ABSTRACT
Over 40% of arable land in the world is acidic. Al stress has become a global agricultural problem affecting plant growth and limiting crop production in acidic soils. Plants have evolved different regulatory mechanisms of adaptation to exogenous environmental challenges, such as Al stress, by altering their growth patterns. In the past decades, several key genes involved in plant response to Al stress and the mechanism of Al detoxification have been revealed. However, the signaling pathways of plant response to Al stress and the regulatory mechanism of plant Al tolerance remain poorly understood. In this review, we summarized the findings of recent studies on the plant Al tolerance mechanism and the molecular regulation mechanism of phytohormones in response to Al stress. This review improves our understanding of the regulatory mechanisms of plants in response to Al stress and provides a reference for the breeding of Al-tolerant crops.
1. Introduction
As the most abundant metal element on earth, Al is widespread in the environment. It is an amphoteric metal with active chemical properties; apart from reacting with strong bases, it also dissolves in acidic solutions. In the soil, Al normally exists in the form of insoluble oxides or aluminosilicates, and in this chemical form, it exerts no toxic effects on plants.Citation1 When the soil pH is below 5.5, Al and aluminide become increasingly soluble and form Al3+, Al(OH)2+, or Al(OH)2+.Citation2–4 Solubilized Al, especially Al3+, is highly toxic to the root apex and severely restricts the ability of the root system to absorb water and nutrients in acidic soils.Citation5,Citation6 Although Al is not considered a nutrient element, previous studies have shown that low concentrations of Al3+ can promote plant growth.Citation7,Citation8 When the concentration of Al3+ in the soil reaches micromolar levels, plant growth and development are negatively affected and show symptoms of Al toxicity.Citation9,Citation10
Currently, approximately 40% of arable land in the world is acidic.Citation11 With the increase in acid gases emitted by different industries as well as the increase in acid rain pollution, the threat of Al stress to plant growth and human food security is further exacerbated. Consequently, Al stress has emerged as crucial environmental issue for plants, second only to drought stress.Citation11 Al causes toxicity and irreversible damage to the growth of plants, consequently affecting crop yield and quality.Citation12,Citation13 Therefore, exploring the molecular mechanisms of Al tolerance in plants is essential to improving agricultural practices, as there will be a continual demand for plants that can cope with environmental changes, as well as for increasing the production and supply of safe food.
2. Mechanisms of Al toxicity and adaptive response in plants
2.1 Al toxicity in plants at the cellular level
Roots are the main plant parts exposed to Al stress, and primary root elongation and vitality are severely inhibited by Al3+.Citation14–18 When plants are exposed to Al stress, the most obvious symptom is the inhibition of root elongation, following which the acquisition of water and nutrients becomes limited.Citation8,Citation19,Citation20 Consequently, plants fail to obtain sufficient nutrients and begain to manifest symptoms of nutritional deficiencies.Citation5,Citation14 Moreover, Al stress can cause programmed cell death and leaf yellowing, which leads to early plant senescence.Citation21,Citation22 Therefore, Al stress is critical factor affecting plant growth and limiting crop yield in areas with acidic soil.Citation23
As mentioned above, the toxic effect of Al on plants mainly occurs through the inhibition of root elongation, which further affects plant growth and development.Citation24,Citation25 For this reason, researchers usually use roots as experimental materials to explore the response mechanisms of plants to Al stress.Citation25,Citation26 Previous studies have shown that Al3+ mainly affects root growth by inhibiting cell elongation and division.Citation17,Citation27,Citation28 The root tip is considered the primary target site for Al3+, and the transition zone between the root tip meristem and the elongation zone is the root area most sensitive to Al stress.Citation8,Citation29–31 Therefore, the root tip region should be the focus of research on Al stress resistance mechanisms.
The toxicity of Al to plants is primarily caused by its influence on cell structure and cell life activities, especially cell wall structure and cell division.Citation17,Citation27,Citation28,Citation32 The cell wall serves as the first natural barrier for plants to resist harmful environments, and is vital for plant defense.Citation33 It is rich in carboxyl and phosphate groups which carry a substantial amount of negative charge.Citation34 Al3+ binds to the cell wall through cation exchange to prevent itself from binding to the plasma membrane or entering the symplasm.Citation34,Citation35 The amount of Al3+ binding to the cell wall is directly correlated with the damage to the plant.Citation33,Citation36,Citation37 Studies have shown that Al3+ thickens the cell wall and changes its composition, hindering cell division and elongation, consequently inhibiting root elongation.Citation17,Citation28,Citation38,Citation39
Reactive oxygen species (ROS) are important signaling molecules in plant stress response.Citation40,Citation41 Oxidative stress is an integral aspect of the toxic effects of Al on plants.Citation30,Citation42,Citation43 Al exposure causes the increase in ROS and leads to lipid peroxidation, resulting in cell organelles dysfunction and damage.Citation30,Citation42–46 The over-accumulation of ROS is induced by Al stress, which leads to peroxidative damage to the plasma membrane and destroys cell membrane integrity.Citation34,Citation47–50 Further studies showed that ROS accumulation and related cell dysfunction are also involved in Al-induced inhibition of cell elongation and division.Citation47 Impairment of cellular function and DNA damage are major factors responsible for inhibiting root elongation.Citation12,Citation51–53 Previous research showed that the scavenging of ROS contributes to plant Al.Citation43 Therefore, strategies to improve the scavenging ability of ROS and reduce the production of ROS will be a lucrative research direction to enhance plant tolerance to Al stress.
Previous studies have shown that Al also affects cell-membranes function and numerous physiological processes.Citation19,Citation27,Citation54 Al3+ is a blocker of various cation channels on the cell membrane. It therefore affects the absorption of mineral elements by changing plasma membrane fluidity and structure, further interfering with the normal physiological process of plant cells.Citation19,Citation27,Citation55 Al3+ competitively binds to Ca2+ receptors on the plasma membrane, inhibits Ca2+ transmembrane transport, and disrupts cytosolic Ca2+ homeostasis.Citation54,Citation56 In addition, Al3+ inhibits the absorption of K+ by the root system and reduces the plant potassium content, causing symptoms of K+ deficiency.Citation57 Active transport of numerous ions is driven by H+ gradient established by proton pumps, such as vacuolar H+-pyrophosphatase (V-PPase), vacuolar H+-ATPase (V-ATPase), and plasma membrane (PM) H+-translocating adenosine triphosphatase (PM H+-ATPase).Citation18,Citation58,Citation59 Al toxicity not only destroys the structure and physiological activities of plant cells, but also affects the metabolism and life processes of cells. However, further studies are required to reveal the mechanisms of Al toxicity.
2.2 Adaptive mechanisms of plant tolerance to Al toxicity
During their long-term evolution, plants have developed a variety of adaptation strategies to cope with Al toxicity, among which internal tolerance and external exclusion are widely considered to be the main strategies.Citation5,Citation14,Citation18 The exclusion mechanism includes secreting organic acids (OAs) or phosphoric acid into the apoplastic space to chelate external Al.Citation13,Citation18,Citation60 In addition to the chelation, the cell wall is considered another natural barrier for Al.Citation61,Citation62 On the other hand, the internal tolerance mechanisms involve the chelation of Al3+ by OAs in the cytosol, its transport, and the storage of its complexes into vacuoles.Citation13,Citation18
The cell wall is the plant’s first barrier against harmful external environments. Studies have shown that most of the Al3+ absorbed by plants is distributed in the cell wall.Citation63–65 Cell wall polysaccharides, especially pectin, carry numerous carboxyl groups and demonstrate a strong affinity for Al3+.Citation5,Citation27,Citation66 Extracellular Al3+ ions can bind directly to the cell wall.Citation61 Xyloglucan is an important structural component of cell walls. XTHs, encoded xyloglucan endotransglucosylase-hydrolase, are involved in cell wall extension.Citation61 XTH31 modulates Al binding capacity by regulating the content of xyloglucan in the cell wall, thereby affecting plant sensitivity to Al toxicity.Citation61 The fixation of Al on cell wall results in the sensitivity of root growth to Al toxicity.Citation12 The components and structure of the cell wall are altered by Al toxicity. Excessive Al binding to the cell wall leads to the disruption of cell wall extension, thereby inhibiting cell and root elongation.Citation61 Multiple genes related to cell wall synthesis or modification are involved in plant response to Al toxicity.Citation61,Citation67,Citation68 WAK1 (cell wall-associated receptor kinase 1) co-localizes with pectin, which is critical for Al bindingCitation69 GRP3, a glycine-rich protein (GRP), is involved in plant response to Al stress by interacting with AtWAK1.Citation70 WAK1 overexpression or a mutation in GPR3 results in enhanced plant Al tolerance.Citation69,Citation70 However, the molecular mechanism underlying cell wall involvement in regulating plant tolerance to Al stress remains to be elucidated.
Furthermore, studies have shown that pectin methylation in the cell wall is related to the ability of plants to resist Al3+ absorption.Citation28,Citation33,Citation71 PME is a gene encoding pectin methylesterase in plants, and its expression level in Al-tolerant plants is significantly lower than that in Al-sensitive plants.Citation71 In maize, exogenous application of pectin methylesterase resulted in the accumulation of Al3+ in the roots and inhibited root growth.Citation28,Citation72 The higher the methylation level, the lower the cation exchange capacity in the cell wall. This, in turn, reduces the amount of Al3+ bound to pectin, resulting in a decrease in the damage caused by Al stress.Citation28,Citation71,Citation72 In addition, Al3+ destroys the plasma membrane structure and transmembrane ion channels by binding to plasma membrane phospholipids. This inhibits the transmembrane transport of certain ions and interferes with the ion balance in the cell, consequently affecting intracellular physiological functions.Citation27
In plant roots, Al toxicity induces the secretion of chelating agents such as OAs and phosphoric acid.Citation18,Citation73 These substances chelate with Al3+ around the plant roots to form macromolecule chelates, thereby hampering the entry of Al3+ into cells and ameliorating the toxic effects of Al on plants.Citation18 Plants mainly transport OAs such as malic acid and citric acid, to the cell exterior through a transport carrier on the plasma membrane to chelate Al3+ around the rhizosphere.Citation73 The transmembrane transport of OAs is driven by the proton pump on the plasma membrane (PM H+-ATPase).Citation18,Citation74,Citation75 The activity of the proton pump is significantly enhanced under Al stress, which promotes the efflux of malic and citric acids.Citation18 In plants, MATE encodes a citric acid transporter, and ALMT encodes a malic acid transporter.Citation73,Citation76–79 Al stress significantly increases the expression of MATE and ALMT, promotes the secretion of citric and malic acids, and chelates Al3+ in the rhizosphere, thereby reducing Al toxicity stress.Citation73,Citation80–84 TaALMT1, the first Al-tolerance gene, was identified from wheat.Citation85 Although TaALMT1 is functionally active without Al stress, its transport capability can be improved further by Al.Citation85–87 In addition, ALMT1 from other species has also been shown to improve Al tolerance in plants.Citation75,Citation88,Citation89 Different from ALMT, MATE exhibits two distinct physiological functions in plants. Certain members of the MATE family facilitate Fe translocation while others are involved in external Al detoxification.Citation73,Citation90–92 The vacuole is the principal storage site for OAs. Malic and citric acids synthesized in the cytoplasm are transported to the vacuole, which enhances the plant’s internal tolerance to Al toxicity.Citation18 In addition, plants express the ABC transporter family gene ALS3 to promote the transport of Al3+ in the roots and reduce the distribution of Al3+ in Al-sensitive cells or tissues, thereby mitigating the effects of Al toxicity on plants.Citation93
Previous studies showed that STOP1, a C2H2-type transcription factor, plays an important role in plant Al resistance;Citation80,Citation86 it enhances plant tolerance to Al by regulating the expression of Al-resistance genes, including ALS3, MATE, and ALMT1 ().Citation6,Citation73,Citation80,Citation86 Al stress promotes the accumulation of STOP1 in cells.Citation6 STOP1 directly acts on the promoter region of RAE1 to augment the expression of RAE1, and RAE1 interacts with the STOP1 protein through the ubiquitinated 26S protease pathway to promote the degradation of STOP1.Citation6 Therefore, a feedback regulation loop is formed between RAE1 and STOP1 ().Citation6
Figure 1. Proposed model for the regulation of malate and citrate secretion by STOP1 in response to Al stress and the proposed signaling pathway of Al-activated root malate and citrate exudation based on recent research on Arabidopsis.Citation6,Citation18,Citation73,Citation94,Citation95 In response to Al stress, Al3+ signals can be perceived by the plant and trigger the accumulation of STOP1 in the cell. As a transcription factor, STOP1 upregulates the expression of RAE1, ALMT1, and MATE. RAE1 reduces the amount of STOP1 by promoting the ubiquitination (Ub) and degradation of STOP1.Citation6,Citation18,Citation95 Al-activated excretion of malate and citrate occurs through the PM localized transporters of ALMT1 and MATE, respectively. The secretion of OAs plays a critical role in plant Al tolerance through the chelation of external Al.
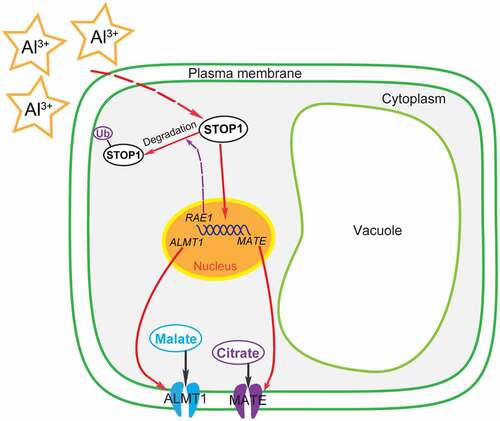
The secretion of malate and citrate by root cells plays a crucial role in plant Al tolerance and contribute to its detoxification.Citation73 Al-activated malate and citrate exudation were found to be affected by the loss of the STOP1 function, and the stop1 mutant showed increased sensitivity to Al.Citation73,Citation80 To cope with Al toxicity, plants secrete OAs to chelate external Al and/or regulate the expression of related genes responsible for plant Al-resistance ().Citation6,Citation18,Citation25,Citation73,Citation77,Citation78,Citation85,Citation90,Citation93–105 Although plants have different regulatory mechanisms and adaptive strategies to cope with Al toxicity, the molecular mechanisms underlying these strategies remain largely unclear.
Table 1. Related genes responsible for Al-activated secretion of OAs and plant Al-resistance
Previous studies have shown that the ability of plants to tolerate Al stress is related to rhizosphere pH.Citation3 Al solubility increases under acidic conditions, whereas it decreases significantly in a weakly alkaline environment. In addition to the chelation of Al3+ by OAs secretion, the solubility of Al can also be decreased by maintaining a higher pH of the rhizosphere and reducing the entry of Al3+ into the plant. This strategy is considered an effective method to enhance plant Al resistance. Therefore, a higher pH environment is beneficial for enhancing plant Al tolerance and alleviating its toxicity.Citation106,Citation107
2.3 Hormone signaling in plant Al stress response: ethylene and auxin as the key factors
Phytohormones play key roles in plant growth regulation in response to Al stress ().Citation8,Citation19,Citation20,Citation25,Citation108–112 Al stress upregulates TAA1 and YUCs (YUC3/5/7/8/9) in the roots, which promotes a localized increase in auxin synthesis and causes root growth inhibition.Citation8,Citation20 Ethylene has been reported to regulate plant Al tolerance through crosstalk with auxin signaling.Citation19,Citation20 Al exposure upregulates the expression of ethylene biosynthesis-related genes, such as ACSs and ACOs, thereby promoting ethylene synthesis.Citation19 As a signaling molecule, ethylene activates specific expression of the transcription factors EIN3 and EIL1 in the root apex transition zone.Citation20 EIN3 directly binds to the promoters of YUC9 and activates its expression.Citation20 The expression of PIF4 is also regulated by EIN3 and EIL1; moreover, the bHLH transcription factor PIF4 affects auxin biosynthesis and signaling by directly regulating the expression of YUC5, YUC8, and YUC9.Citation20 In addition, ethylene also upregulates the expression of TAA1 and promotes the local biosynthesis of auxin in the root apex transition zone to enhance the inhibition of root growth.Citation8 Therefore, the accumulation of auxin induced by Al stress is regulated by ethylene signaling.Citation19
Table 2. Hormone signaling-related genes in plant response to Al stress
Recent studies have shown that the polar transport of auxin is also involved in plant response to Al stress.Citation109 Ethylene production was found to be induced by Al3+, which acts as a signal to disrupt polar auxin transport by upregulating the expression of AUX1 and PIN2 auxin transporters, leading to auxin accumulation in the roots and inhibiting root growth.Citation19 Overexpression of the auxin efflux carrier OsPIN2 can alleviate Al-induced damage to the roots, which is a consequence of the decrease in extracellular Al3+ binding to the cell walls and reduced Al-targeted peroxidative cellular damage.Citation109 In maize, the auxin efflux carrier ZmPGP1 is involved in regulating auxin distribution in the root response to Al stress. ZmPGP1 expression was induced by Al treatment, but the accumulation of auxin was reduced in root tips.Citation25
Although auxin plays an important role in plant response to Al stress, its regulation mechanism varies completely among different plant species.Citation8,Citation25 In Arabidopsis, Al stress induces the biosynthesis and accumulation of auxin in the root apex transition zone, and excessive auxin inhibits root growth.Citation8 However, in maize, Al stress reduces auxin accumulation and inhibits root growth.Citation25 These two distinct actions of auxin imply that the auxin regulation mechanisms differ among plant species; however, their molecular background remains unclear.Citation8,Citation20,Citation25
TIR1/AFB-mediated auxin signaling pathways play vital roles in regulating root elongation.Citation113,Citation114 However, there are conflicting experimental results concerning TIR1/AFB signaling in root growth under Al stress.Citation4,Citation8,Citation115 Previous studies have shown that tir1-1 and tir1-1;afb2-1;afb3-1 mutants and wild type (WT) did not differ significantly in Al-induced root growth inhibition.Citation4 However, Yang et al. (2014) found that the Al stress-induced auxin signals were significantly decreased by PEO-IAA (specific antagonists to block TIR1/AFB signaling) treatment.Citation8 Consistently, TIR1/AFB is involved in the regulation of barley root growth inhibition under Al stress.Citation115 These contradictory experimental results might be due to the different pH values of the AlCl3 solutions used in different experiments. The pH 7.0 was used in the former experimentCitation4 while pH 4.3 and 5.0 was used in the other two experiments,Citation8,Citation115 respectively. Al3+ is mainly formed at pH≤5.0, whereas at pH 7.0 Al(OH)3 is predominant.Citation4 Therefore, different forms of aluminum present lead to distinct results. Based on these results, it is inferred that Al-induced inhibition of root growth is regulated by TIR1/AFB-mediated auxin signaling pathways ().Citation87,Citation108,Citation113,Citation115 Moreover, whether the known signaling pathway of TIR1/AFB-mediated apoplast alkalization is also involved in Al-induced root growth inhibition requires further study ().
Figure 2. Schematic representation of ethylene- and auxin-mediated regulation of root growth inhibition in response to Al stress. The proposed hormone signaling pathway under Al stress was based on recent research on plants.Citation8,Citation19,Citation20,Citation87,Citation108,Citation110,Citation111,Citation114,Citation115 The root tip is considered the main site that identifies Al toxicity. The transition zone (TZ) between the meristem and the elongation zone of the root apex is the most sensitive area for plants to perceive Al stress. Al stress induces auxin response in the root TZ, which is dependent on the ethylene signaling pathway. Al3+ was found to upregulate the expression of ACSs and ACOs and promote ethylene biosynthesis.Citation19 Ethylene promotes local auxin accumulation through TAA1- and YUCs-mediated local auxin biosynthesis.Citation8,Citation20,Citation110 In addition, ethylene promotes local auxin accumulation through AUX1- and PIN2-mediated polar auxin transport, resulting in root growth inhibition.Citation19,Citation108 ARF-mediated auxin signaling controls the Al-induced inhibition of root growth by regulating IPT-dependent cytokinin biosynthesis and cell wall modification-related genes.Citation8,Citation110,Citation111
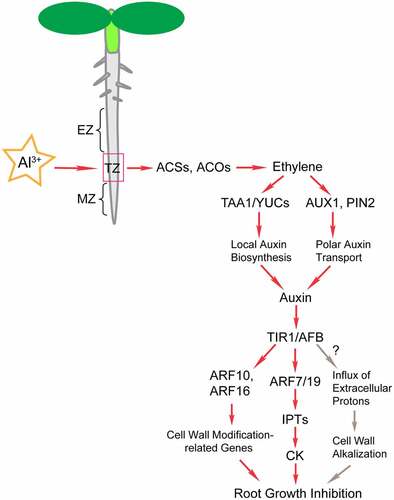
As transcription factors, auxin response factors (ARFs) are involved in auxin signaling downstream of TIR1/AFB. The auxin-regulated root growth inhibition induced by Al stress is mainly mediated by ARFs, which activates the expression of auxin response genes.Citation8,Citation20,Citation111 ARF7 promotes cytokinin biosynthesis by upregulating the expression of IPT5 and IPT7, whereas ARF10 and ARF16 are involved in Al-induced inhibition of root growth by regulating the expression of cell wall modification-related genes.Citation8,Citation111
In summary, Al-induced ethylene production is involved in auxin signaling to control root elongation under Al stress (). Although studies have shown that exogenous application of auxin can increase the expression of ALMT1, malate exudation was not affected by its application.Citation116 The cumulative evidence indicates that auxin and OAs exudation independently regulate the Al-induced inhibition of root growth.Citation8
3. Conclusions
Al stress is a major constraint for plant growth and crop yield in acidic soils. Therefore, over the past decade, studies aimed at elucidating the physiological and molecular mechanisms underlying plant tolerance to Al toxicity have attracted intense research interest. To cope with Al toxicity, many plant species have evolved various mechanisms to survive in unfavorable environments. There are two adaptive mechanisms that enable plants to withstand Al stress in acidic soils: external Al exclusion and internal Al tolerance.Citation14,Citation73 The mechanism underlying internal Al tolerance involves Al fixation in the cell wall, Al chelation by OAs in the cytosol, or Al sequestration into the vacuole. The exclusion mechanism involves the secretion of OAs from plant roots for Al3+ chelation. Although the responses of different plant species to Al share the same or similar regulatory mechanisms, there are still slight differences among different plant species, which depend on the signaling pathway activated by Al. Further research will help reveal species-specific mechanisms of plant Al tolerance. The Al tolerance phenotypes are the result of both environmental and genetic factors. In agricultural practices, two methods are used to overcome the threat of Al toxicity and improve plant tolerance to Al stress. Furthermore, the low pH values of acidic soils can be improved by applying alkaline substances such as CaO or Ca(OH)2; however, this requires considerable manpower and material resources. This issue should instead be tackled by planting Al-tolerant species or by improving cultivars through molecular-assisted plant breeding.
From the perspective of coping with changes in environmental conditions, breeding Al-tolerant and Al-insensitive plant species is the most effective and economical way to improve their ability to cope with Al stress. Exploring the response mechanism of different plant species to Al stress will help us understand the different pathways of Al tolerance. Using transcriptome analysis and genetic engineering technology to identify genes related to Al stress and improve plant Al tolerance via transgenic technology will be one of the most effective methods for breeding Al-tolerant plants.
Abbreviations
Al: Aluminum; ROS: Reactive oxygen species; V-PPase: Vacuolar H+-pyrophosphatase; V-ATPase: Vacuolar H+-ATPase; PM: Plasma membrane; H+-ATPase: H+-translocating adenosine triphosphatase; OAs: Organic acids.
Notes on contributions
HBL and CLW designed the study. HBL and RZ drafted the manuscript. KS, SW, and WXL revised the manuscript. All authors have read and approved the submitted version of the manuscript.
Acknowledgments
Thanks to all the peer reviewers for their opinions and helpful suggestions.
Disclosure statement
No potential conflict of interest was reported by the author(s).
Additional information
Funding
References
- May HM, Nordstrom DK. Assessing the solubilities and reaction kinetics of aluminous minerals in soils. In: Ulrich B, Sumner M.E, editors. Soil Acidity eds . Springer Berlin: Heidelberg; 1991. p. 125–11. doi:10.1007/978-3-642-74442-6.
- Kinraide TB, Parker DR. Assessing the phytotoxicity of mononuclear hydroxyaluminum. Plant Cell Environ. 1989;12(5):479–487. doi:10.1111/j.1365-3040.1989.tb02120.x.
- Kinraide TB. Identity of the rhizotoxic aluminum species. Plant Soil. 1991;134(1):167–178. doi:10.1007/BF00010729.
- Ruíz-Herrera LF, López-Bucio J. Aluminum induces low phosphate adaptive responses and modulates primary and lateral root growth by differentially affecting auxin signaling in Arabidopsis seedlings. Plant Soil. 2013;371(1–2):593–609. doi:10.1007/s11104-013-1722-0.
- Kochian LV. Cellular mechanisms of aluminum toxicity and resistance in plants. Annu Rev Plant Physiol Plant Mol Biol. 1995;46(1):237–260. doi:10.1146/annurev.pp.46.060195.001321.
- Zhang Y, Zhang J, Guo J, Zhou F, Singh S, Xu X, Xie Q, Yang Z, Huang CF. F-box protein RAE1 regulates the stability of the aluminum-resistance transcription factor STOP1 in Arabidopsis. Proc Natl Acad Sci U S A. 2019;116(1):319–327. doi:10.1073/pnas.1814426116.
- Ghanati F, Morita A, Yokota H. Effects of aluminum on the growth of tea plant and activation of antioxidant system. Plant Soil. 2005;276(1–2):133–141. doi:10.1007/s11104-005-3697-y.
- Yang ZB, Geng X, He C, Zhang F, Wang R, Horst WJ, Ding Z. TAA1-Regulated Local Auxin Biosynthesis in the Root-Apex Transition Zone Mediates the Aluminum-Induced Inhibition of Root Growth in Arabidopsis. Plant Cell. 2014;26(7):2889–2904. doi:10.1105/tpc.114.127993.
- Foy CD, Charnay RL, White MC. The physiology of metal toxicity in plants. Annu Rev Plant Physiol. 1978;29(1):511–566. doi:10.1146/annurev.pp.29.060178.002455.
- Delhaize E, Ryan PR. Aluminum toxicity and tolerance in plants. Plant Physiol. 1995;107(2):315–321. doi:10.1104/pp.107.2.315.
- Von Uexkull HR, Mutert E. Global extent, development and economic-impact of acid soils. Plant Soil. 1995;171(1):1–15. doi:10.1007/BF00009558.
- Chauhan DK, Yadav V, Vaculík M, Gassmann W, Pike S, Arif N, Singh VP, Deshmukh R, Sahi S, Tripathi DK. Aluminum toxicity and aluminum stress-induced physiological tolerance responses in higher plants. Crit Rev Biotechnol. 2021;41(5):715–730. doi:10.1080/07388551.2021.1874282.
- Kochian LV, Hoekenga OA, Pineros MA. How do crop plants tolerate acid soils? Mechanisms of aluminum tolerance and phosphorous efficiency. Annu Rev Plant Biol. 2004;55(1):459–493. doi:10.1146/annurev.arplant.55.031903.141655.
- Ma JF. Syndrome of aluminum toxicity and diversity of aluminum resistance in higher plants. Int Rev Cytol. 2007;264:225–252.
- Jones DL, Kochian LV. Aluminum inhibition of the inositol 1,4,5-trisphosphate signal transduction pathway in wheat roots: a role in aluminum toxicity? Plant Cell. 1995;7(11):1913–1922. doi:10.2307/3870198.
- Barceló J, Poschenrieder C. Fast root growth responses, root exudates, and internal detoxification as clues to the mechanisms of aluminium toxicity and resistance: a review. Environ Exp Bot. 2002;48(1):75–92. doi:10.1016/S0098-8472(02)00013-8.
- Kochian LV, Piñeros MA, Liu J, Magalhaes JV. Plant adaptation to acid soils: the molecular basis for crop aluminum resistance. Annu Rev Plant Biol. 2015;66(1):571–598. doi:10.1146/annurev-arplant-043014-114822.
- Zhang F, Yan X, Han X, Tang R, Chu M, Yang Y, Yang YH, Zhao F, Fu A, Luan S, et al. A defective vacuolar proton pump enhances aluminum tolerance by reducing vacuole sequestration of organic acids. Plant Physiol. 2019;181(2):743–761. doi:10.1104/pp.19.00626.
- Sun P, Tian QY, Chen J, Zhang WH. Aluminium-induced inhibition of root elongation in Arabidopsis is mediated by ethylene and auxin. J Exp Bot. 2010;61(2):347–356. doi:10.1093/jxb/erp306.
- Liu G, Gao S, Tian H, Wu W, Robert HS, Ding Z. Local transcriptional control of YUCCA regulates auxin promoted root-growth inhibition in response to aluminium stress in Arabidopsis. PLoS Genet. 2016;12:e1006360. doi:10.1371/journal.pgen.1006360.
- Li Z, Xing D. Mechanistic study of mitochondria-dependent programmed cell death induced by aluminium phytotoxicity using fluorescence techniques. J Exp Bot. 2011;62(1):331–343. doi:10.1093/jxb/erq279.
- Liu J, Li Z, Wang Y, Xing D. Overexpression of ALTERNATIVE OXIDASE1a alleviates mitochondria-dependent programmed cell death induced by aluminium phytotoxicity in Arabidopsis. J Exp Bot. 2014;65(15):4465–4478. doi:10.1093/jxb/eru222.
- Pereira JF, Ryan PR. The role of transposable elements in the evolution of aluminium resistance in plants. J Exp Bot. 2019;70(1):41–54. doi:10.1093/jxb/ery357.
- Singh S, Tripathi DK, Singh S, Sharma S, Dubey NK, Chauhan DK, Vaculík M. Toxicity of aluminium on various levels of plant cells and organism. Environ Exp Bot. 2017;137:177–193. doi:10.1016/j.envexpbot.2017.01.005.
- Zhang M, Lu X, Li C, Zhang B, Zhang C, Zhang XS, Ding Z. Auxin efflux carrier ZmPGP1 mediates root growth inhibition under aluminum stress. Plant Physiol. 2018;177(2):819–832. doi:10.1104/pp.17.01379.
- Liu Y, Xu J, Guo S, Yuan X, Zhao S, Tian H, Dai S, Kong X, Ding Z. AtHB7/12 regulate root growth in response to aluminum stress. Int J Mol Sci. 2020;21(11):4080. doi:10.3390/ijms21114080.
- Matsumoto H. Cell biology of aluminum toxicity and tolerance in higher plants. Int Rev Cytol. 2000;200:1–46.
- Horst WJ, Wang Y, Eticha D. The role of the root apoplast in aluminium-induced inhibition of root elongation and in aluminium resistance of plants. Ann Bot. 2010;106(1):185–197. doi:10.1093/aob/mcq053.
- Sivaguru M, Horst WJ. The distal part of the transition zone is the most aluminum-sensitive apical root zone of maize. Plant Physiol. 1998;116(1):155–163. doi:10.1104/pp.116.1.155.
- Panda SK, Baluska F, Matsumoto H. Aluminum stress signaling in plants. Plant Signal Behav. 2009;4(7):592–597. doi:10.4161/psb.4.7.8903.
- Nezames CD, Sjogren CA, Barajas JF, Larsen PB. The Arabidopsis Cell Cycle Checkpoint Regulators TANMEI/ALT2 and ATR Mediate the Active Process of Aluminum-Dependent Root Growth Inhibition. Plant Cell. 2012;24(2):608–621. doi:10.1105/tpc.112.095596.
- Ma JF, Chen ZC, Shen RF. Molecular mechanisms of Al tolerance in gramineous plants. Plant Soil. 2014;381(1–2):1–12. doi:10.1007/s11104-014-2073-1.
- Geng X, Horst WJ, Golz JF, Lee JE, Ding Z, Yang ZB. LEUNIG_HOMOLOG transcriptional co-repressor mediates aluminium sensitivity through PECTIN METHYLESTERASE46 -modulated root cell wall pectin methylesterification in Arabidopsis. Plant J. 2017;90(3):491–504. doi:10.1111/tpj.13506.
- Poschenrieder C, Gunsé B, Corrales I, Barceló J. A glance into aluminum toxicity and resistance in plants. Sci Total Environ. 2008;400(1–3):356–368. doi:10.1016/j.scitotenv.2008.06.003.
- Horst WJ. The role of the apoplast in aluminum toxicity and resistance of higher plants. Z Pflanzenernahr Bodenk. 1995;158(5):419–428. doi:10.1002/jpln.19951580503.
- Rangel AF, Rao IM, Horst WJ. Intracellular distribution and binding state of aluminum in root apices of two common bean (Phaseolus vulgaris) genotypes in relation to Al toxicity. Physiol Plantarum. 2009;135(2):162–173. doi:10.1111/j.1399-3054.2008.01183.x.
- Kopittke PM, Menzies NW, Wang P, McKenna BA, Wehr JB, Lombi E, Kinraide TB, Blamey FP. The rhizotoxicity of metal cations is related to their strength of binding to hard ligands. Environ Toxicol Chem. 2014;33(2):268–277. doi:10.1002/etc.2435.
- Schmohl N, Horst WJ. Cell-wall composition modulates aluminium toxicity. Springer Netherlands. 2001;92:262–263.
- Mao C, Yi K, Yang L, Zheng B, Wu Y, Liu F, Wu P. Identification of aluminium-regulated genes by cDNA-AFLP in rice (Oryza sativa L.): aluminium-regulated genes for the metabolism of cell wall components. J Exp Bot. 2004;55(394):137–143. doi:10.1093/jxb/erh030.
- Volkov RA, Panchuk II, Mullineaux PM, Schöffl F. Heat stress-induced H2O2 is required for effective expression of heat shock genes in Arabidopsis. Plant Mol Biol. 2006;61(4–5):733–746. doi:10.1007/s11103-006-0045-4.
- Königshofer H, Tromballa HW, Löppert HG. Early events in signalling high-temperature stress in tobacco BY2 cells involve alterations in membrane fluidity and enhanced hydrogen peroxide production. Plant Cell Environ. 2008;31(12):1771–1780. doi:10.1111/j.1365-3040.2008.01880.x.
- Yamamoto Y, Kobayashi Y, Devi SR, Rikiishi S, Matsumoto H. Oxidative stress triggered by aluminum in plant roots. Plant Soil. 2003:255, 239–243.
- Du H, Huang Y, Qu M, Li Y, Hu X, Yang W, Li H, He W, Ding J, Liu C, et al. A Maize ZmAT6 gene confers aluminum tolerance via reactive oxygen species scavenging. Front Plant Sci. 2020;11:1016. doi:10.3389/fpls.2020.01016.
- Exley C. The pro-oxidant activity of aluminum. Free Radic Biol Med. 2004;36(3):380–387. doi:10.1016/j.freeradbiomed.2003.11.017.
- Kochian LV, Piñeros MA, Hoekenga OA. The physiology, genetics and molecular biology of plant aluminum resistance and toxicity. Plant Soil. 2005;274(1–2):175–195. doi:10.1007/s11104-004-1158-7.
- Huang J, Han R, Ji F, Yu Y, Wang R, Hai Z, Liang W, Wang H. Glucose-6-phosphate dehydrogenase and abscisic acid mediate programmed cell death induced by aluminum toxicity in soybean root tips. J Hazard Mater. 2022;425:127964. doi:10.1016/j.jhazmat.2021.127964.
- Yamamoto Y, Kobayashi Y, Matsumoto H. Lipid peroxidation is an early symptom triggered by aluminum, but not the primary cause of elongation inhibition in pea roots. Plant Physiol. 2001;125(1):199–208. doi:10.1104/pp.125.1.199.
- Hossain MA, Hossain AKMZ, Kihara T, Koyama H, Hara T. Aluminum-induced lipid peroxidation and lignin deposition are associated with an increase in H2O2 generation in wheat seedlings. Soil Sci Plant Nutr. 2005:51, 223–230.
- Sujkowska-Rybkowska M. Reactive oxygen species production and antioxidative defense in pea (Pisum sativum l.) root nodules after short-term aluminum treatment. Acta Physiol Plant. 2012;34(4):1387–1400. doi:10.1007/s11738-012-0935-9.
- Cao H, Wu D, Shen H. AtPIN2 is involved in aluminum tolerance by affecting antioxidant capacity in the roots of Arabidopsis. J Plant Physiol. 2015;51:377–384.
- Sade H, Meriga B, Surapu V, Gadi J, Sunita MS, Suravajhala P, Kavi Kishor PB. Toxicity and tolerance of aluminum in plants: tailoring plants to suit to acid soils. Biometals. 2016;29(2):187–210. doi:10.1007/s10534-016-9910-z.
- Jaskowiak J, Kwasniewska J, Milewska-Hendel A, Kurczynska EU, Szurman-Zubrzycka M, Szarejko I. Aluminum alters the histology and pectin cell wall composition of barley roots. Int J Mol Sci. 2019;20(12):3039. doi:10.3390/ijms20123039.
- Wei P, Demulder M, David P, Eekhout T, Yoshiyama KO, Nguyen L, Vercauteren I, Eeckhout D, Galle M, De Jaeger G, et al. Arabidopsis casein kinase 2 triggers stem cell exhaustion under Al toxicity and phosphate deficiency through activating the DNA damage response pathway. Plant Cell. 2021;33(4):1361–1380. doi:10.1093/plcell/koab005.
- Rengel Z, Zhang WH. Role of dynamics of intracellular calcium in aluminium-toxicity syndrome. New Phytol. 2003;159(2):295–314. doi:10.1046/j.1469-8137.2003.00821.x.
- Taylor GJ. The Physiology of Aluminum Phytotoxicity. Sigel AH, Sigel A, editors. metal ions in biological systems. Marcel Dekker New York: Vol. 1988. 123–163.
- Rengel Z, Elliott DC. Mechanism of Aluminum Inhibition of Net 45Ca2+ Uptake by Amaranthus Protoplasts. Plant Physiol. 1992;98(2):632–638. doi:10.1104/pp.98.2.632.
- Gassmann W, Schroeder JI. Inward-rectifying K+ channels in root hairs of wheat (A mechanism for aluminum-sensitive low-affinity K+ uptake and membrane potential control). Plant Physiol. 1994;105(4):1399–1408. doi:10.1104/pp.105.4.1399.
- Gaxiola RA, Palmgren MG, Schumacher K. Plant proton pumps. FEBS Lett. 2007:581, 2204–2214.
- Schumacher K, Krebs M. The V-ATPase: small cargo, large effects. Curr Opin Plant Biol. 2010;13(6):724–730. doi:10.1016/j.pbi.2010.07.003.
- Delhaize E, Gruber BD, Ryan PR. The roles of organic anion permeases in aluminium resistance and mineral nutrition. FEBS Lett. 2007;581(12):2255–2262. doi:10.1016/j.febslet.2007.03.057.
- Zhu XF, Shi YZ, Lei GJ, Fry SC, Zhang BC, Zhou YH, Braam J, Jiang T, Xu XY, Mao CZ, et al. XTH31, Encoding an in Vitro XEH/XET-Active Enzyme, Regulates Aluminum Sensitivity by Modulating in Vivo XET Action, Cell Wall Xyloglucan Content, and Aluminum Binding Capacity in Arabidopsis. Plant Cell. 2012;24(11):4731–4747. doi:10.1105/tpc.112.106039.
- Li YY, Zhang YJ, Zhou Y, Yang JL, Zheng SJ. Protecting cell walls from binding aluminum by organic acids contributes to aluminum resistance. J Integr Plant Biol. 2009:51, 574–580.
- Clarkson DT. Interactions between aluminum and phosphorus on root surfaces and cell wall material. Plant Soil. 1967;27(3):347–356. doi:10.1007/BF01376328.
- Ma JF, Yamamoto R, Nevins DJ, Matsumoto H, Brown PH. Al binding in the epidermis cell wall inhibits cell elongation of okra hypocotyl. Plant Cell Physiol. 1999;40(5):549–556. doi:10.1093/oxfordjournals.pcp.a029576.
- Taylor GJ, McDonald-Stephens JL, Hunter DB, Bertsch PM, Elmore D, Rengel Z, Reid RJ. Direct Measurement of Aluminum Uptake and Distribution in Single Cells of Chara corallina. Plant Physiol. 2000;123(3):987–996. doi:10.1104/pp.123.3.987.
- Schmohl N, Pilling J, Fisahn J, Horst WJ. Pectin methylesterase modulates aluminium sensitivity in Zea mays and Solanum tuberosum. Physiol Plant. 2000;109(4):419–427. doi:10.1034/j.1399-3054.2000.100408.x.
- Li CX, Yan JY, Ren JY, Sun L, Xu C, Li GX, Ding ZJ, Zheng SJ. A WRKY transcription factor confers aluminum tolerance via regulation of cell wall modifying genes. J Integr Plant Biol. 2020;62(8):1176–1192. doi:10.1111/jipb.12888.
- Yang JL, Zhu XF, Peng YX, Zheng C, Li GX, Liu Y, Shi YZ, Zheng SJ. Cell wall hemicellulose contributes significantly to aluminum adsorption and root growth in Arabidopsis. Plant Physiol. 2011;155(4):1885–1892. doi:10.1104/pp.111.172221.
- Sivaguru M, Ezaki B, He ZH, Tong H, Osawa H, Baluska F, Volkmann D, Matsumoto H. Aluminum-induced gene expression and protein localization of a cell wall-associated receptor kinase in Arabidopsis. Plant Physiol. 2003;132(4):2256–2266. doi:10.1104/pp.103.022129.
- Mangeon A, Pardal R, Menezes-Salgueiro AD, Duarte GL, de Seixas R, Cruz FP, Cardeal V, Magioli C, Ricachenevsky FK, Margis R, et al. AtGRP3 is implicated in root size and aluminum response pathways in Arabidopsis. PLoS One. 2016;11(3):e0150583. doi:10.1371/journal.pone.0150583.
- Eticha D, Stass A, Horst WJ. Cell-wall pectin and its degree of methylation in the maize root-apex: significance for genotypic differences in aluminium resistance. Plant Cell Environ. 2005;28(11):1410–1420. doi:10.1111/j.1365-3040.2005.01375.x.
- Horst WJ, Kollmeier M, Schmohl N, Sivaguru M, Wang Y, Felle HH, Hedrich R, Schröder W, Staß A. Significance of the root apoplast for aluminium toxicity and resistance of maize. In: Sattelmacher B, Horst W, editors. The apoplast of higher plants: compartment of storage, transport, and reactions. Dordrecht: Kluwer Academic Publishers; 2007. p. 49–66.
- Liu J, Magalhaes JV, Shaff J, Kochian LV. Aluminum-activated citrate and malate transporters from the MATE and ALMT families function independently to confer Arabidopsis aluminum tolerance. Plant J. 2009;57(3):389–399. doi:10.1111/j.1365-313X.2008.03696.x.
- Shen H, He LF, Sasaki T, Yamamoto Y, Zheng SJ, Ligaba A, Yan XL, Ahn SJ, Yamaguchi M, Sasakawa H, et al. Citrate secretion coupled with the modulation of soybean root tip under aluminum stress. Up-regulation of transcription, translation, and threonine-oriented phosphorylation of plasma membrane H+-ATPase. Plant Physiol. 2005;138(1):287–296. doi:10.1104/pp.104.058065.
- Liang C, Piñeros MA, Tian J, Yao Z, Sun L, Liu J, Shaff J, Coluccio A, Kochian LV, Liao H. Low pH, Aluminum, and Phosphorus Coordinately Regulate Malate Exudation through GmALMT1 to Improve Soybean Adaptation to Acid Soils. Plant Physiol. 2013;161(3):1347–1361. doi:10.1104/pp.112.208934.
- Wang Z, Liu L, Su H, Guo L, Zhang J, Li Y, Xu J, Zhang X, Guo YD, Zhang N. Jasmonate and aluminum crosstalk in tomato: identification and expression analysis of WRKYs and ALMTs during JA/Al-regulated root growth. Plant Physiol Biochem. 2020;154:409–418. doi:10.1016/j.plaphy.2020.06.026.
- Hoekenga OA, Maron LG, Piñeros MA, Cançado GM, Shaff J, Kobayashi Y, Ryan PR, Dong B, Delhaize E, Sasaki T, et al. AtALMT1, which encodes a malate transporter, is identified as one of several genes critical for aluminum tolerance in Arabidopsis. Proc Natl Acad Sci U S A. 2006;103(25):9738–9743. doi:10.1073/pnas.0602868103.
- Maron LG, Piñeros MA, Guimarães CT, Magalhaes JV, Pleiman JK, Mao C, Shaff J, Belicuas SN, Kochian LV. Two functionally distinct members of the MATE (multi-drug and toxic compound extrusion) family of transporters potentially underlie two major aluminum tolerance QTLs in maize. Plant J. 2010;61(5):728–740. doi:10.1111/j.1365-313X.2009.04103.x.
- Kobayashi Y, Hoekenga OA, Itoh H, Nakashima M, Saito S, Shaff JE, Maron LG, Piñeros MA, Kochian LV, Koyama H. Characterization of AtALMT1 Expression in Aluminum-Inducible Malate Release and Its Role for Rhizotoxic Stress Tolerance in Arabidopsis. Plant Physiol. 2007;145(3):843–852. doi:10.1104/pp.107.102335.
- Sawaki Y, Iuchi S, Kobayashi Y, Kobayashi Y, Ikka T, Sakurai N, Fujita M, Shinozaki K, Shibata D, Kobayashi M, et al. STOP1 regulates multiple genes that protect Arabidopsis from proton and aluminum toxicities. Plant Physiol. 2009;150(1):281–294. doi:10.1104/pp.108.134700.
- Balzergue C, Dartevelle T, Godon C, Laugier E, Meisrimler C, Teulon JM, Creff A, Bissler M, Brouchoud C, Hagège A, et al. Low phosphate activates STOP1-ALMT1 to rapidly inhibit root cell elongation. Nat commun. 2017;8(1):15300. doi:10.1038/ncomms15300.
- Cardoso TB, Pinto RT, Paiva LV. Comprehensive characterization of the ALMT and MATE families on Populus trichocarpa and gene co-expression network analysis of its members during aluminium toxicity and phosphate starvation stresses. 3 Biotech. 2020;10(12):525. doi:10.1007/s13205-020-02528-3.
- Ribeiro AP, Vinecky F, Duarte KE, Santiago TR, Das Chagas Noqueli Casari RA, Hell AF, da Cunha B, Martins PK, da Cruz Centeno D, de Oliveira Molinari PAH, et al. Enhanced aluminum tolerance in sugarcane: evaluation of SbMATE overexpression and genome-wide identification of ALMTs in Saccharum spp. BMC Plant Biol. 2021;21(1):300. doi:10.1186/s12870-021-02975-x.
- Zhu X, Wang P, Bai Z, Herde M, Ma Y, Li N, Liu S, Huang CF, Cui R, Ma H, et al. Calmodulin-like protein CML24 interacts with CAMTA2 and WRKY46 to regulate ALMT1 -dependent Al resistance in Arabidopsis thaliana. New Phytol. 2022;233(6):2471–2487. doi:10.1111/nph.17812.
- Sasaki T, Yamamoto Y, Ezaki B, Katsuhara M, Ahn SJ, Ryan PR, Delhaize E, Matsumoto H. A wheat gene encoding an aluminum-activated malate transporter. Plant J. 2004;37(5):645–653. doi:10.1111/j.1365-313X.2003.01991.x.
- Xu J, Zhu J, Liu J, Wang J, Ding Z, Tian H. SIZ1 negatively regulates aluminum resistance by mediating the STOP1–ALMT1 pathway in Arabidopsis. J Integr Plant Biol. 2021;63(6):1147–1160. doi:10.1111/jipb.13091.
- Chen W, Tang L, Wang J, Zhu H, Jin J, Yang J, Fan W. Research advances in the mutual mechanisms regulating response of plant roots to phosphate deficiency and aluminum toxicity. Int J Mol Sci. 2022;23(3):1137. doi:10.3390/ijms23031137.
- Wang J, Yu X, Ding ZJ, Zhang X, Luo Y, Xu X, Xie Y, Li X, Yuan T, Zheng SJ, et al. Structural basis of ALMT1-mediated aluminum resistance in Arabidopsis. Cell Res. 2022;32(1):89–98. doi:10.1038/s41422-021-00587-6.
- Ding ZJ, Yan JY, Xu XY, Li GX, Zheng SJ. WRKY46 functions as a transcriptional repressor of ALMT, regulating aluminum-induced malate secretion in Arabidopsis. Plant J. 2013;76(5):825–835. doi:10.1111/tpj.12337.
- Yokosho K, Yamaji N, Ma JF. An Al-inducible MATE gene is involved in external detoxification of Al in rice. Plant J. 2011;68(6):1061–1069. doi:10.1111/j.1365-313X.2011.04757.x.
- Rogers EE, Wu X, Stacey G, Nguyen HT. Two MATE proteins play a role in iron efficiency in soybean. J Plant Physiol. 2009;166(13):1453–1459. doi:10.1016/j.jplph.2009.02.009.
- Yokosho K, Yamaji N, Ma JF. Isolation and characterization of two MATE genes in rye. Func Plant Biol. 2010;37(4):296–303. doi:10.1071/FP09265.
- Larsen PB, Geisler MJ, Jones CA, Williams KM, Cancel JD. ALS3 encodes a phloem-localized ABC transporter-like protein that is required for aluminum tolerance in Arabidopsis. Plant J. 2005;41(3):353–363. doi:10.1111/j.1365-313X.2004.02306.x.
- Fang Q, Zhang J, Zhang Y, Fan N, van den Burg HA, Huang CF. Regulation of aluminum resistance in Arabidopsis involves the SUMOylation of the zinc finger transcription factor STOP1. Plant Cell. 2020;32(12):3921–3938. doi:10.1105/tpc.20.00687.
- Guo J, Zhang Y, Gao H, Li S, Wang ZY, Huang CF. Mutation of HPR1 encoding a component of the THO/TREX complex reduces STOP1 accumulation and aluminium resistance in Arabidopsis thaliana. New Phytol. 2020;228(1):179–193. doi:10.1111/nph.16658.
- Magalhaes JV, Liu J, Guimarães CT, Lana UG, Alves VM, Wang YH, Schaffert RE, Hoekenga OA, Piñeros MA, Shaff JE, et al. A gene in the multidrug and toxic compound extrusion (MATE) family confers aluminum tolerance in sorghum. Nat Genet. 2007;39(9):1156–1161. doi:10.1038/ng2074.
- Furukawa J, Yamaji N, Wang H, Mitani N, Murata Y, Sato K, Katsuhara M, Takeda K, Ma JF. An aluminum-activated citrate transporter in barley. Plant Cell Physiol. 2007;48(8):1081–1091. doi:10.1093/pcp/pcm091.
- Ryan PR, Raman H, Gupta S, Horst WJ, Delhaize E. A second mechanism for aluminum resistance in wheat relies on the constitutive efflux of citrate from roots. Plant Physiol. 2009;149(1):340–351. doi:10.1104/pp.108.129155.
- Yokosho K, Yamaji N, Ma JF. Global transcriptome analysis of Al-induced genes in an Al-accumulating species, common buckwheat (Fagopyrum esculentum Moench). Plant Cell Physiol. 2014;55(12):2077–2091. doi:10.1093/pcp/pcu135.
- Lei GJ, Yokosho K, Yamaji N, Ma JF. Two MATE transporters with different subcellular localization are involved in Al tolerance in buckwheat. Plant Cell Physiol. 2017;58(12):2179–2189. doi:10.1093/pcp/pcx152.
- Murtas G, Reeves PH, Fu YF, Bancroft I, Dean C, Coupland G. A nuclear protease required for flowering-time regulation in Arabidopsis reduces the abundance of SMALL UBIQUITIN-RELATED MODIFIER conjugates. Plant Cell. 2003:15, 2308–2319.
- Xu XM, Rose A, Muthuswamy S, Jeong SY, Venkatakrishnan S, Zhao Q, Meier I. NUCLEAR PORE ANCHOR, the Arabidopsis Homolog of Tpr/Mlp1/Mlp2/Megator, Is Involved in mRNA Export and SUMO Homeostasis and Affects Diverse Aspects of Plant Development. Plant Cell. 2007;19(5):1537–1548. doi:10.1105/tpc.106.049239.
- Fang Q, Zhou F, Zhang Y, Singh S, Huang CF. Degradation of STOP1 mediated by the F-box proteins RAH1 and RAE1 balances aluminum resistance and plant growth in Arabidopsis thaliana. Plant J. 2021;106(2):493–506. doi:10.1111/tpj.15181.
- Huang CF, Yamaji N, Mitani N, Yano M, Nagamura Y, Ma JF. A bacterial-type ABC transporter is involved in aluminum tolerance in rice. Plant Cell. 2009;21(2):655–667. doi:10.1105/tpc.108.064543.
- Larsen PB, Cancel J, Rounds M, Ochoa V. Arabidopsis ALS1 encodes a root tip and stele localized half type ABC transporter required for root growth in an aluminum toxic environment. Planta. 2007:225, 1447–1458.
- Degenhardt J, Larsen PB, Howell SH, Kochian LV. Aluminum Resistance in the Arabidopsis Mutant alr −104 Is Caused by an Aluminum-Induced Increase in Rhizosphere pH1. Plant Physiol. 1998;117(1):19–27. doi:10.1104/pp.117.1.19.
- Kidd PS, Llugany M, Poschenrieder C, Gunsé B, Barceló J. The role of root exudates in aluminium resistance and silicon-induced amelioration of aluminium toxicity in three varieties of maize (Zea mays L.). J Exp Bot. 2001;52:1339–1352.
- Li C, Liu G, Geng X, He C, Quan T, Hayashi KI, De Smet I, Robert HS, Ding Z, Yang ZB. Local regulation of auxin transport in root-apex transition zone mediates aluminium-induced Arabidopsis root-growth inhibition. Plant J. 2021;108(1):55–66. doi:10.1111/tpj.15424.
- Wu D, Shen H, Yokawa K, Baluška F. Alleviation of aluminium-induced cell rigidity by overexpression of OsPIN2 in rice roots. J Exp Bot. 2014;65(18):5305–5315. doi:10.1093/jxb/eru292.
- Yang ZB, Liu G, Liu J, Zhang B, Meng W, Müller B, Hayashi KI, Zhang X, Zhao Z, De Smet I, et al. Synergistic action of auxin and cytokinin mediates aluminum-induced root growth inhibition in Arabidopsis. EMBO Rep. 2017;18(7):1213–1230. doi:10.15252/embr.201643806.
- Yang ZB, He C, Ma Y, Herde M, Ding Z. Jasmonic acid enhances Al-induced root growth inhibition. Plant Physiol. 2017;173(2):1420–1433. doi:10.1104/pp.16.01756.
- Ranjan A, Sinha R, Lal SK, Bishi SK, Singh AK. Phytohormone signalling and cross-talk to alleviate aluminium toxicity in plants. Plant Cell Rep. 2021;40(8):1331–1343. doi:10.1007/s00299-021-02724-2.
- Fendrych M, Akhmanova M, Merrin J, Glanc M, Hagihara S, Takahashi K, Uchida N, Torii KU, Friml J. Rapid and reversible root growth inhibition by TIR1 auxin signalling. Nat Plants. 2018;4(7):453–459. doi:10.1038/s41477-018-0190-1.
- Li L, Verstraeten I, Roosjen M, Takahashi K, Rodriguez L, Merrin J, Chen J, Shabala L, Smet W, Ren H, et al. Cell surface and intracellular auxin signalling for H+ fluxes in root growth. Nature. 2021:599, 273–277.
- Bai B, Bian H, Zeng Z, Hou N, Shi B, Wang J, Zhu M, Han N. miR393-mediated auxin signaling regulation is involved in root elongation inhibition in response to toxic aluminum stress in barley. Plant Cell Physiol. 2017;58(3):426–439. doi:10.1093/pcp/pcw211.
- Kobayashi Y, Kobayashi Y, Sugimoto M, Lakshmanan V, Iuchi S, Kobayashi M, Bais HP, Koyama H. Characterization of the Complex Regulation of AtALMT1 Expression in Response to Phytohormones and Other Inducers. Plant Physiol. 2013;162(2):732–740. doi:10.1104/pp.113.218065.