ABSTRACT
Necrosis and ethylene-inducing peptide 1 (Nep1) -like proteins (NLP) are secreted by multiple taxonomically unrelated plant pathogens (bacteria, fungi, and oomycete) and are best known for inducing cell death and immune responses in dicotyledonous plants. A group of putative NLP genes from obligate biotrophic oomycete Plasmopara viticola were predicted by RNA-Seq in our previous study, but their activity has not been established. Therefore, we analyzed the P. viticola NLP (PvNLP) family and identified seven PvNLP genes. They all belong to type 1 NLP genes and form a P. viticola-specific cluster when compared with other pathogen NLP genes. The expression of PvNLPs was induced during early infection process and the expression patterns could be categorized into two groups. Agrobacterium tumefaciens-mediated transient expression assays revealed that only PvNLP7 was cytotoxic and could induce Phytophthora capsici resistance in Nicotiana benthamiana. Functional analysis showed that PvNLP4, PvNLP5, PvNLP7, and PvNLP10 significantly improved disease resistance of Arabidopsis thaliana to Hyaloperonospora arabidopsidis. Moreover, the four genes caused an inhibition of plant growth which is typically associated with enhanced immunity when over-expressed in Arabidopsis. Further research found that PvNLP7 could activate the expression of defense-related genes and its conserved NPP1 domain was critical for cell death- and immunity-inducing activity. This record of NLP genes from P. viticola showed a functional diversification, laying a foundation for further study on pathogenic mechanism of the devastating pathogen.
Introduction
Plants have evolved a complicated and multilayered immune system to monitor and cope with infection by diverse phytopathogens. The first layer of plant surveillance system invokes the recognition of evolutionarily conserved molecular signatures derived from various microbes, termed pathogen- or microbe-associated molecular patterns (PAMPs/MAMPs), by employing pattern recognition receptors (PRRs).Citation1 A large set of defense responses are subsequently triggered including accumulation of reactive oxygen species (ROS), reinforcement of cell walls, production of antimicrobial substances, activation of mitogen-activated protein kinase (MAPK) cascades, and increased levels of defense-related hormones,Citation2–4 collectively resulting in plant resistance to pathogens which is also referred to as PAMP/MAMP-triggered immunity (PTI/MTI). A wide range of microbial patterns or components have been identified and verified as MAMPs from bacteria, fungi, and oomycetes.Citation5 However, for virtually MAMPs identified from different kingdoms of life are distinct. For example, flagellin,Citation6 EF-TUCitation7 and peptidoglycanCitation8 are three characterized bacterial MAMPs. Chitin and elicitins act as MAMPs which are secreted from fungi and oomycetes, respectively. Intriguingly, a class of necrosis and ethylene-inducing peptide 1 (Nep1)-like proteins (NLPs) have been described as MAMPs from mostly phytopathogens across all three of the taxonomically unrelated groups.Citation9–11
The founding member of NLPs, a 24-kDa protein, was isolated in 1995 from Fusarium oxysporum culture filtrates based on its capability to induce necrosis and ethylene biosynthesis in numerous dicotyledonous but not in monocotyledonous plants, therefore named after necrosis and ethylene inducing peptide 1 (Nep1).Citation12 Since then, an emerging group of Nep and Nep1-like proteins (NLPs) were identified from various phytopathogenic microorganisms including bacteria, fungi, and oomycete by the presence of a common NPP1 (necrosis-inducing Phytophthora protein 1) domain.Citation13–17 A hallmark of conserved NPP1 domain is a heptapeptide motif “GHRHDWE” in the central region of the NLPs and two to six cysteine residues. Base on the number of cysteine residues, NLPs can be classified into types 1, 2, and 3, which possess two, four, and six cysteine residues, respectively.Citation9,Citation18 In addition, type 1 NLPs contain a noncytotoxic subgroup of NLPs (designated type 1a) which can be distinguished according to the amino acid substitution in the cation-binding pocket.Citation18 The number of NLP gene family members can vary significantly among diverse taxonomy of microorganisms. For instance, the Arabidopsis pathogen Hyaloperonospora arabidopsidis have 12 NLP genes, and soybean pathogen Phytophthora sojae have a total number of 33 NLP members, whereas only a single NLP gene has been identified from the genome of wheat pathogen Mycosphaerella graminicola.Citation19–21 Comparative genome analysis among the already fully sequenced genomes of a broad range of oomycetes and fungi revealed that the NLP family in oomycetes is more expanded than in fungi pathogens.Citation19
It is well known that NLPs are capable of stimulating plant immunity-associated defenses in dicotyledonous but not in monocotyledonous plants.Citation22–24 Thus, NLPs were proposed to have dual function in plant-pathogen interaction, i.e., activating immune responses and acting as a toxin-like virulence factor which inducing plant necrosis.Citation25 Cytotoxic NLPs were found to trigger defense-related genes expression in Arabidopsis thaliana, which clearly overlaps with that activated by flg22,15,Citation23 suggesting the similarity of cytotoxic NLP-triggered immune response to the MAMP-triggered immunity (MTI). Furthermore, cytotoxic NLPs also induce a myriad of other defense responses in plants including activation of mitogen-activated protein (MAP) kinase activity, deposition of callose and increased production of reactive oxygen species.Citation20,Citation23,Citation26 However, not all NLPs have the ability to induce necrosis which are called noncytotoxic proteins. When tested by transient expression in tobacco, necrosis was only induced by 2 out of 9 NLPs from Verticillium dahlia,Citation27 8 out of the 30 NLPs of Phytophthora sojae,Citation21 whereas none of the 10 tested NLPs of H. arabidopsidis were able to cause necrosis, including the host A. thaliana.Citation19 These observations indicate that NLPs may have roles independent of cytotoxicity. For the hemibiotrophic pathogens P. sojae and P. infestans, cytotoxic NLPs were expressed during the infection stages coinciding with the transition from biotrophy to necrotrophy.Citation28,Citation29 In contrast to cytotoxic NLPs which are mainly detected at the necrotrophic stages of infection, most noncytotoxic NLPs appear to be expressed during early stages of infection,Citation19,Citation28 suggesting they may function in penetration or initial colonization of the host. Several observations showed that NLPs from different pathogens play distinct roles in pathogen virulence. In some cases, NLPs were regarded as positive virulence factors that facilitated pathogen growth in hosts through disintegration of host´s membrane, resulting in serious plant diseases. The virulence of Colletotrichum coccodes was increased by transformed with NEP1 gene from Fusarium oxysporum and the spectrum of hosts also be infected.Citation30 Also, transformation of the NLP-deficient mutants of Pectobacterium carotovoum with NLPPya or NLPPp showed 30%-40% restoration in virulence and maceration of potato tuber.Citation31 The deletion of NLP in Erwinia carotovora attenuated virulence on both rubbers and stems of potato.Citation32 However, NLP-deficient mutants of Botrytis elliptica, Mycosphaerella graminicola, Verticillium dahliae, and Magnaporthe oryzae were not reduced in virulence on each host, indicating NLP genes are not virulence determinants in these plant pathogen interactions.Citation20,Citation24,Citation26,Citation27
In the current publication we provide an analysis of the potential biological roles of the 7 PvNLP genes that have been previously identified in Plasmopara viticola, the causal agent of grapevine downy mildew, whose transcriptome- and genome-sequencing were completed in our previous work.Citation33,Citation34 The objectives of this study were to define variation in their function, to use agroinfiltration assays to determine whether any of them play important roles in cell death-inducing activity or immune responses, and to generated PvNLP-expressing Arabidopsis plants to determine whether any of them have the ability to trigger immunity as a MAMP.
Materials and methods
Plant material, strains, and growth condition
The grapevine (V. vinifera cv. Thompson seedless) and Nicotiana benthamiana used in this study was grown in a greenhouse at 25°C with 16 h of illumination per day. The Arabidopsis thaliana (Col-0) were cultivated in a phytochamber with a photoperiod of 22°C/16 h light and 16°C/8 h darkness under 40%-60% humidity. Highly virulent Plasmopara viticola strain ZJ-1-1 has been tested in our previous study and routinely subcultured on grapevine leaf discs every 10 days at 22°C/16 h light and 16°C/8 h dark cycles. Agrobacterium tumefaciens strain GV3101 carrying the disarmed Ti plasmid was cultured on Luria-Bertani (LB) agar or broth medium at 28°C.
RNA isolation, cDNA synthesis and quantitative RT-PCR
To monitor PvNLPs transcript profiling during P. viticola infection of grapevine, leaf inoculation using spore drops of P. viticola ZJ-1-1 and total RNA was extracted using the CTAB procedure were conducted as previous described.Citation35 For RNA isolation of N. benthamiana, a commercial kit (RNA simple Total RNA Kit, Tiangen) was used following the recommended protocols. All cDNA synthesis and quantitative RT-PCR reaction were performed by using protocols established in our lab. Briefly, the total RNA was used as a template for reverse transcription with a RevertAid TM First Strand cDNA Synthesis Kit (Invitrogen, Carlsbad, CA, USA). The qRT-PCR was performed on the QIAGEN Rotor-Gene Q system (QIAGEN, Hilden, Germany) using a SuperReal PreMix Plus (SYBR Green) kit (TIANGEN Biotech Co., Ltd., Beijing, China). Primers (Table S1) were designed to anneal specifically to each targeted gene and by using Beacon Designer 8.14 software with default setting for SYBR Green real-time PCR. The PvActin geneCitation36 and NbEF1α geneCitation35 were used as constitutively expressed endogenous controls to determine relative expression values by the 2−ΔΔCt methodCitation37 for P. viticola and N. benthamiana, respectively.
Gene cloning and sequence analysis
The coding sequences of the PvNLPs were amplified using cDNA from P. viticola isolate ZJ-1-1 based on our previous RNA-seq results,Citation33 cloned into a pLB vector ((Tiangen, Beijing) and verified by PCR and Sanger sequencing. The gene-specific primers for PvNLPs cloning are listed in the Table S1. The potential signal peptides of PvNLPs were predicted with online software SignalP 5.0 (http://www.cbs.dtu.dk/services/SignalP/). Multiple sequence alignment of NLPs from three different kingdoms of life was carried out using software MEGA 7.0 (Oxford University, England, UK). The phylogenetic relationship analysis of three different types of NLPs was done using the Neighbor-Joining method,Citation38 with 1000 bootstrap replicates.
Construction of expression plasmids
The open reading frame sequences of seven genes were amplified from the pLB-PvNLPs with primers containing restriction enzyme cutting site (Table S1). To make the stable expression recombinant vectors pHB-PvNLPs-flag, the PCR fragments were digested with Bgl II/ Bcl I and Spe I restriction enzymes which are isocaudarners of BamH I and Xba I, respectively. The binary vectors pHB-flag was cut using BamH I and Xba I restriction enzymes. Then the digestion fragments of PvNLPs were ligated into the digested pHB-flag vectors. To generate the pGR106-PvNLPs-flag for PVX and Western blot assays, the fragments harboring flag tag were amplified from pHB-PvNLPs-flag and cut with Asc I and Not I restriction enzymes and inserted into the pGR106 vector. All the generated plasmids were verified by Sanger sequencing by Majorbio, Inc. (Shanghai, China).
Agrobacterium-mediated transient expression in Nicotiana benthamiana and pathogen infection assays
Constructs were transformed into A. tumefaciens strain GV3101 by electroporation and cultured in LB medium supplemented with the appropriate antibiotics (12.5 μg/ml tetracycline, 50 μg/ml kanamycin and 50 μg/ml rifampicin) at 28°C for 2 days.Citation39 For cytotoxicity assay, Agrobacteria harboring constructs of pGR106-PvNLPs were cultured in LB broth medium containing 50 μg/mL of kanamycin and 12.5 μg/ml of tetracycline at 28°C with shaking at 200 rpm for 60 h. The culture was harvested by centrifugation and washed three times using 10 mM MgCl2, then resuspended in 10 mM MgCl2 to achieve a final OD600 of 0.4. After incubation for 1 h at 28°C, then 1 mL of A. tumefaciens cell suspension was infiltrated into plant leaves by using needleless syringes. Symptom development was monitored 4–8 dpi in N. benthamiana. Each assay consisted of at least three plants (40 days old) inoculated on four leaves. For Phytophthora capsici infection, zoospores were prepared as described and the infection assays were conducted as our previous study.Citation40,Citation41 Lesion diameters were determined by manually measuring with vernier caliper.
Electrolyte leakage assays
Cell death of N. benthamiana leaves was quantitatively assessed by measuring ion leakage of leaf discs as previously described.Citation42 In brief, for each sample, three leaf discs (8 mm diameter) cut from inoculated leaves using a cork borer were placed into a 50-ml tube containing 5 ml sterile and double-distilled water for 3 h at room temperature (RT). Then the conductivity EC1 of the bathing solution was measured using the sensor of an electrical conductivity meter (DDS-307, Rex Shanghai, China). The tubes containing the solution and leaf disks were then boiled for 25 min. After cooling to room temperature, the conductivity EC2 of the solution was measured. Electrolyte leakage (%) = 100 × EC1/EC2. These experiments were repeated for a total of three times.
Western blot assays
Agroinfiltrated N. benthamiana leaves were harvested at 2 dpi and homogenized in liquid nitrogen. And then 1 mL lysis buffer was added to 600 mg of each ground sample. The samples were mixed gently and centrifuged at 12500 rpm for 10 min at 4°C. Supernatants were obtained and separated by 12% sodium dodecyl sulfate-polyacrylamide gel electrophoresis (SDS-PAGE) and transferred to a nitrocellulose blotting membrane. Western blot analysis was conducted using an anti-cFlag peroxidase conjugate (Sigma-Aldrich).
A. thaliana stable transformation and pathogenicity assays
Agrobacterium tumefaciens carrying the empty vector pHB-flag or pHB-PvNLP-flag was cultured, harvested and resuspended in a solution of 5% sucrose and 0.02% Silwet L-77 (Lablead Biotech Co., Ltd., Beijing, China). Arabidopsis wild-type plants (Col-0) were transformed by dipping flowers into the suspension as described previously.Citation43 The hygromycin-resistant seedlings were screened on selective medium containing 30 μg/mL of hygromycin (Sigma-Aldrich) for 2 weeks and transplanted in soil. Positive transgenic plants were validated by semi-quantitative PCR.
Infection assays on Arabidopsis seedlings were performed with downy mildew pathogen Hyaloperonospora arabidopsidis (50 spores per μL). To collect spores for inoculation, wild-type (Col-0) leaves with sporulating Hyaloperonospora arabidopsidis were harvested 7 dpi and washed with deionized H2O by vortexing in tubes. The spore suspension was then filtered and sprayed onto the 14-days-old plants of transgenic and wild-type Arabidopsis using a mini spraying device. Subsequently, plants were left to dry for about 30 minutes and cultured at 100% humidity under long day conditions (16 h light, 18°C). The disease severity or zoospores growth was quantified 6 dpi. For zoospores counting, the aerial parts of seedlings were cut and suspended in a known volume of water and the total number of zoospores per milligram of plant tissue was measured.
Results
Identification of the NLP family in P. viticola
In our previous work, the secretomes of three different P. viticola isolates (JL-7-2, ZJ-1-1 and CSIRO-L-2) were explored by de novo transcriptome analysis.Citation33 A total number of 17 NLP coding genes were predicted in these three P. viticola isolates. After removing duplicate genes and incorrect genes which have no typical conserved motifs of NLP proteins, there are 7 PvNLP putative genes left. Then the full-length of PvNLPs were cloned from the cDNA of pathogen isolate ZJ-1-1 for further functional evaluation. None of them has an intron which was confirmed by cloning genes from genome of P. viticola isolate ZJ-1-1. The protein sequences of these PvNLPs, ranging in size from 223 to 279 amino acids, were submitted to SignalP 5.0 for secreted signal peptide prediction. PvNLP4, PvNLP5, PvNLP7, PvNLP9 and PvNLP10 had a signal peptide consisting of 17 to 21 amino acid residues (Table S2), which was predicted to regulate the secreted proteins. Whereas PvNLP2 and PvNLP3 did not have a predicted signal peptide and therefore may not be secreted into the apoplast in native mycelia. In our research, PvNLP1, PvNLP6, and PvNLP8 were missing compared with the previous study.Citation44 It is possible that the expression of these three genes was not detected in our transcriptome or the transcriptome sequencing is not deep enough. On the basis of multiple sequence alignment of PvNLPs with other pathogen NLPs revealed they shared the conserved 24-aa peptide (nlp24) containing conserved region (AIMYAWYFPKD) and (GHRHDWE) at the C-terminal end (). These characteristics were demonstrated to be sufficient to trigger immune response in plants and can identify any new peptide sequence as an NLP in pathogens10−11.
Figure 1. Multiple sequence alignment of PvNLPs with other pathogens NLP proteins.
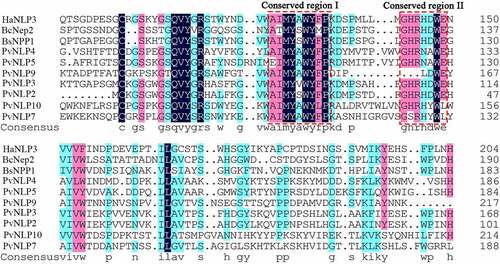
To determine the relationship of PvNLPs to the reported NLP subfamilies, a maximum likelihood phylogenetic analysis of PvNLPs was conducted together with representatives of type 1, type 2 and type 3 NLP proteins in various pathogens including fungi, oomycetes and bacteria. Phylogenetic analysis showed that PvNLPs were all grouped together with type 1 NLPs whereas type 2 and type 3 NLPs were separated into different groups (). These results indicating the seven PvNLPs most probably belong to type 1 NLP proteins which were found almost exclusively in plant-associated microbes, whereas type 2 and type 3 NLPs were found in microorganisms with different lifestyles.Citation10
Figure 2. Phylogenetic tree analysis of PvNLPs with other plant pathogen-derived necrosis- and ethylene-inducing protein (Nep1)-like proteins (NLP).
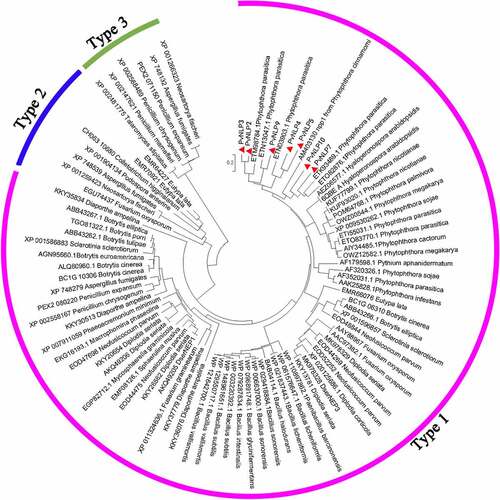
Expression profiles of PvNLP genes
To determine the potential role of PvNLPs during the interaction between P. viticola and its host plants, we inoculated susceptible grapevine leaves with strain ZJ-1-1, and analyzed transcriptional patterns for PvNLPs at different time points (0, 6, 12, 24, 48, 72, 96 and 120 hpi) after infection by quantitative real-time PCR. Results showed that all seven members of the PvNLP family were strikingly up-regulated during the early stages of infection process but with seemingly distinct activation patterns. Four genes (PvNLP2, PvNLP4, PvNLP7, and PvNLP9) were already highly expressed at 0 hpi before the interaction with the grapevine leaves. PvNLP5 and PvNLP10 were strongly induced at 6 hpi and reached a maximum level at 24 hpi. Whereas a significant transient induction of PvNLP3 were detected at a relatively late time point (24 hpi) (). According to their temporal expression patterns during infection, the PvNLPs genes could be grouped into two kinds. In general, the first group, comprising PvNLP2, PvNLP3, PvNLP7 and PvNLP9, was expressed strongest at the early stages of infection (up to 24 hpi) but subsided quickly afterward. However, the second group (PvNLP4, PvNLP5 and PvNLP10) genes were highly expressed at multiple time points in both early and late stages almost the entire infection process. Although previous study showed that PvNLP2 and PvNLP3 expression also increased during the first 6 h of the infection, the expression stayed elevated during the whole infection and both reached a maximum level at 72 hpiCitation44 which was different from our results. It may be resulted from the different isolates of P. viticola and grapevine varieties.
Figure 3. Transcriptional expression of 7 PvNLP genes from P. viticola isolate “ZJ-1-1” during the pathogen-grapevine infection.
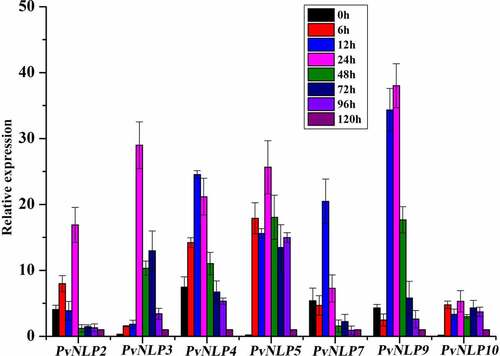
Cytotoxicity of PvNLPs in N. benthamiana leaves
Several NLPs from various microorganisms have been shown to be able to induce necrosis cell death preferentially in dicotyledonous plants. Nevertheless, the ability to induce necrosis of NLPs was not always consistent in many pathogens, such as the obligate biotroph Hyaloperonospora arabidopsidis. In order to investigate the PvNLP-induced cellular responses in N. benthamiana plants, we transiently expressed the seven different PvNLP coding sequences by using agroinfiltration, a system that has been previous validated to determine cytotoxic activity of NLP proteins.Citation45 INF1 from P. infestans, which is known to induce cell death in N. benthamiana, was served as positive controlCitation46 and GFPCitation35 and empty vector pGR106 were negative controls. showed that only PvNLP7 was able to induce necrosis in N. benthamiana leaves 8 days after infiltration, showing the similar symptom to that induced by INF1. The other six PvNLP proteins did not result in any cell death response as did the negative controls GFP and pGR106 ( and ), with no visible symptoms up to 2 weeks post-inoculation. Immunoblot analysis of PvNLPs showed that all PvNLP genes were expressed normally in N. benthamiana (). These results indicated that PvNLPs differed in cytotoxicity in N. benthamiana.
Figure 4. Comparative analysis of necrosis-inducing response observed for various PvNLP genes by PVX agroinfiltration in N. benthamiana leaves.
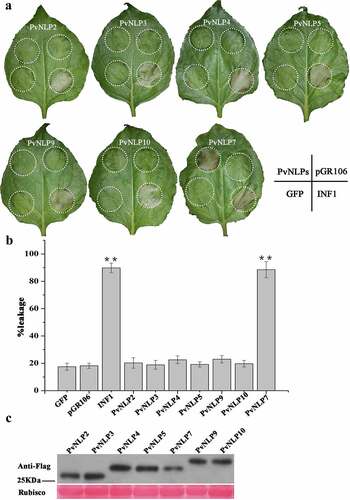
PvNLP induces disease resistance in N. benthamiana
NLP proteins, secreted from multiple pathogens, were proposed to have the function of triggering many characteristic immune responses in dicotyledonous plants.Citation27,Citation47,Citation48 To determine whether PvNLPs could trigger defense responses in plants, the leaves of N. benthamiana were agroinfiltrated with separately PvNLP, and GFP was used as a control. The Agrobacterium suspension was adjusted to a non-lethal concentration (OD600 = 0.2) which could not induce cell death in N. benthamiana at 3 d post-infiltration. The infiltrated regions were inoculated with P. capsici zoospores after 24 h of agroinfiltration. The disease resistance induced by transient PvNLPs expression was evaluated by calculating lesion diameter after 36 h of inoculation. The lesion diameter was significantly smaller in PvNLP7 than GFP-expressing leaves, whereas the remaining 6 PvNLP-expressing leaves showed similar levels of disease symptom with control ( and ). Therefore, PvNLP7 could induce P. capsici resistance in transiently transformed N. benthamiana.
Figure 5. Disease resistance induction in N. benthamiana by PvNLPs.
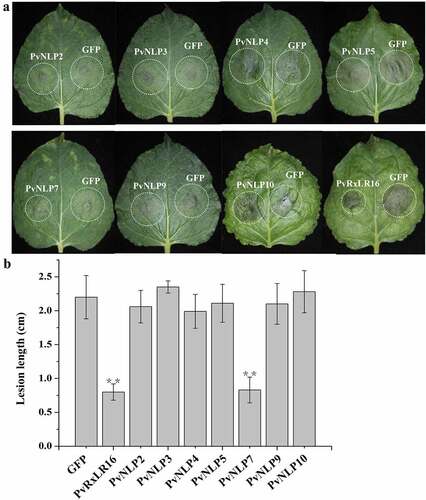
PvNLPs expression in arabidopsis leads to severe growth reduction and enhances plant resistance to downy mildew
The aforementioned experiments demonstrated PvNLP proteins have the abilities to improve N. benthamiana disease resistance. To verify whether PvNLPs enhance disease resistance in other dicotyledonous plants as in N. benthamiana, they were fused with the N terminus of flag under the control of the CaMV 35S promoter and stably expressed in Arabidopsis. All seven PvNLP genes transgenic lines were generated by hygromycin resistance selection and verified by DNA PCR and qRT-PCR detection. Surprisingly, ectopic expression of 4 of the 7 PvNLP genes (PvNLP4, PvNLP5, PvNLP7, and PvNLP10) resulted in transgenic Arabidopsis showing aberrant phenotypes such as dwarfed and severely reduced growth, compared with control plants transformed with empty vector (, b and c). Some severely abnormal transgenic plants even stopped growing with only two purple cotyledons, but some weakly aberrant plants did transition to flowering and produced seeds for homozygous screening (T3). However, the remain PvNLPs (PvNLP2, PvNLP3, and PvNLP9) did not show any significant differentiation in phenotypes in contrast with flag-expressing control. All PvNLPs-expressing lines growth was evaluated in the T3 generation by determining weight of the aerial parts of 20 seedlings per transgenic line. The PvNLP4-, PvNLP5-, PvNLP7-, and PvNLP10- expressing lines showed significant weight reduction compared with other PvNLPs and control plants, which confirmed the growth effects observed on individual T3 generation plants ().
Figure 6. PvNLPs expression in Arabidopsis leads to growth reduction and enhanced resistance to downy mildew.
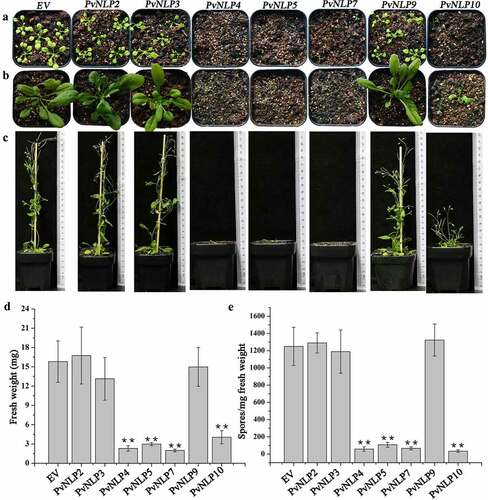
To investigate the role of PvNLPs in the process of resistance to the downy mildew H. arabidopsidis, we inoculated living plant seedlings with the pathogen and counted spore number per milligram of different transgenic lines. PvNLPs-expressing Arabidopsis plants showed enhanced resistance to the pathogen, and strikingly, these same transgenic lines also showed severe growth inhibition (). It seems that there was a strong correlation between the level of resistance and the fresh weight of different PvNLPs-expressing lines. In previous reports, there is a great many instances of plant growth suppression as a result of triggering plant immune response.Citation18,Citation49 The fact that the level of immunity of the transgenic lines expressing PvNLP genes is strongly correlated to their growth reduction, therefore, indicates that PvNLPs triggered immunity in Arabidopsis plants resulting in the observed growth reduction.
The NPP1 domain of PvNLP7 is essential for inducing cell death and disease resistance
It is well known that NLPs share a conserved domain referred as the NPP1 domain (also called nlp20 peptide) in diverse microbial pathogens.Citation14 In an attempt to investigate whether the NPP1 domain of PvNLP7, containing conserved region I and region II (shown in ), has effect on the cell death-inducing or immunity activity, deletion mutant PvNLP7ΔNPPCitation1 lacking NPP1 domain was tested in N. benthamiana. As expected, PvNLP7ΔNPPCitation1 could not induce cell death as wild type anymore ( and ). Likewise, the ability of enhancing disease resistance to P. capsici was abolished ( and ). The expression of PvNLP7 and truncated protein PvNLPΔNPPCitation1 were checked by Western blot (). This finding highlights the importance of this NPP1 domain for the cell death-activation and disease resistance activity of PvNLP7.
Figure 7. The NPP1 domain (a 27-aa peptide) of PvNLP7 is essential for inducing necrosis and disease resistance.
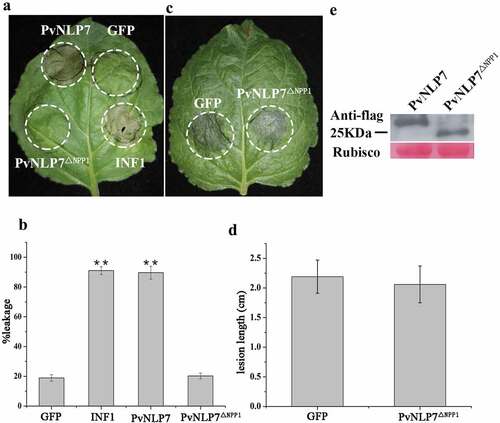
PvNLP7 enhances the expression of defense-associated genes
To increase our understanding of the role of PvNLPs in plant disease resistance, PvNLP7, the only one inducing cell death in N. benthamiana was chosen for further study. GFP and PvNLP9 which could not trigger immunity in N. benthamiana and Arabidopsis were selected as negative controls. The transcriptional levels of two defense-associated genes, PR1b and PR2b, were monitored in N. benthamiana transiently expressing PvNLP7 using real-time qRT-PCR. The PR1b and PR2b are both marker genes for salicylate-mediated signaling which play a vital role in defense resistance to pathogens.Citation50,Citation51 The relative expression levels of PR1b and PR2b induced by PvNLP7 began to increase significantly compared to controls at 48 h and 24 h, and then reached peaks at 96 h and 72 h, respectively (). Whereas the PvNLP9 and GFP slightly or even not induced the expression levels of PR1b and PR2b. These results revealed that PvNLP7 recognition by plants may induce the expression of defense-associated genes, resulting in disease resistance.
Figure 8. Upregulation of defense related genes mediated by PvNLP7 in N. benthamiana.
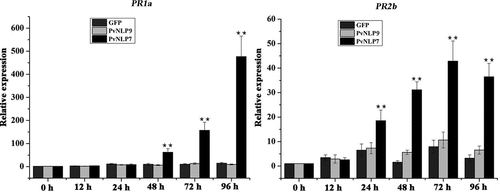
Discussion
In this report, we described the characterization of seven independent NLPs genes from the obligate biotrophic pathogen P. viticola. They were all cloned and classified as type 1 NLPs, like all other oomycete NLPs characterized so far.Citation9,Citation18 Five members of the NLP gene family in P. viticola have been identified elsewhere,Citation44 but only two (PvNLP2 and PvNLP3) were selected for functional analysis. Considering these new findings, the PvNLP family comprises a total of 10 genes and is more expanded in P. viticola than initially described.
It is well known that NLPs typically possess a signal peptide followed by a nlp24 peptide. PvNLP genes encode predicted secreted proteins that are likely targeted to the host apoplast during infection. Intriguingly, PvNLP2 and PvNLP3 were predicted to have no signal peptide (SP) and SP cleavage site based on SignalP HMM probability test. It is possible that the algorithms of online software used for prediction of SP are not accumulate or accurate for oomycete sequences. The secretory function of these two genes needs to be further confirmed by signal sequence trap assay (SST) in the future. The nlp24 peptide is strongly conserved in the type 1 of both cytotoxic and noncytotoxic NLPs, which can trigger plant immune responses10−11. All studied NLPs from P. viticola contain the nlp24 peptide with variable degeneracy, which of PvNLP9 has only a minimum of 16 amino acids (). Previous studies showed that the peptide is indispensable for necrosis-inducing activity10−11. We discovered that the nlp24 peptide of PvNLP7 is essential for phytotoxic activity and immune responses, which strengthen the importance of nlp24 peptide in the interaction between microorganisms and plants.
NLP genes from different pathogens expressed under certain conditions have been reported in several cases. In Erwinia carotovora, Nep was upregulated only when the bacteria were grown on solid medium.Citation52 In necrotrophic fungal pathogen Botrytis cinerea, BcNep1 and BcNep2 exhibit a significant upregulation at early and late stage of infection, respectively.Citation53 In P. sojae, the expression of most of NLP genes was generally higher at late infection phases.Citation21 In P. capsici, transcript levels of 6 of 11 expressed PcNLP genes gradually accumulated to a maximum at the late stage of infection, while the induction of remainder five PcNLP genes occurred at the early stages of infection.Citation54 In contrast, for all NLP genes with detectable expression in the obligate biotroph oomycete H. arabidopsis, relative high expression was detected at the early stages of infection.Citation19 We performed transcriptional profiling of seven PvNLP genes by RT-qPCR and found that all genes were expressed during very early stages of the infection. Therefore, it seems that most NLP genes are naturally involved in the interaction between the pathogens and their hosts, but the different expression patterns of NLP genes suggest their diversified roles during infection.
In this study, 6 of 7 identified PvNLP proteins are noncytotoxic which could not induce cell death in N. benthamiana. This is not surprising, since P. viticola is an obligate biotrophic pathogen that needs living host cells for completion of its life cycle. According to the expression patterns of PvNLP genes, it is tempting to speculate that these six nontoxic NLP genes play functional roles different from necrosis-inducing during the infection process and may function in development stages or primary contact between pathogen and host. It is intriguing that PvNLP7 have the ability of necrosis-inducing acting as a toxic protein though 10 out of 11 critical amino acids required for cytotoxicity are substituted in PvNLP718. It may be that too many mutated amino acids have caused the protein structure to change, forming a novel conformation. And it is possible that there is a new mechanism, different from previous studies, resulting in NLP cytotoxicity which needs more in-depth research to reveal in the future. Whereas the life cycle of P. viticola has only a biotrophic phase, it needs living host cells for colonization. It is very likely that the phytotoxic activity of PvNLP7 expressed during physiological infection is suppressed by other effectors like RxLR protein secreted by P. viticola.Citation18,Citation55 Some NLPs can act as microbe-associated molecular patterns (MAMPs) which can trigger MTI.Citation23 MTI is generally associated with pathogen resistance, activation of mitogen-activated protein (MAP) kinase activity, induction of ROS burst, deposition of callose, and increased expression levels of defense-related genes.Citation56 In this study, we observed that expression of PvNLP4, PvNLP5, PvNLP7, and PvNLP10 in A. thaliana enhanced resistance against H. arabidopsidis. And also, the PvNLP7 enhanced the expression level of defense-associated genes in N. benthamiana. It suggests that PvNLP4, PvNLP5, PvNLP7, and PvNLP10 may each play a role as MAMP triggering MTI in Arabidopsis. It is worth noting that PR1b and PR2b genes were activated by PvNLP7. It is plausible that PvNLP7 could elicit SA signaling pathway to restrict pathogen growth. SA is the classical immunity hormone which plays key roles in plant defenses against pathogens and is generally crucial for immunity against biotrophs or hemibiotrophs.Citation50 It will be very interesting to determine whether these four PvNLP proteins induced immunity can inhibit invasion of different pathogens to plants. NLPs often function as key virulence determinants of pathogens on plants due to the cytotoxic activity.Citation31,Citation57 Several NLP genes have been proved to be positive factors in virulence.Citation30,Citation32,Citation52 However, three NLPs from M. oryzae functioned as cytolytic toxins to induce necrotic cell death the production of reactive oxygen species, but they were all dispensable for the infection of rice plants.Citation26 Unfortunately, it is difficult to determine whether PvNLPs are crucial for the pathogenicity of P. viticola in grapevine infection because the pathogen can hardly be genetically modified due to its typical obligate lifestyle. In the further investigation, we should grope the genetic transformation system of P. viticola, so as to better dig out the pathogenic virulence factors of the special pathogen.
In summary, 7 NLP genes from P. viticola were characterized in detail. PvNLP4, PvNLP5, PvNLP7, and PvNLP10 could enhance plants resistance to pathogens, indicating they may act as MAMPs like HaNLP3. Specifically, PvNLP7 has the dual function of stimulating defense response and cytotoxic activity. In contrast, PvNLP2, PvNLP3, and PvNLP9 are noncytotoxic proteins and have no effect on plant disease resistance. Collectively, these data demonstrated that the NLPs of P. viticola show a functional diversification. Moreover, these immunity-inducing NLPs will be very useful to identify novel defense-related genes against P. viticola or other pathogens and to lay a foundation for further study on host-pathogen interactions.
Supplemental Material
Download MS Word (25.1 KB)Acknowledgments
The authors are grateful to Ling Yin (Guangxi Academy of Agricultural Sciences) for her technical assistance with sequence analysis, and to Pengbo Xu (Shanghai Jiao Tong University) for providing vectors.
Disclosure statement
No potential conflict of interest was reported by the author(s).
Supplementary material
Supplemental data for this article can be accessed on the publisher’s website
Additional information
Funding
References
- Dodds P, Rathjen J. Plant immunity: towards an integrated view of plant–pathogen interactions. Nat Rev Genet. 2010;11:539–12. doi:10.1038/nrg2812.
- Katagiri F, Tsuda K. Understanding the plant immune system. Mol Plant Microbe Interact. 2010;23(12):1531–1536. doi:10.1094/MPMI-04-10-0099.
- Thomma BPHJ, Nürnberger T, Joosten MHAJ. Of PAMPs and effectors: the blurred PTI-ETI dichotomy. Plant Cell. 2011;23(1):4–15. doi:10.1105/tpc.110.082602.
- Bigeard J, Colcombet J, Hirt H. Signaling mechanisms in pattern-triggered immunity (PTI). Mol Plant. 2015;8(4):521–539. doi:10.1016/j.molp.2014.12.022.
- Zipfel C. Plant pattern-recognition receptors. Trends Immunol. 2014;35(7):345–351. doi:10.1016/j.it.2014.05.004.
- Felix G, Duran JD, Volko S, Boller T. Plants have a sensitive perception system for the most conserved domain of bacterial flagellin. Plant Journal. 1999;18(3):265–276. doi:10.1046/j.1365-313X.1999.00265.x.
- Kunze G, Zipfel C, Robatzek S, Niehaus K, Boller T, Felix G. The N terminus of bacterial elongation factor Tu elicits innate immunity in Arabidopsis plants. Plant Cell. 2004;16(12):3496–3507. doi:10.1105/tpc.104.026765.
- Gust AA, Biswas R, Lenz HD, Rauhut T, Ranf S, Kemmerling B, Götz F, Glawischnig E, Lee J, Felix G, et al. Bacteria-derived peptidoglycans constitute pathogen-associated molecular patterns triggering innate immunity in arabidopsis. Journal Biol Chem. 2007;282(44):32338. doi:10.1074/jbc.M704886200.
- Gijzen M, Nürnberger T. Nep1-like proteins from plant pathogens: recruitment and diversification of the NPP1 domain across taxa. Phytochemistry. 2006;67(16):1800–1807. doi:10.1016/j.phytochem.2005.12.008.
- Oome S, Raaymakers TM, Cabral A, Samwel S, Böhm H, Albert I, Nürnberger T, Van Den Ackerveken G. Nep1-like proteins from three kingdoms of life act as a microbe-associated molecular pattern in Arabidopsis. Proc Natl Acad, U S A. 2014;111(47):16955–16960. doi:10.1073/pnas.1410031111.
- Böhm H, Albert I, Oome S, Raaymakers TM, Van Den Ackerveken G, Nürnberger T, Birch P. A conserved peptide pattern from a widespread microbial virulence factor triggers pattern-induced immunity in Arabidopsis. PLoS Pathog. 2014;10(11):e1004491. doi:10.1371/journal.ppat.1004491.
- Bailey BA. Purification of a protein from culture filtrates of Fusarium oxysporum that induces ethylene and necrosis in leaves of Erythroxylum coca. Phytopathology. 1995;85(10):1250–1255. doi:10.1094/Phyto-85-1250.
- Fellbrich G, Blume B, Brunner F, Hirt H, Kroj T, Ligterink W, Romanski A, Nurnberger T. Phytophthora parasitica elicitor-induced reactions in cells of petroselinum crispum. Plant Cell Physiol. 2000;41(6):692–701. doi:10.1093/pcp/41.6.692.
- Fellbrich G, Romanski A, Varet A, Blume B, Brunner F, Engelhardt S, Felix G, Kemmerling B, Krzymowska M, Nürnberger T. NPP1, a Phytophthora‐ associated trigger of plant defense in parsley and Arabidopsis. Plant J. 2002;32(3):375–390. doi:10.1046/j.1365-313X.2002.01454.x.
- Bae H, Kim MS, Sicher RC, Bae HJ, Bailey BA. Necrosis- and ethylene-inducing peptide from Fusarium oxysporum induces a complex cascade of transcripts associated with signal transduction and cell death in Arabidopsis. Plant Physiol. 2006;141(3):1056–1067. doi:10.1104/pp.106.076869.
- Kamoun SA. Catalogue of the effector secretome of plant pathogenic oomycetes. Annu Rev Phytopathol. 2006;44(1):41–60. doi:10.1146/annurev.phyto.44.070505.143436.
- Pemberton CL, Salmond GP. The Nep1-like proteins-a growing family of microbial elicitors of plant necrosis. Mol Plant Pathol. 2004;5(4):353–359. doi:10.1111/j.1364-3703.2004.00235.x.
- Oome S. Van den Ackerveken G. Comparative and functional analysis of the widely occurring family of Nep1-Like proteins. Mol Plant Microbe Interact. 2014;27(10):1081–1094. doi:10.1094/MPMI-04-14-0118-R.
- Cabral A, Oome S, Sander N, Küfner I, Nürnberger T, Van Den Ackerveken G. Nontoxic Nep1-like proteins of the downy mildew pathogen Hyaloperonospora arabidopsidis: repression of necrosis-inducing activity by a surface-exposed region. Mol Plant-Microbe Interact. 2012;25(5):697–708. doi:10.1094/MPMI-10-11-0269.
- Motteram J, Küfner I, Deller S, Brunner F, Hammond-Kosack KE, Nürnberger T, Rudd JJ. Molecular characterization and functional analysis of MgNLP, the sole NPP1 domain-containing protein, from the fungal wheat leaf pathogen Mycosphaerella graminicola. Mol Plant Microbe Interact. 2009;22(7):790–799. doi:10.1094/MPMI-22-7-0790.
- Dong S, Kong G, Qutob D. The NLP toxin family in Phytophthora sojae includes rapidly evolving groups that lack necrosis-inducing activity. Mol Plant Microbe Interact. 2012;25(7):896–909. doi:10.1094/MPMI-01-12-0023-R.
- Boller T, Felix G. A renaissance of elicitors: perception of microbe-associated molecular patterns and danger signals by pattern-recognition receptors. Annu Rev Plant Biol. 2009;60(1):379. doi:10.1146/annurev.arplant.57.032905.105346.
- Qutob D, Kemmerling B, Brunner F, Kufner I, Engelhardt S, Gust AA, Luberacki B, Seitz HU, Stahl D, Rauhut T. Phytotoxicity and innate immune responses induced by Nep1-like proteins. Plant Cell. 2006;18(12):3721–3744. doi:10.1105/tpc.106.044180.
- Staats M, van Baarlen P, Schouten A, van Kan JA. Functional analysis of NLP genes from Botrytis elliptica. Mol Plant Pathol. 2007;8(2):209–214. doi:10.1111/j.1364-3703.2007.00382.x.
- Küfner I, Ottmann C, Nürnberger T, Oecking C. Cytolytic toxins as triggers of plant immune response. Plant Signal Behav. 2009;4(10):977–979. doi:10.4161/psb.4.10.9669.
- Fang YL, Peng YL, Fan J. The Nep1-like protein family of Magnaporthe oryzae is dispensable for the infection of rice plants. Sci Rep. 2017;7(1):4372. doi:10.1038/s41598-017-04430-0.
- Zhou BJ, Jia PS, Gao F, Guo HS. Molecular characterization and functional analysis of a necrosis- and ethylene-inducing, protein-encoding gene family from Verticillium dahliae. Molecular Plant-microbe Interactions. 2012;25(7):964. doi:10.1094/MPMI-12-11-0319.
- Kanneganti TD, Huitema E, Cakir C, Kamoun S. Synergistic interactions of the plant cell death pathways induced by Phytophthora infestans Nep1-like protein PiNPP1.1 and INF1 elicitin. Molecular Plant-microbe Interactions. 2006;19(8):854–863. doi:10.1094/MPMI-19-0854.
- Qutob D, Kamoun S, Gijzen M. Expression of a Phytophthora sojae necrosis-inducing protein occurs during transition from biotrophy to necrotrophy. Plant Journal. 2002;32(3):361–373. doi:10.1046/j.1365-313X.2002.01439.x.
- Amsellem Z, Cohen BA, Gressel J. Engineering hypervirulence in a mycoherbicidal fungus for efficient weed control. Nat Biotechnol. 2002;20(10):1035–1039. doi:10.1038/nbt743.
- Ottmann C, Luberacki B, Kufner I, Koch W, Brunner F, Weyand M, Mattinen L, Pirhonen M, Anderluh G, Seitz HU. A common toxin fold mediates microbial attack and plant defense. Proceedings of the National Academy of Sciences. 2009;106(25):10359–10364. doi:10.1073/pnas.0902362106.
- Pemberton CL, Whitehead NA, Sebaihia M, Bell KS, Hyman LJ, Harris SJ, Matlin AJ, Robson ND, Birch PRJ, Carr JP. Novel quorum-sensing-controlled genes in Erwinia carotovora subsp. carotovora: identification of a fungal elicitor homologue in a soft-rotting bacterium. Molecular Plant-microbe Interactions. 2005;18(4):343–353. doi:10.1094/MPMI-18-0343.
- Yin L, Li XL, Xiang J, Qu JJ, Zhang YL, Dry IB, Lu J. Characterization of the secretome of Plasmopara viticola by de novo transcriptome analysis. Physiol Mol Plant Pathol. 2015;91:1–10. doi:10.1016/j.pmpp.2015.05.002.
- Yin L, An YH, Qu JJ, Li XL, Zhang YL, Dry IB, Wu H, Lu J. Genome sequence of Plasmopara viticola and insight into the pathogenic mechanism. Sci Rep. 2017;7:1–12.
- Xiang J, Li XL, Wu J, Yin L, Zhang YL, Lu J. Studying the mechanism of Plasmopara viticola RxLR effectors on suppressing plant immunity. Front Microbiol. 2016;7:709. doi:10.3389/fmicb.2016.00709.
- Lan X, Liu Y, Song S, Yin L, Xiang J, Qu J, Lu J. Plasmopara viticola effector PvRXLR131 suppresses plant immunity by targeting plant receptor-like kinase inhibitor BKI1. Mol Plant Pathol. 2019;20(6):765–783. doi:10.1111/mpp.12790.
- Livak KJ, Schmittgen TD. Analysis of relative gene expression data using real-time quantitative PCR and the 2− ΔΔCT method. Methods. 2001;25(4):402–408. doi:10.1006/meth.2001.1262.
- Saitou N, Nei M. The neighbor-joining method: a new method for reconstructing phylogenetic trees. Mol Biol Evol. 1987;4:406–425.
- Hellens RP, Edwards EA, Leyland NR, Bean S, Mullineaux PM. pGreen: a versatile and flexible binary Ti vector for Agrobacterium-mediated plant transformation. Plant Mol Biol. 2000;42(6):819–832. doi:10.1023/A:1006496308160.
- Zhang M, Meng Y, Wang Q, Liu D, Quan J, Hardham AR, Shan W. PnPMA1, an atypical plasma membrane H+-ATPase, is required for zoospore development in Phytophthora parasitica. Fungal Biol. 2012;116(9):1013–1023. doi:10.1016/j.funbio.2012.07.006.
- Xiang J, Li XL, Yin L, Liu YX, Zhang YL, Qu JJ, Lu J. A candidate RxLR effector from Plasmopara viticola can elicit immune responses in Nicotiana benthamiana. BMC Plant Biol. 2017;17(1):1–14. doi:10.1186/s12870-017-1016-4.
- Yu X, Tang J, Wang Q, Ye W, Tao K, Duan S, Lu C, Yang X, Dong S, Zheng X, et al. The RxLR effector Avh241 from Phytophthora sojae requires plasma membrane localization to induce plant cell death. New Phytol. 2012;196(1):247–260. doi:10.1111/j.1469-8137.2012.04241.x.
- Clough SJ, Bent AF. Floral dip: a simplified method for Agrobacterium‐mediated transformation of Arabidopsis thaliana. Plant J. 1998;16(6):735–743. doi:10.1046/j.1365-313x.1998.00343.x.
- Schumacher S, Grosser K, Voegele RT, Kassemeyer HH, Fuchs R. Identification and characterization of Nep1-like proteins from the grapevine downy mildew pathogen Plasmopara viticola. Front Plant Sci. 2020;11:65. doi:10.3389/fpls.2020.00065.
- Schouten A, Van Baarlen P, Van Kan JA. Phytotoxic Nep1‐like proteins from the necrotrophic fungus Botrytis cinerea associate with membranes and the nucleus of plant cells. New Phytol. 2008;177(2):493–505. doi:10.1111/j.1469-8137.2007.02274.x.
- Kamoun S, Van West P, Vleeshouwers VG, De Groot KE, Govers F. Resistance of Nicotiana benthamiana to Phytophthora infestans is mediated by the recognition of the elicitor protein INF1. Plant Cell. 1998;10(9):1413–1425. doi:10.1105/tpc.10.9.1413.
- Villela‐Dias C, Camillo LR, de Oliveira GA, Sena JAL, Santiago AS, de Sousa STP, Mendes JS, Pirovani CP, Alvim FC, Costa MGC. Nep1-like protein from Moniliophthora perniciosa induces a rapid proteome and metabolome reprogramming in cells of Nicotiana benthamiana. Physiol Plant. 2014;150(1):1–17. doi:10.1111/ppl.12061.
- Teh CY, Pang CL, Tor XY, Ho PY, Lim YY, Namasivayam P, Ho CL. Molecular cloning and functional analysis of a necrosis and ethylene inducing protein (NEP) from Ganoderma boninense. Physiol Mol Plant Pathol. 2019;106:42–48. doi:10.1016/j.pmpp.2018.12.003.
- Bolton MD. Primary metabolism and plant defense—fuel for the fire. Molecular Plant-microbe Interactions. 2009;22(5):487–497. doi:10.1094/MPMI-22-5-0487.
- Glazebrook J. Contrasting mechanisms of defense against biotrophic and necrotrophic pathogens. Annu Rev Phytopathol. 2005;43:205–227. doi:10.1146/annurev.phyto.43.040204.135923.
- Lee S, Ishiga Y, Clermont K, Mysore KS. Coronatine inhibits stomatal closure and delays hypersensitive response cell death induced by nonhost bacterial pathogens. PeerJ 2013;1:e34. doi:10.7717/peerj.34.
- Mattinen L, Tshuikina M, Mae A, Pirhonen M. Identification and characterization of Nip, necrosis-inducing virulence protein of Erwinia carotovora subsp. carotovora. Mol Plant Microbe Interact. 2004;17(12):1366–1375. doi:10.1094/MPMI.2004.17.12.1366.
- Arenas YC, Kalkman ERIC, Schouten A, Dieho M, Vredenbregt P, Uwumukiza B, Ruiz MO, van Kan JAL. Functional analysis and mode of action of phytotoxic Nep1-like proteins of Botrytis cinerea. Physiol Mol Plant Pathol. 2010;74(5–6):376–386. doi:10.1016/j.pmpp.2010.06.003.
- Feng BZ, Zhu XP, Fu L, Lv RF, Storey D, Tooley P, Zhang XG. Characterization of necrosis-inducing NLP proteins in Phytophthora capsici. BMC Plant Biol. 2004;14:1–19.
- Chen XR, Xing YP, Li YP, Tong YH, Xu JY. RNA-Seq reveals infection-related gene expression changes in Phytophthora capsici. PLoS One. 2013;8(9):e74588. doi:10.1371/journal.pone.0074588.
- Newman MA, Sundelin T, Nielsen JT, Erbs G. MAMP (microbe-associated molecular pattern) triggered immunity in plants. Front Plant Sci. 2013;4:139. doi:10.3389/fpls.2013.00139.
- Baroncelli R, Amby DB, Zapparata A, Sarrocco S, Vannacci G, Le Floch G, Harrison RJ, Holub E, Sukno SA, Sreenivasaprasad S. Gene family expansions and contractions are associated with host range in plant pathogens of the genus Colletotrichum. BMC Genomics. 2016;17(1):555. doi:10.1186/s12864-016-2917-6.