochemical regulation of sunflower seedling growth. Mitochondria and peroxisomes are primarily involved in contributing to the cytosolic NO pool of the cell. NO serves as an early signaling molecules in response to application of NaCl.Citation24 Transient increase in the activity of putative NO-synthase (NOS) evident in sunflower seedling roots subjected to salt stress correlates with enhanced NO biosynthesis. It also correlates with the regulation of Na+/K+ ratio, indicating that NO modulates the endogenous levels of noxious sodium ions.Citation24 LR induction and extension are probably governed by a probable crosstalk between NO and ethylene biosynthesizing enzyme - ACC oxidase. NaCl stress and NO depletion have addictive effect on the enhancement of ACC oxidase activity. It is proposed that salt stress mediated LR proliferation is regulated by NO through its binding with ACC oxidase, an iron-containing enzyme.Citation30 Similarly, enhanced levels of NO on the surface of OB membrane from salt stressed sunflower seedlings cotyledons indicates NaCl-triggered accumulation of NO on OB surface. This accompanies slower oleosin degradation during NaCl stress. Putative NOS activity in roots is possibly regulated by the activity of Na+/K+ transporters during NaCl stress. Thus, a protective role of NO during lipolysis and oleosin degradation in salt-stressed sunflower seedling cotyledons is evident. Pharmacological investigations with various NO donors like – sodium nitroprusside (SNP), diethylenetriamine NONOate (DETA), S-nitroso-n-acetyl-D, L- penicillamine (SNAP), and 4-(p-methoxyphenyl)-1,3,2-Oxathiazolylium-5-olate (CAY) have demonstrated their differential and concentration-dependent NO releasing ability in the growth medium.Citation31 Hemoglobin functions as a more effective NO quencher in comparison with routinely used cPTIO.Citation32 Among the various NO donors used, DETA (500 μM) is most effective with 95% increase in endogenous NO in dark-grown sunflower seedling roots. SNP dissociates in the medium thus releasing free iron and cyanide ions, which limits its use as a NO donor.Citation31 CLSM imaging has shown differences in the extent of tyrosine nitration of proteins in roots and cotyledons of seedlings treated with DETA or SNP as NO donors. With higher NO releasing ability of DETA, the extent of tyrosine nitration is also found to be higher.Citation31
Various abiotic stress factors, primarily salinity stress, lead to excessive generation of ROS in plant cells by the impairment of cellular electron transport within different subcellular compartments, such as chloroplasts and mitochondria. This causes lipid peroxidation as well as induction of metabolic pathways, such as photorespiration. Plant cells possess both enzymatic and non-enzymatic machinery to ameliorate the lethal impact of enhanced ROS production during stress. Differential spatial distribution of different isoforms of superoxide dismutase (SOD) constitutes the first line of defense against ROS. Work in the author’s laboratory has provided new insights on the synchronous modulation of the activity of SOD isoforms by NaCl and its crosstalk with NO.Citation33 In seedling cotyledons, three spatially located SOD isoforms respond differently to NaCl stress with the advent of seedling growth and development. CLSM imaging has shown that NaCl stress elevates the distribution of MnSOD in the cotyledon cells and decreases the distribution of Cu/ZnSOD. Exogenous application of NO donor differentially induces SOD isoforms in sunflower seedlings in response to NaCl stress. NO enhances FeSOD and Cu/ZnSOD activities in salt-stressed seedling roots. Interestingly, NaCl inversely modulates the impact of NO on FeSOD activity in seedling cotyledons. NO enhances FeSOD activity in cotyledons of control seedlings. These results suggest different intracellular signaling pathways operative in seedling roots as an early salt stress mechanism and in cotyledons as an early long-distance NaCl stress sensing mechanism. NO leads to variable expression of SOD isoforms due to its concentration dependent biphasic (pro- and antioxidant) nature of action.Citation34 It has further been observed that sunflower seedlings subjected to salt stress (120 mM NaCl) exhibit high POD activity, differential expression of its isoforms and accumulation of lipid hydroperoxides.Citation35,Citation36 This coincides with high hydroperoxide glutathione peroxidase (PHGPX) activity in seedling cotyledons. Thus, sensing of salt stress in relation with lipid hydroperoxide accumulation and its scavenging through an upregulation of PHGPX activity in the seedling cotyledons is evident.
Work from the author’s laboratory examined the temporal and spatial distribution of heme oxygenase (HO-1), its activity and expression in sunflower seedling cotyledons as a long distance signaling response to salt stress (120 mM NaCl).Citation37 Results also demonstrated the role of specialized cells around the secretory canals in the seedling cotyledons. Significantly enhanced distribution of HO-1 has been observed in the specialized cells enclosing the secretory canals in response to NaCl stress. Modulation of spatial distribution of HO-1 in response to NaCl stress indicates long-distance salt stress sensing through its differential distribution in sunflower seedling cotyledons utilizing secretory canals for the transport of signaling molecules. In plant cells, secretions of diverse substances are likely to play critical roles in long distance sensing of stress. NO positively modulates HO-1 activity in seedling cotyledons and NaCl stress tends to antagonize NO action. NO action on HO-1 is probably through its interaction with heme group. Thus, a probable link between endogenous NO, NaCl stress and iron homeostasis by way of HO-1 activity modulation is proposed. This research area needs further exploration at ultrastructural level, in order to decipher long distance salt sensing mechanisms operative in plants.
Polyamine (PA) metabolic pathways are highly regulated in plants as dynamic balance between their synthesis and catabolism is crucial for these molecules to combat stress-induced adversities. In plants, free PA titers are regulated through the reversible conjugate formation and/ or through modulation of their synthesis, transport, and degradation. NO regulates PA-signaling pathways operative in plants. Putrescine serves as the precursor molecule for the synthesis of higher PAs. Recent work analyzed the role of NO in modulating the activity/expression of the PA biosynthetic enzymes (ornithine decarboxylase, ODC; EC 4.1.1.17 ODC: arginine decarboxylase, ADC; EC 4.1.1.19 and S-adenosylmethionine decarboxylase, SAMDC; EC 4.1.1.50) and catabolic enzymes (diamine oxidases, DAO and polyamine oxidases, PAO) in sunflower seedling cotyledons raised in the presence of 120 mM NaCl stress.Citation38 NO positively regulates cellular equilibrium of PA and minimizes the impact of salt-stress in seedling cotyledons. Salt stress enhances PA catabolic enzyme (PAO) activity. However, NO tends to scale down the impact of salt-induced degradation of PAs and regulates PA homeostasis by increasing the activity and abundance of PA biosynthetic enzymes (especially ADC and SAMDC). NO also regulates the spatial distribution of spermine in the affected cells.
Under stress, plants accumulate compatible solutes to maintain cellular osmotic balance, plasma membrane integrity and fluidity, ROS detoxification and to protect macromolecules. Under salt stress, glycine betaine (GB) regulates the cellular mechanisms by reducing the loss of K+ ions and controlling high K+/Na+ ratio by suppressing the apoplastic routes of Na+ uptake thereby maintaining the equilibrium of K+ ions. Recent findings from the author’s laboratory have put forward new insights on the co-regulation of GB homeostasis by NO and light in sunflower seedling cotyledons.Citation39 These investigations also highlight the prime role of NO in GB accumulation as a long-distance response to salt (120 mM NaCl) stress. Three possible ways of NO action in maintaining GB homeostasis have been proposed: 1. Preferential mobilization of S-adenosylmethionine (SAM) in the biosynthesis of choline by inhibiting the activity of aminocyclopropanecarboxylate oxidase (ACC oxidase) in the cytosol as NO has affinity to bind with Fe 2+ present in the active site of the enzyme; 2. NO further modulates the abundance and activity of BADH; and 3. GB-NO complex may act as a key complex in regulating plant growth under salt stress. These findings hold promise for further interesting work.Citation39
Recent work has provided crucial evidence for the osmoregulatory role of polyamines and glycine betaine as a function of modulation of two major aquaporins (AQP) subfamilies, namely plasma membrane intrinsic protein 2 (PIP2) and tonoplast intrinsic protein 1 (TIP1).Citation40,Citation41 Salt Stress (120 mM NaCl) induced reduction in relative water content (RWC) accompanies enhanced abundance of PIP2 and TIP1 in cotyledons.Citation40 Salt stress and NO donor (DETA) application lead to preferential accumulation of monomeric form of PIP2 whereas expression of TIP1 decreases in seedlings roots. The impact of salt stress is independent of the impact of NO.Citation41 Thus, NO signaling seems to have a role in the expression and accumulation of PIP2 and TIP1 water channels in seedling roots under salt stress. NO-induced PTMs might possibly functionally modify PIP2 and TIP1 isoforms in seedling roots. AQPs may also serve as turgor sensors to regulate conductance of K+ channels in the root cell membranes.
It is thus evident from these findings that salt stress sensitivity and tolerance in sunflower seedlings is a manifestation of various independent and crosstalking biochemical routes whose modulation by NO as a signaling molecule paves way for adaptive molecular mechanisms over transcellular long distances in the seedlings facing stress.
NO-melatonin interaction and associated ROS-RNS signaling in salt-stressed seedlings
Serotonin and melatonin have been known to play a pivotal role in abiotic stress tolerance.Citation42 In line with earlier investigations on serotonin and melatonin in plants from other laboratories, work in the author’s laboratory has revealed differential spatio-temporal distribution of serotonin and melatonin accumulation in NaCl-stressed sunflower seedling roots and cotyledons. In this context, author’s laboratory has provided the first report on the effect of salt-stress on serotonin and melatonin content and their accumulation in seedling roots and cotyledons.Citation43 NaCl stress upregulates serotonin and melatonin biosynthesis in sunflower seedlings, which further increases with the advancement of seedling age. Exogenous serotonin and melatonin treatments lead to amelioration of NaCl stress-induced inhibition of seedling growth, which coincides with high endogenous accumulation of these biomolecules. NaCl-stress induced high melatonin accumulation in sunflower seedling cotyledons implies the role of these indoleamines in long distance signaling during NaCl stress. Immunohistochemical localization has demonstrated that serotonin distribution is associated with endodermal cells of roots while melatonin is distributed more in the cortical region. High accumulation of serotonin and melatonin is also observed in the OB-containing cells of cotyledons. The observations on spatio-temporal distribution of serotonin and melatonin are in correlation with variations in their endogenous content. The evidence thus obtained shows possible long distance signaling of serotonin and melatonin in NaCl-stressed sunflower seedlings. Future investigations are necessary to know the role of serotonin and/or melatonin in the regulation of OB mobilization and associated lipolytic events in sunflower seedling cotyledons. It is possible that melatonin might be involved in slower degradation of OB in cotyledons during salt stress, which is likely to enhance the longevity of the seedlings subjected to salt stress. Further work is, thus, expected to reveal the role of melatonin in OB biogenesis and lipolysis in sunflower. Since auxin and serotonin biosynthesis are initiated from tryptophan (common precursor), it is likely that a surge in serotonin biosynthesis might interfere with auxin biosynthesis and its distribution in some tissues of salt-stressed sunflower seedlings. NaCl stress-induced growth changes in sunflower seedlings with high endogenous serotonin accumulation might correlate with altered auxin biosynthesis and distribution.
NO and melatonin crosstalk has been demonstrated in response to NaCl stress induced modulation of glutathione homeostasis in sunflower seedling cotyledons.Citation44 Application of SNP upregulates melatonin biosynthetic enzyme – hydroxyindole-O-methyltransferase (HIOMT) in salt stressed seedling cotyledons. NO and melatonin differentially regulate glutathione content (GSH/GSSG ratio) and glutathione reductase activity in sunflower seedling cotyledons.Citation44 Similar instance of the integrative role of NO and melatonin has been reported later wherein interaction between the two biomolecules results in differential modulation of the two SOD isoforms, i.e., Cu/Zn SOD and Mn SOD in NaCl stressed sunflower seedlings.Citation33 Furthermore, both the biomolecules participate in mediating an early long distance signaling from seedling roots to cotyledons. Melatonin potentially regulates the surge in ROS and RNS generated in NaCl-stressed sunflower seedlings. NO acts as a positive regulator of endogenous melatonin accumulation in seedling cotyledons, which is also accompanied with differential expression of SOD isoforms. Based on the cumulative evidence, authors also proposed similar mechanisms of crosstalk and signaling pathways to be associated with NO, melatonin and hemoglobin interaction in plants and human beings alike during stress conditions.Citation45
Does NO as a long distance signaling molecule require a carrier molecule for its targeted delivery in plant cells? Endogenously produced S-nitrosothiols (SNOs) are known to be involved in transport, storage and delivery of NO. SNOs also play a significant role in redox-based, PTMs, such as S-nitosylation of cysteine residues of proteins. S-nitrosoglutathione (GSNO) is the most abundant SNO in biological systems, and is thought to act as an intracellular reservoir of biologically active NO both in animal and plant cells. Recent investigations from the author’s laboratory proposed the probable role of N-nitrosomelatonin (NOMela) in the intracellular transport of NO in plant cells.Citation46 At physiological pH, melatonin can be easily nitrosated at the nitrogen atom of the indole ring to produce NOMela. NOMela can act as a potential NO releasing compound and can compete with SNOs to function as intracellular NO carrier. It can efficiently transfer its nitroso function to activated hydroxyl compounds, such as vitamin C and E, catechols and serotonin. As NOMela releases melatonin with the generation of NO, it acts both as an efficient NO reservoir and transporter, and as an efficient antioxidant. However, SNOs, such as GSNO, under aerobic conditions produce other noxious oxidizing nitrogen species upon NO release. Furthermore, NO release from NOMela is independent of the composition of the buffer system. In contrast, in GSNO it is dependent on buffer composition. In fact, most recently, NOMela has actually shown to be a much better NO transporter than GSNO from roots to cotyledons in dark-grown Arabidopsis seedlings.Citation47
S-nitrosylation and tyrosine nitration of proteins as salt sensing regulatory mechanisms
Sensing of salt stress accompanies modulation of NO accumulation and protein tyrosine nitration as marker of nitorsative stress.Citation48 Sodium nitroprusside (SNP) is one of the most extensively used NO donor in plant science research. However, its use in NO research is debatable since SNP releases deleterious ions, such as cyanide and iron, in solution along with NO. Enhancement of ROS levels in tissues subjected to SNP treatment further highlights its limitations as NO donor. NONOates, on the other hand, are among the purest NO donors. Exogenous application of NO donors (SNP and DETA) ameliorates the detrimental effects of salt stress in sunflower seedlings by promoting hypocotyl extension and root growth. Exhausted SNP (exSNP) prepared by irradiation and heat treatments, releases NO, free iron and cyanide at even higher concentrations than SNP, thereby questioning its use as a reliable control in NO research. A detailed analysis of SNP-modulated growth responses vis-à-vis other established NO donor (DETA) has been carried in sunflower seedling cotyledons.Citation49 A thorough proteomic and biochemical approach has helped in deciphering the NO, CN-, and Fe-mediated actions of SNP. Proteomic analysis of cotyledons obtained from sunflower seedlings grown in presence of 120 mM NaCl, SNP and exSNP has highlighted differential abundance of 138 proteins belonging to 10 different functional categories, namely transport, storage, primary metabolism, other metabolic proteins, proteolysis, stress-response, translation, chaperone, regulatory and miscellaneous category.Citation49 Salt stress alone modulates the abundance of 127 proteins. However, SNP and exSNP modulate the abundance of 117 (in the absence of NaCl) and 129 (in the presence of NaCl) proteins. Both SNP and exSNP enhance the abundance of many proteins which are positively modulated by salt stress as well. Subsequent biochemical analysis has demonstrated that NO (in the form of SNP and DETA) decreases the activities of salt-responsive proteins, glyceraldehyde-3-phosphate dehydrogenase (GAPDH) and S-adenosylmethionine synthase (SAMS). Based on these findings from the author’s laboratory, SNP-modulated cytosolic proteins have been segregated depending on their probable effects due to NO, Fe and cyanide thus, highlighting NO, cyanide and Fe mediated action of SNP.Citation49 These findings will facilitate careful evaluation of data from SNP as a pharmacological agent in plant science research.
A probable crosstalk between NO, auxin and actin during adventitious root formation has been demonstrated in sunflower hypocotyls.Citation50 Hypocotyl protoplasts treated with auxin exhibit rapid accumulation of NO. Whole hypocotyl explants showing adventitious root development also show a surge in NO production. AR development has also been shown to be regulated by NO-mediated PTMs. Immunoblot and immunofluorescent localization analysis has provided evidence for tyrosine nitration of proteins in sections obtained from basal regions of AR-producing hypocotyl segments subjected to various treatments.Citation22 AR-inducing treatments (IAA and SNP) result in the accumulation of certain tyrosine nitrated proteins having molecular mass close to 25 kDa. However, tyrosine nitration is completely absent in AR suppressing treatment (NPA).
Under various biotic and abiotic stress conditions, there is an overproduction of NO and RNS [such as peroxynitrite (ONOO−), S-nitrosoglutathione (GSNO), etc.] which serve as biomarkers of nitrosative stress in plants. NO overcomes the negative impact of salt stress in sunflower seedlings by promoting hypocotyl elongation. A preferential and enhanced accumulation of NO around OBs has been demonstrated in sunflower seedling cotyledons within 48 hrs of exposure to 120 mM NaCl, thus suggesting that a long-distance signal is rapidly being transported in salt-stressed sunflower seedlings from roots to cotyledons.Citation24 NO-mediated PTMs of ROS-scavenging enzymes play vital roles in regulating oxidative stress and maintaining ROS homeostasis in plants. NO modulates the activities of various ROS-scavenging enzymes, such as peroxidase, catalase, superoxide dismutase and glutathione reductase in sunflower seedling roots and cotyledons in response to salinity stress.Citation34,Citation44,Citation51,Citation52 NO regulates the activity of target proteins via two major PTMs, S-nitrosylation and tyrosine nitration.Citation53 Enhanced tyrosine nitration of POD as a mechanism to increase enzyme activity accompanying salt stress has been demonstrated in sunflower seedlings, thereby suggesting a probable NO-POD interaction as a rapid long distance signaling response.Citation52 Immunoblot analysis and CLSM imaging has revealed enhanced tyrosine nitration of proteins in salt stressed sunflower seedling roots and cotyledons. These observations suggest that salt stress sensing in sunflower is accompanied by transport of nitrosative stress signal from roots to cotyledons within 48 h of exposure to salt stress. Out of the four tyrosine residue found in the amino acid sequence of POD, the one at position 100 is predicted to undergo nitration.Citation52 Enhanced tyrosine nitration of oil body membrane proteins provides longevity to oil bodies thereby helping in survival under salt stress conditions.Citation24
Nitrite and nitrosothiols (RSNO) are considered as biomarkers of NO metabolism and nitrosative stress. Sunflower seedling roots contain high levels of both nitrite and nitrosothiols as compared to seedling cotyledons.Citation54 Salt stress and NO donor treatment further enhance the levels of both these biomolecules, thereby indicating their importance in plant defense responses. S-nitrosoglutathione reductase (GSNOR) is an important enzyme which regulates intracellular levels of S-nitrosoglutathione (GSNO). A significant reduction in the activity of S-nitrosoglutathione reductase (GSNOR) in response to salt stress suggests its role in mediating nitrosative stress in plants. Biotin switch assay and LC-MS/MS analysis to assess qualitative and quantitative variations in the pattern and extent of S-nitrosylation of cytosolic proteins extracted from sunflower seedling roots and cotyledons has shown that both the tissues exhibit opposite patterns of S-nitrosylation in response to salt stress. Denitrosylation of proteins is evident in seedling roots while cotyledons exhibit enhanced S-nitrosylation in response to 120 mM NaCl. Sixty-one proteins are regulated by S-nitrosylation in sunflower seedlings, of which seventeen are unique to cotyledons, four are found only in roots and forty are common to both the tissues. Eighteen novel S-nitrosylated proteins, including malate synthase, calmodulin, aquaporin, calreticulin, oleosin, pectinesterase and phospholipase D, have been reported in sunflower seedlings in response to salt stress. Proteins belonging to various categories, primary metabolism, regulatory, cytoskeletal, transport, defense-related, chaperones and other metabolic proteins, exhibit significant modulations in the extent of their S-nitrosylation. These observations implicate protein S-nitrosylation and denitrosylation as regulatory mechanisms in the sensing of salt stress in sunflower seedlings.Citation54
Novel protocols for localization of signaling and related biomolecules
The progression of lipolysis leading to degradation of TAGs in the OBs is accompanied by the coordinated action of various enzymatic and non-enzymatic signaling molecules which are transiently expressed on the surface of OB membranes. Novel fluorescence imaging approaches have been developed in the author’s laboratory to localize biomolecules associated with lipolytic events in sunflower seedlings. Additionally, analysis of OB membrane proteins by purification using detergent has proved very useful.
Analysis of membrane proteins: Membrane proteins are associated with important signaling molecules which function in specific lipidic environment within the cells, and are involved in various cellular functions. Although detergents are capable of fragmenting membranes, efforts for complete removal of lipids from the surroundings of the proteins often render them non-functional/ inactive. Furthermore, detergents meant for membrane rupture and protein solubilization can alter the physiochemical properties of the lipid environment in which these proteins essentially functions. Methods have been developed in the author’s laboratory which are suitable for isolation and electrophoretic separation of OB membrane proteins from sunflower seedling cotyledons.Citation5 OBs obtained after centrifugation of cotyledon homogenates (10,000 g supernatant) are subjected to successive washing with 9 M urea and 0.1 M NaHCO3 as denaturants. In this context, it may be noted that bicarbonate washing of OBs is performed for extracting proteins which are not firmly attached to the OB membrane (extrinsic proteins), while urea washing releases all other intrinsic proteins except oleosins, which are resistant to denaturation by urea. The OB membrane proteins are solubilized in diethyl ether after removal of neutral lipids present in the washed OBs. Electrophoretic separation of OB membrane proteins in linear gradient-urea containing gel revealed new polypeptides of 47, 57, 85 and 195 kDa (carbonate- washed fractions) to be associated with various stages of seedling development. Furthermore, separation of urea-washed OBs revealed the presence of three distinct isoforms of oleosins with molecular masses of 16, 17.5, and 20 kDa, respectively. Although, 16 kDa isoform of oleosin is present in all stages of seedling cotyledons after seed germination, 17.5 kDa and 20 kDa isoforms gradually disappear with advancing stages of seedling growth. A comparison of oleosin profile from urea-washed OBs from peanut, rape seed and soybean has shown their differences in oleosin degradation patterns as compared to sunflower. OB membrane proteins obtained after urea washing are also prone to degradation by TSP (total soluble protein) from seedling cotyledons as a source of protease. Furthermore, oleosins subjected to trypsin digestion-produced polypeptide fragments of 8 to 8.8 kDa as final degradation products.
Work has also been undertaken in the author’s laboratory to characterize OB membrane proteins associated with the onset of desiccation (20–30 d after anthesis; DAA) during seed development in sunflower.Citation17 Urea-washed OB membrane proteins subjected to 2D-PAGE followed by protein characterization by LC-MS/MS have shown the proteins unique to 20–30 DAA which belong to seven functional classes of proteins (energy metabolism, reactive oxygen scavenging, proteolysis and protein turnover, signaling, oleosin and oil body biogenesis-associated proteins, desiccation, and cytoskeleton) whereas 30 DAA is characterized by the unique expression of enzymes associated with energy metabolism, desiccation and cytoskeletal functioning.
Thus, these methods developed in the author’s laboratory are suitable for optimizing the extraction of OBs membrane proteins (carbonate-washed fraction) and to analyze oleosin expression (urea-washed fraction) associated with in vivo demonstration of proteolyitc degradation of OB membranes in cotyledons during seedling growth. Furthermore, characterization of OB membrane proteins expressed during late seed filing (dessication) stage demonstrates an increment in metabolic and enzyme activity
Localization of OBs and PSVs: OB matrix is primarily composed of neutral lipids which can be visualized by the use of specific fluorescent probe – Nile red. Nile red is a heterocyclic uncharged non-fluorescent lipid phenoxazone molecule which fluoresces upon interaction with lipids. The range (580 nm; 650 nm) and intensity of its fluorescence emission depends upon the hydrophobicity of the environment, concentration of the dye and nature of its interaction with hydrophobic and amphiphatic lipid moieties in plant tissues. Methods developed in the author’s laboratory have been exploited to detect lipid composition dependent varying fluorescence (yellowish-green, green or red) emnating from OBs in the nile red-treated viable protoplasts from hypocotyls, root and cotyledons of sunflower and safflower seedlings.Citation55,Citation56 Variations in the nature of fluorescence indicate changes in the composition of OB-associated neutral lipids in protoplasts from different tissues. OB localization in sunflower seedling cotyledons reveals the parietal position of OBs in the cells, which tend to degenerate earlier due to increased lipolytic events. PSVs are commonly associated with cotyledons and they contain a variety of storage molecules, namely globulin proteins, lectins, acid phosphatases and cysteine proteases.Citation55 Acidification of PSV lumen is associated with mobilization of storage compounds during seed germination. Low pH (4.0) in the PSVs triggers the activity of aspartic and cysteine proteases, which further regulate various metabolic activities across these storage bodies. BCECF-AM is a lipophilic acetoxymethyl ester (non-fluorescent) which can penetrate plant protoplasts and shows negligible leakage and toxicity. The non-fluorescent ester of this probe is enzymatically cleaved by esterases in the cells to produce fluorescent anions of BCECF (ex. 482 nm; em. 520 nm). Using this probe, the size and number of PSVs can be estimated in protoplasts.Citation55 Interesting correlations have been obtained between the decrease in the size and number of PSVs and peripherally localized OBs in the cotyledons of 4 d old seedlings. Furthermore, in seedlings at later stages of development, number of PSVs reduces and they tend to coalesce forming vegetative vacuole. Thus, fluorescent localization of OBs and PSVs in seedling cotyledon protoplasts has demonstrated that reduction in PSVs precedes the mobilization of OBs in sunflower seeding cotyledons.
OB-associated thiol protease activity in protoplasts: The activity of regulatory thiol proteases on OB membrane is crucial for membrane degradation prior to mobilization of TAGs from the OB matrix. Proteolytic processing of oleosins results in higher susceptibility of OBs to lipase activity. Using a novel fluorescence approach, sunflower seedling cotyledon protoplasts are labeled in vivo with fluorescein mercuric acetate (FMA), a specific fluorescent probe (ex. 499 nm; em. 523 nm) which binds to – SH-proteases.Citation7 FMA is taken up by the cells and binds to – SH-proteases at their intracellular activity sites, thus causing emission (em. 525 nm) of fluorescence upon excitation at 485 nm. The fluorescence obtained due to thiol-protease activity has been found to be associated with OBs, and is thus, probably involved in the degradation of oleosins. Furthermore, the fluorescent localization of thiol protease on OBs using FMA, supported by zymographic detection of a 65 kDa cytosolic thiol protease activity highlights proteolytic degradation of oleosins. Fluorometric assay further revealed the binding of FMA with gel-eluted thiol protease, which was confirmed by spectral shift in the emission of FMA.
Phospholipase (PLA2) activity: Phospholipases catalyze the degradation of phospholipids. PLA2 specifically brings about phospholipid hydrolysis at sn-2 position and produces fatty acid and lysophospholipid. A novel fluorescent method using the probe PED6, has been developed in the author’s laboratory to localize the activity of PLA2 associated with OBs in sunflower seedling cotyledon protoplasts.Citation8 The report highlights the use of the fluorogenic substrate for detection of PLA2 in plant protoplasts. PED6 is a fluorogenic substrate which incorporates a BODIPY FL-dyelabeled-sn-2-acyl chain and a dinitrophenyl quencher group. The PED6-labeled acyl chain is cleaved by the activity of PLA2, which results in the formation of lysophospholipid (non-fluorescent) and fluorescent fatty acid tagged to the fluorophore-Bodipy FL (ex. 505 nm). The fluorescence method thus developed is effective in detection of PLA2 activity sites on OB surface in protoplasts. The activity sites for PLA2 have been found to be associated with OB surface in early stages of seedling development, prior to initiation of lipase activity.Citation8 Apart from OB surface, PLA2 fluorescence is also associated with cytosol around the OB, thus indicating the role of PLA2 in the hydrolysis of OB membrane phospholipids. This renders TAG matrix of OBs susceptible to lipolytic degradation. PED6 has also been used for localization of PLA2 activity sites in protoplasts of germinating safflower and cotton seeds.
Lipase activity: Measurement of lipase activity in oil seed homogenates imposes hurdles due to the presence of lipase inhibitors getting released during tissue homogenization. In view of this limitation, a novel fluorescence protocol has been developed in the author’s laboratory to perform in vivo localization of lipase activity in protoplasts from sunflower cotyledons. This is a nondestructive method which implies the use of a glycerol derivative – resorufin ester (1,2-O-dilauryl-rac-glycerol-3-glutaric acid-resorufin ester.Citation6,Citation19 The non-fluorescent resorufin ester is lipophilic in nature and thus permeates the plasma membrane. Following its uptake in cells, it is cleaved by lipase at the intracellular activity sites, thus liberating the fluorescent resorufin (ex. 569 nm, em. 584 nm). The demonstration of lipase activity using this probe has been performed in dark-grown seedling cotyledons of sunflower, cotton and peanut. Cotyledons of etiolated seedlings reduce the chances of spectral interference caused due to chloroplast autofluorescence in the range of red emission wavelength. The excitation and emission maxima of resorufin exhibit negligible changes due to pH. Localization of lipase activity sites on OB membrane surface correlates with OB (Nile red stained) mobilization and PSV degradation (BCECF-AM-stained). Thus, spatial and temporal changes in lipase activity can be monitored along the changes in PSV degradation and oil body mobilization.
Putative calcium channels (phenylalkylamine-binding sites): Calcium channels are expected to be involved in the regulation of various biomolecules. In view of the fact that Ca2+ channels in plants are similar to the L-type channels characterized in animals, the fluorescence method of co-localization of putative calcium channels and OBs from the author’s laboratory provided first report of that kind.Citation56 The fluorescence probe being employed in this investigation is DM-Bodipy-PAA (ex. 504 nm) which liberates bright green fluorescence upon binding with Ca2+ channel proteins in the scytosol and on tonoplast and OB membranes. Co-localization of putative calcium channels and OBs is performed using the probes DM-Bodipy-PAA and nile red, respectively due to the different excitation and emission ranges of the two probes. The neutral lipid accumulating sites (nile red positive) and PAA binding sites in the OBs (DM-Bodipy-PAA positive) reveal the signaling role of putative PAA-binding calcium channels. The role of calcium flux across the OB membranes is possibly related to upregulation of calcium-induced signaling events – namely thiol protease activity, lipoxygenase, lipase activity and caleosin expression. Thus, these finding provide scope for the detailed investigations on the role of calcium channels in OB-associated signaling events during lipolysis.
NO estimation and localization, and NO scavenging: Although DAF has been extensively used for NO detection in plants, DAF-mediated fluorescence and NO estimation procedures possess various limitations. DAF does not detect NO directly in the extract or tissue preparations, but detects the oxidized form of NO, i.e., N2O3, under oxygenic conditions. The formation of fluorescent derivative (DAF-2 T) is pH sensitive and DAF is susceptible to nucleophilic attack by OH−, thus reducing the chances of DAF-2 T formation. Various other factors like pH, ionic strength of the buffer and presence of ascorbic acid, are also likely to provide false positive results with DAF application. In view of the above limitations, MNIP-Cu [Cu derivative of 4-methoxy-2-(1 H-napthol [2,3- d (imidazol-2-yl) phenol] has been synthesized and used as an alternative novel probe for NO detection in plant tissues. This novel method devised in the author’s laboratory involves a two-step synthesis, characterization by NMR and its application for NO detection in various physiological investigations. MNIP-Cu (ex. 330–385 nm; em. 420–500 nm) enables more rapid and specific binding to NO in plant tissues. Moreover, in vivo detection of NO by using this probe does not involve any time lag and it is applicable in both anoxic and oxygenic conditions. Various plant tissues from sunflower (whole seedlings, hypocotyl segments, stigma from capitulum, protoplasts and isolated oil bodies) have been tested for detection of NO using MNIP-Cu. In this context, the probe has been observed to be relatively nontoxic at concentrations up to 50 µM. Imaging of whole sunflower seedlings (grown in the absence and presence of NaCl) for NO detection by this method revealed differences in NO fluorescence in various regions of root and cotyledon.Citation57 Similarly, adventitious roots in hypocotyl explants, protoplasts, stigma papillae and OBs have also been successfully used for NO detection by MNIP-Cu.Citation58
cPTIO has often been used as a potent scavenger of NO. cPTIO has limitations for its role as a NO scavenger since it is known to oxidize NO, thus producing NO2 radical, which, in turn, reacts with NO to form N2O3. Thus, instead of functioning as a NO quencher, cPTIO might contribute to further NO production. In view of such limitations, hemoglobin has been used as a potent NO scavenger in methods developed for NO localization by MNIP-Cu in the author’s laboratory.Citation32 Non-symbiotic hemoglobin is ubiquitous in occurrence and plays regulatory role in various aspects of plant metabolism. Deoxygenated hemoglobin (with ferrous heme iron) can bind to NO, thus functioning as a potent scavenger. In this report, methemoglobin formation (hemoglobin combined with NO) has also been analyzed by observing its spectral shift from 415 nm to 406 nm. CLSM analysis of 2d old dark-grown seedling roots incubated with hemoglobin and MNIP-Cu has revealed the efficiency of hemoglobin as a good choice for NO scavenging.
Oubain-sensitive ATPase: Sodium influx into the root cells and its sequestration or long distance transport, are highly regulated. Influx of sodium ions into the root cells mostly occurs by passive diffusion, mediated by ‘Nonspecific Cation Channels’ (NSCCs) in the plasma membrane and some ‘High-Affinity Potassium Transporters’ (HKTs).Citation59 Over the past few decades, plant scientists have been assuming that plants possess ouabain-sensitive ATPases, which might share some functional similarity with Na+/K+ ATPase in animals. However, further investigations on this ATPase could not make progress due to lack of suitable methodology in late nineties. Genomic sequence data confirmed the absence of Na+/K+ ATPase in plants. This, however, have not ruled out the possibility of finding ouabain-sensitive ATPases in plants having functional homology to Na+/K+ ATPase. Among the various mechanisms known to block Na+ influx into the plant cells, NaCl stress is likely to enhance ouabain (OU)-sensitive ATPase activity, thereby maintaining ion homeostasis. Ouabain (OU), a well-known cardiac glycoside, is known to be a specific inhibitor of Na+,K+-ATPase activity in animals.
Based on the already known possibilities of the presence of ouabain-sensitive ATPase in plants, a novel fluorescence imaging approach was developed in the author’s laboratory to localize and monitor the impact of NaCl stress on ouabain-sensitive ATPase activity during the early phase of seedling growth in sunflower.Citation60 In this context, 9-anthroylouabain (a fluorescent probe known to localize ouabain-sensitive ATPase activity in animal cells) has been used in plant systems for the first time. OU–sensitive ATPase activity in the root homogenates was estimated spectrofluorometrically by incubating with 9-anthroylouabain (AO). AO exhibits a spectral shift in its fluorescence emission spectrum (from 425 nm to 460 nm) and subsequent enhancement of fluorescence intensity due to its interaction with the target sites of the enzyme. CLSM imaging of salt-treated seedling root tips showed significantly enhanced fluorescence due to OU-sensitive ATPase activity. The enzyme activity is observed in columella, epidermis, subepidermal cells and meristematic cells. The presence of putative ouabain-sensitive enzyme activity in the nuclear and plasma membrane of sunflower seedling roots is a manifestation of the impact of salt stress. Furthermore, a correlation is evident between ouabain-induced inhibition of the ATPase activity and intracellular sodium accumulation in root protoplasts. The fluorescence methodology thus developed strengthens the hypothesis that plants seem to possess ouabain-sensitive ATPases. Further work is necessary for the characterization of the putative enzyme but present findings provide promising scope in understanding the mechanism of action of ouabain-sensitive ATPase in plants, which can regulate sodium accumulation during salt-stress.
Perspective
It is evident from the above analysis of major findings using sunflower as a model plant system that quite a few unique and several common biochemical routes and signaling mechanisms govern pollen-stigma interaction, seed development, seedling growth and management of salt stress. Redox modulation by ROS scavenging enzymes seems to have been significantly controlled by nitric oxide through molecular interactions.Citation36 In fact, NO has been discovered as a key molecule affecting salt stress related cascade of biochemical events. provides a comparative overview of differential expression of NO, ROS and PTMs in the roots and cotyledons of salt-tolerant and salt-sensitive sunflower seedlings. Future work holds promise in the following directions: 1. Understanding long distance transport of NOMela through vasculature will provide information on cell specific affinity of NO and melatonin and its impact on development. 2. Analyzing the possible role of iron-NO complexes in modulating the action/activity of iron-containing enzymes and non-enzymatic proteins which, in turn, affect developmental processes. 3. Analysis of elemental and biochemical markers of pollen-stigma interaction can be analyzed by surface plasma resonance to decipher actual molecular interactions governing the interactions between these two systems.Citation14,Citation61
Figure 3. An overview of differential expression pattern of NO, ROS, post-translational protein modifications (tyrosine nitration and S-nitrosylation) and organeller abundance in 2 d old salt-tolerant and sensitive seedlings in response to salt stress, NO donor and NO scavenger
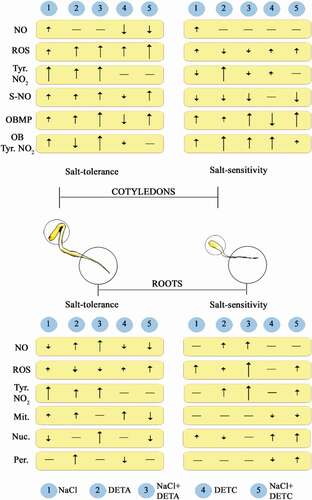
Acknowledgments
Authors are thankful to all the scholars from the laboratory of Professor. Satish C. Bhatla for their immense efforts in understanding the physiology of sunflower growth and development. Collaboration with Professor Dr. Frantisek Baluska (Institute for Cellular and Molecular Biology, University of Bonn, Germany) provided significant addition of new information in this work.
Disclosure statement
No potential conflict of interest was reported by the author(s).
Additional information
Funding
References
- Bhatla SC, Kaushik V, Yadav MK. Use of oil bodies and oleosins in recombinant protein production and other biotechnological applications. Biotechnol Adv. 2010;28(3):1–15. doi:https://doi.org/10.1016/j.biotechadv.2010.01.001.
- Kalra G, Bhatla SC. Auxin-ethylene interaction in adventitious rooting and related changes in anodic peroxidase isozymes in sunflower hypocotyls. J Plant Biochem Biotechnol. 1996;5(1):37–41. doi:https://doi.org/10.1007/BF03262977.
- Kalra G, Bhatla SC. Auxin-calcium interaction in adventitious root formation in the hypocotyl explants of sunflower (Helianthus annuus L.). J Plant Biochem Biotechnol. 1998;7(2):107–110. doi:https://doi.org/10.1007/BF03263045.
- Sadeghipour HR, Bhatla SC. Light-enhanced oil body mobilization in sunflower seedlings accompanies faster protease action on oleosins. Plant Physiol Biochem. 2003;41(4):309–316. doi:https://doi.org/10.1016/S0981-9428(03)00024-X.
- Sadeghipour HR, Bhatla SC. Differential sensitivity of oleosins to proteolysis during oil body mobilization in sunflower seedlings. Plant Cell Physiol. 2002;43(10):1117–1126. doi:https://doi.org/10.1093/pcp/pcf142.
- Gupta A, Bhatla SC. Spatial and temporal changes in lipase activity sites during oil body mobilization in protoplasts from sunflower seedling cotyledons. Plant Growth Regul. 2005;46(1):11–17. doi:https://doi.org/10.1007/s10725-005-5231-x.
- Vandana S, Bhatla SC. Evidence for the probable oil body association of a thiol-protease, leading to oleosin degradation in sunflower seedling cotyledons. Plant Physiol Biochem. 2006;44(11–12):714–723. doi:https://doi.org/10.1016/j.plaphy.2006.09.022.
- Gupta A, Bhatla SC. Preferential phospholipase A2 activity on the oil bodies in cotyledons during seed germination in Helianthus annuus L. cv. Morden Plant Sci. 2007;172(3):535–543. doi:https://doi.org/10.1016/j.plantsci.2006.11.008.
- Yadav MK, Bhatla SC. Localization of lipoxygenase activity on the oil bodies and in protoplasts using a novel fluorescence imaging method. Plant Physiol Biochem. 2011;49(2):230–234. doi:https://doi.org/10.1016/j.plaphy.2010.11.012.
- Yadav M, Bhatla SC. Photomodulation of fatty acid composition and lipoxygenase activity in sunflower seedling cotyledons. Acta Physiol Plant. 2015;37(8):147. doi:https://doi.org/10.1007/s11738-015-1891-y.
- Gogna M, Bhatla SC. Biochemical mechanisms regulating salt tolerance in sunflower. Plant Signal Behav. 2019;14(12):1670597. doi:https://doi.org/10.1080/15592324.2019.1670597.
- Shakya R, Bhatla SC. A comparative analysis of the distribution and composition of lipidic constituents and associated enzymes in pollen and stigma of sunflower. Sex Plant Reprod. 2010;23(2):163–172. doi:https://doi.org/10.1007/s00497-009-0125-0.
- Sharma B, Bhatla SC. Structural analysis of stigma development in relation with pollen–stigma interaction in sunflower. Flora - Morphol Distrib Funct Ecol Plants. 2013;208(7):420–429. doi:https://doi.org/10.1016/j.flora.2013.07.003.
- Sharma B, Bhatla SC. Elemental and biochemical markers of stigma receptivity in sunflower. Acta Physiol Plant. 2014;36(6):1299–1311. doi:https://doi.org/10.1007/s11738-014-1504-1.
- Yadav M, Bhatla SC. Oil body biogenesis and expression of structural and expression of structural and regulatory proteins during seed development in sunflower. In: Gorawala P, Mandhatri, editors. SAgricultural Research Updates. Vol. 34 Nova Science Publishers Inc; New York, USA: 2021. p. 1–39.
- Kaushik V, Yadav MK, Bhatla SC. Temporal and spatial analysis of lipid accumulation, oleosin expression and fatty acid partitioning during seed development in sunflower (Helianthus annuus L.). Acta Physiol Plant. 2010;32(1):199–204. doi:https://doi.org/10.1007/s11738-009-0378-0.
- Thakur A, Bhatla SC. Proteomic analysis of oil body membrane proteins accompanying the onset of desiccation phase during sunflower seed development. Plant Signal Behav. 2015;10(12):e1030100. doi:https://doi.org/10.1080/15592324.2015.1030100.
- Thakur A, Bhatla SC. A probable crosstalk between Ca2+ reactive oxygen species accumulation and scavenging mechanisms and modulation of protein kinase C activity during seed development in sunflower. Plant Signal Behav. 2014;9(2):e27900. doi:https://doi.org/10.4161/psb.27900.
- Gupta A, Sadeghipour HR, Bhatla SC. Subcellular detection of lipase activity in plant protoplasts using fluorescence microscopy. Plant Growth Regul. 2003;41(3):259–264. doi:https://doi.org/10.1023/B:GROW.0000007525.48895.f5.
- Yadav S, David A, Bhatla SC. Nitric oxide modulates specific steps of auxin-induced adventitious rooting in sunflower. Plant Signal Behav. 2010;5(10):1163–1166. doi:https://doi.org/10.4161/psb.5.10.12159.
- Bharti N, Tripathi S, Bhatla SC. Photomodulation of strigolactone biosynthesis and accumulation during sunflower seedling growth. Plant Signal Behav. 2015;10(8):e1049792. doi:https://doi.org/10.1080/15592324.2015.1049792.
- Yadav S, David A, Baluška F, Bhatla SC. Rapid auxin-induced nitric oxide accumulation and subsequent tyrosine nitration of proteins during adventitious root formation in sunflower hypocotyls. Plant Signal Behav. 2013;8(3):e23196. doi:https://doi.org/10.4161/psb.23196.
- Bharti N, Bhatla SC. Nitric oxide mediates strigolactone signaling in auxin and ethylene-sensitive lateral root formation in sunflower seedlings. Plant Signal Behav. 2015;10(8):37–41. doi:https://doi.org/10.1080/15592324.2015.1054087.
- David A, Yadav S, Bhatla SC. Sodium chloride stress induces nitric oxide accumulation in root tips and oil body surface accompanying slower oleosin degradation in sunflower seedlings. Physiol Plant. 2010;140(4):342–354. doi:https://doi.org/10.1111/j.1399-3054.2010.01408.x.
- Ebrahimi R, Bhatla SC. Effect of sodium chloride levels on growth, water status, uptake, transport, and accumulation pattern of sodium and chloride ions in young sunflower plants. Commun Soil Sci Plant Anal. 2011;42(7):815–831. doi:https://doi.org/10.1080/00103624.2011.552657.
- Ebrahimi R, Bhatla S. Ion distribution measured by electron probe X-ray microanalysis in apoplastic and symplastic pathways in root cells in sunflower plants grown in saline medium. J Biosci. 2012;37(4):713–721. doi:https://doi.org/10.1007/s12038-012-9246-y.
- Hryvusevich P, Navaselsky I, Talkachova Y, Straltsova D, Keisham M, Viatoshkin A, Samokhina V, Smolich I, Sokolik A, Huang X, et al.Sodium influx and potassium efflux currents in sunflower root cells under high salinity. Front Plant Sci. 2021;11. doi:https://doi.org/10.3389/fpls.2020.613936.
- Gogna M, Bhatla SC. Plant signaling & behavior Salt-tolerant and-sensitive seedlings exhibit noteworthy differences in lipolytic events in response to salt stress. Plant Signal Behav. 2020;15(4):e–1737451. doi:https://doi.org/10.1080/15592324.2020.1737451.
- Gogna M, Choudhary A, Mishra G, Kapoor R, Bhatla SC. Changes in lipid composition in response to salt stress and its possible interaction with intracellular Na+-K+ ratio in sunflower (Helianthus annuus L.). Environ Exp Bot. 2020;178:104147. doi:https://doi.org/10.1016/j.envexpbot.2020.104147.
- Singh N, Bhatla SC. Nitric oxide regulates lateral root formation through modulation of ACC oxidase activity in sunflower seedlings under salt stress. Plant Signal Behav. 2018;13(5):e1473683. doi:https://doi.org/10.1080/15592324.2018.1473683.
- Singh N, Bhatla SC. Signaling through reactive oxygen and nitrogen species is differentially modulated in sunflower seedling root and cotyledon in response to various nitric oxide donors and scavengers. Plant Signal Behav. 2017;12(9):e1365214. doi:https://doi.org/10.1080/15592324.2017.1365214.
- Singh N, Bhatla SC. Hemoglobin as a probe for estimation of nitric oxide emission from plant tissues. Plant Methods. 2019;15(1):1–8. doi:https://doi.org/10.1186/s13007-019-0425-9.
- Arora D, Bhatla SC. Melatonin and nitric oxide regulate sunflower seedling growth under salt stress accompanying differential expression of Cu/Zn SOD and Mn SOD. Free Radic Biol Med. 2017;106(106):315–328. doi:https://doi.org/10.1016/j.freeradbiomed.2017.02.042.
- Arora D, Bhatla SC. Nitric oxide triggers a concentration-dependent differential modulation of superoxide dismutase (FeSOD and Cu/ZnSOD) activity in sunflower seedling roots and cotyledons as an early and long distance signaling response to NaCl stress. Plant Signal Behav. 2015;10(10):e1071753. doi:https://doi.org/10.1080/15592324.2015.1071753.
- Jain P, Bhatla SC. Signaling role of phospholipid hydroperoxide glutathione peroxidase (PHGPX) accompanying sensing of NaCl stress in etiolated sunflower seedling cotyledons. Plant Signal Behav. 2014;9(12):e977746–7. doi:https://doi.org/10.4161/15592324.2014.977746.
- Arora D, Jain P, Singh N, Kaur H, Bhatla SC. Mechanisms of nitric oxide crosstalk with reactive oxygen species scavenging enzymes during abiotic stress tolerance in plants. Free Radic Res. 2015;50(3):291–303. doi:https://doi.org/10.3109/10715762.2015.1118473.
- Singh N, Bhatla SC. Nitric oxide and iron modulate heme oxygenase activity as a long distance signaling response to salt stress in sunflower seedling cotyledons. Nitric Oxide. 2016;53:54–64. doi:https://doi.org/10.1016/j.niox.2016.01.003.
- Tailor A, Tandon R, Bhatla SC. Nitric oxide modulates polyamine homeostasis in sunflower seedling cotyledons under salt stress. Plant Signal Behav. 2019;14(11):1667730. doi:https://doi.org/10.1080/15592324.2019.1667730.
- Kumari A, Kapoor R, Bhatla SC. Nitric oxide and light co-regulate glycine betaine homeostasis in sunflower seedling cotyledons by modulating betaine aldehyde dehydrogenase transcript levels and activity. Plant Signal Behav. 2019;14(11):1666656. doi:https://doi.org/10.1080/15592324.2019.1666656.
- Tailor A, Bhatla SC. Polyamine homeostasis modulates plasma membrane- and tonoplast-associated aquaporin expression in etiolated salt-stressed sunflower (Helianthus annuus L.) seedlings. Protoplasma. 2021;258(3):661–672. doi:https://doi.org/10.1007/s00709-020-01589-8.
- Kumari A, Bhatla SC. Regulation of salt-stressed sunflower (Helianthus annuus) seedling’s water status by the coordinated action of Na+/K+ accumulation, nitric oxide, and aquaporin expression. Funct Plant Biol. 2021;48(6):573. doi:https://doi.org/10.1071/FP20334.
- Kaur H, Mukherjee S, Baluska F, Bhatla SC. Regulatory roles of serotonin and melatonin in abiotic stress tolerance in plants. Plant Signal Behav. 2015;10(11):e1049788. doi:https://doi.org/10.1080/15592324.2015.1049788.
- Mukherjee S, David A, Yadav S, Baluška F, Bhatla SC. Salt stress-induced seedling growth inhibition coincides with differential distribution of serotonin and melatonin in sunflower seedling roots and cotyledons. Physiol Plant. 2014;152(4):714–728. doi:https://doi.org/10.1111/ppl.12218.
- Kaur H, Bhatla SC. Melatonin and nitric oxide modulate glutathione content and glutathione reductase activity in sunflower seedling cotyledons accompanying salt stress. Nitric Oxide. 2016;59:42–53. doi:https://doi.org/10.1016/j.niox.2016.07.001.
- Singh N, Bhatla SC, Demidchik V. Plants and human beings engage similar molecular crosstalk with nitric oxide under stress conditions. Funct Plant Biol. 2019;46(8):695–701. doi:https://doi.org/10.1071/FP19018.
- Singh N, Kaur H. Does N-Nitrosomelatonin compete with S-Nitrosothiols as a long distance nitric oxide carrier in plants? Biochem Anal Biochem. 2016;s3(1):1–4. doi:https://doi.org/10.4172/2161-1009.1000262.
- Singh N, Jain P, Gupta S, Khurana JM, Bhatla SC. N-Nitrosomelatonin, an efficient nitric oxide donor and transporter in Arabidopsis seedlings. Nitric Oxide. 2021;9:113–114. doi:https://doi.org/10.1016/j.niox.2021.05.001.
- David A, Yadav S, Baluška F, Bhatla SC. Nitric oxide accumulation and protein tyrosine nitration as a rapid and long distance signalling response to salt stress in sunflower seedlings. Nitric Oxide. 2015;50:28–37. doi:https://doi.org/10.1016/j.niox.2015.08.003.
- Keisham M, Jain P, Singh N, von Toerne C, Bhatla SC, Lindermayr C. Deciphering the nitric oxide, cyanide and iron-mediated actions of sodium nitroprusside in cotyledons of salt stressed sunflower seedlings. Nitric Oxide. 2019;88:10–26. doi:https://doi.org/10.1016/j.niox.2019.03.008.
- Yadav S, David A, Bhatla SC. Nitric oxide accumulation and actin distribution during auxin-induced adventitious root development in sunflower. Sci Hortic (Amsterdam, Netherlands). 2011;129(1):159–166. doi:https://doi.org/10.1016/j.scienta.2011.03.030.
- Jain P, Bhatla SC. Molecular mechanisms accompanying nitric oxide signalling through tyrosine nitration and S-nitrosylation of proteins in plants. Funct Plant Biol. 2017;45(2):70–82. doi:https://doi.org/10.1071/FP16279.
- Jain P, Bhatla SC. Tyrosine nitration of cytosolic peroxidase is probably triggered as a long distance signaling response in sunflower seedling cotyledons subjected to salt stress. PLoS One. 2018;13(5):e0197132. doi:https://doi.org/10.1371/journal.pone.0197132.
- Arora D, Singh N, Bhatla SC. Electrophoretic detection and confocal microscopy imaging of tyrosine nitrated proteins in plant tissue. In: Mengel A, Lindermayr C, editors. Nitric oxide, Methods and Protocols. New York (USA): Humana Press; 2017. p. 171–182.
- Jain P, von Toerne C, Lindermayr C, Bhatla SC. S-nitrosylation/denitrosylation as a regulatory mechanism of salt stress sensing in sunflower seedlings. Physiol Plant. 2018;162(1):49–72. doi:https://doi.org/10.1111/ppl.12641.
- Bhatla SC, Vandana S, Kaushik V. Recent developments in the localization of oil body-associated signaling molecules during lipolysis in oilseeds. Plant Signal Behav. 2009;4(3):176–182. doi:https://doi.org/10.4161/psb.4.3.7799.
- Vandana S, Bhatla SC. Co-localization of putative calcium channels (phenylalkylamine-binding sites) on oil bodies in protoplasts from dark-grown sunflower seedling cotyledons. Plant Signal Behav. 2009;4(7):604–609. doi:https://doi.org/10.4161/psb.4.7.9165.
- Jain P, David A, Bhatla SC. A novel protocol for detection of nitric oxide in plants. In: Gupta KG, editor. Plant nitric oxide: Methods and protocols. Methods in molecular biology. New York (USA): Springer Science; 2016. p. 69–79.
- Sharma B, Bhatla SC. Accumulation and scavenging of reactive oxygen species and nitric oxide correlate with stigma maturation and pollen–stigma interaction in sunflower. Acta Physiol Plant. 2013;35(9):2777–2787. doi:https://doi.org/10.1007/s11738-013-1310-1.
- Keisham M, Mukherjee S, Bhatla SC. Mechanisms of sodium transport in plants—Progresses and challenges. Int J Mol Sci. 2018;19(3):647. doi:https://doi.org/10.3390/ijms19030647.
- Mukherjee S, Bhatla SC. A novel fluorescence imaging approach to monitor salt stress-induced modulation of ouabain-sensitive ATPase activity in sunflower seedling roots. Physiol Plant. 2014;150(4):540–549. doi:https://doi.org/10.1111/ppl.12101.
- Jain P, Arora D, Bhatla SC. Surface plasmon resonance based recent advances in understanding plant development and related processes. Biochem Anal Biochem. 2016;5(4). doi:https://doi.org/10.4172/2161-1009.1000300.