ABSTRACT
Aconitum carmichaelii Debx. is used as traditional herbal medicine in China, Japan, and other Asian countries. A. carmichaelii has two modes for reproduction: sexual reproduction with seed and vegetative reproduction with vegetative propagules. The vegetative propagules are belowground and invisible. To date, only a handful of studies for the clonal growth are available. In this study, we investigated the clonal growth by anatomical and morphological changes. Results revealed that the axillary bud appeared on the rhizome. Furthermore, the axillary meristem in the axillary bud differentiated a bud upwards and an adventitious root (AR) downwards. The AR expanded to a tuberous root in order to provide the bud nutrients for the new plant. The AR branched LRs. In addition, some lateral roots (LRs) on the AR also swelled. Both the AR and LR were found to follow a similar pattern of development. However, high lignification in the stele region of LRs inhibited further expansion. AR development was attributed to activities of the cambium and meristem cell, starch accumulation, stele lignification, and a polyarch stele. Our study not only provides a better understanding of clonal growth but also provides clues to explore the regulatory mechanisms underlying AR development in A. carmichaelii.
Introduction
Most plants have two modes for regeneration: sexual reproduction and clonal reproduction. Both modes are long-term survival strategies that plants have evolved to adapt to variable environment. Sexual reproduction is limited by biotic and abiotic stress, which need the assistance of pollinators such as wind, water, and insects. However, clonal reproduction is autarkic through vegetative propagules that allow populations to persist in habitats or regions. Clonal plants can be produced in many ways, through rhizomes, tubers, bulbs and other organs.Citation1
Aconitum carmichaelii Debx. (Ranunculaceae) is a toxic plant that contains aconitine alkaloids.Citation2,Citation3 In China, this species has been used for more than 2000 years as traditional Chinese medicine.Citation4 Moreover, it has a cultivation history of more than 1,000 years.Citation5 The roots are mainly used as medicine. The processed mother root is called Chuanwu, whereas the processed daughter root is called Fuzi in the Chinese Pharmacopoeia.Citation6 A. carmichaelii can reproduce sexually with seed or asexually with daughter root. The daughter root, which is starchy and crowned with a bud, separates from the mother plant and become independent individuals. These offspring sprout in the next spring and begin the next life cycle.Citation7 The definition of the underground organ about the daughter root with a bud in Aconitum has always been controversial. KumazawaCitation8 considers it as a root tuber. It has recently been suggested that it is a complex of a swollen adventitious root and a bud.Citation9–11
This study is mainly to investigate the morphological and anatomical changes of the clonal growth. Results revealed that the axillary meristem in the axillary bud differentiated a bud upwards and an adventitious root (AR) downwards; AR branched LRs, and the comparison of the ARs and LRs explored the factors that affect the AR development.
Materials and methods
Plant materials
Asexual propagules of Aconitum carmichaelii Debx. were collected from Fangshi Town (104°56ʹ58.73” E, 32°21ʹ52.93” N, Altitude: 1039 m), Qingchuan County, China. These propagules were planted in the Medicinal Botanical Garden of Chengdu University of TCM (103°48ʹ18.96” E, 30°41ʹ31.89 “ N, Altitude: 504 m) on November 18, 2020.
Morphological characteristics
Seven plants selected were recorded on March 6, 2021, as the first observation day (1 D) and were finished on August 4, 2021 (152 D). The shoot height (cm) and root diameter (mm) of the first AR to appear were recorded after protrusion of ARs from the axillary buds.
Anatomical characterization
Three plants were collected for anatomical study during each observation period. The first AR to appear in each plant was collected. Samples about 3–5 mm thickness from 0 cm, 1 cm, 2 cm below the basal root axis were sectioned. Further, all basal lateral roots on the AR at 61, 92, 125, and 152 D, were also sectioned.
Fresh tissues were immediately fixed in FAA (formalin, acetic acid, 70% ethanol, 5:5:90 v/v/v). The tissues were then dehydrated, cleared and subjected to paraffin infiltration as follows: 50% alcohol, 2 h; 70% alcohol, 2 h; 85% alcohol, 2 h; 95% alcohol, 2 h; 100% alcohol I, 1 h; 100% alcohol II, 1 h; alcohol and xylene (3:1 v/v), 1 h; alcohol and xylene (2:2 v/v), 1 h; alcohol and xylene (1:3 v/v), 1 h; xylene I, 1 h; xylene II,1 h; Xylene: paraffin (1: 1 v/v, 35°C), 6 h; Paraffin I (55°C), 6 h; Paraffin II (60°C), 6 h; Paraffin III (65°C) 6 h. Subsequently, these tissue samples were placed in melted paraffin in cassettes and frozen at −20°C until the paraffin had completely solidified. The paraffin blocks were then removed and appropriately trimmed. Sections (15–28 μm) were made with a sliding microtome (HM450), stained with safranin o-fast green and mounted in neutral balsam. Finally, all sections were viewed using an Axioscope 5 with an Axiocam 208 color camera, and selected sections were scanned by NanoZoomer-S60.
Results
Morphological changes in the aconitum carmichaelii Debx
Growth changes of the seven plants obtained 152 D were recorded. The shoots had an average height of 8 cm at 1 D, which reached 101 cm at 152 D. A decrease in the growth rate of the shoot height was found at 121–152 D, with no evidence of flower buds on the shoot at 152 D. Summarily, the shoot showed a linear increase in height (). White smooth axillary buds for AR formation emerged on the rhizome near the mother root at 1 D (), and adventitious roots appeared on 43% of the plants while the others did not. Root hairs began to appear with AR elongation (). As the adventitious root diameter increased, the root hairs gradually disappeared. The base of AR began to swell, away from the root tip, and this was followed by a downward expansion (). A bud spot appeared on the adventitious root () at 31 D and was distinctly seen on 121D. The diameter of ARs increased with time. In general, the upper diameter was the largest and the middle diameter was small while the lower diameter was the smallest. At 121 D, the upper diameter was about 20 mm, the middle diameter was 15 mm and the lower diameter was 10 mm (). In addition, we found an increase in the total number of ARs. About 5–12 ARs had formed in each plant at 152 D. The AR appearing closest and furthest to the mother root had the highest and least diameters, respectively.
Figure 1. Profiles of morphological changes in Aconitum carmichaelii Debx.
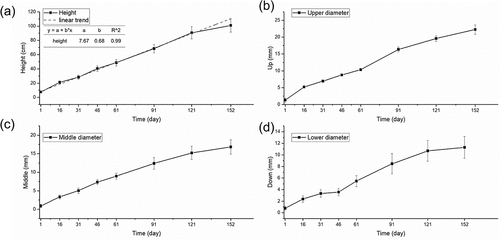
Figure 2. Anatomical changes from the adventitious root primordium to the adventitious root in A. carmichaelii.
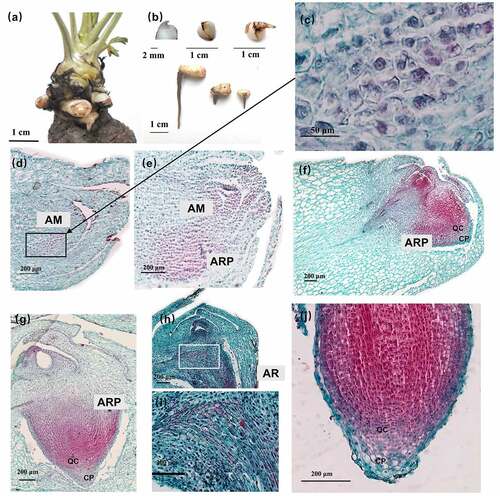
Figure 3. Developmental stages of adventitious root and initial swelling in A. carmichaelii.
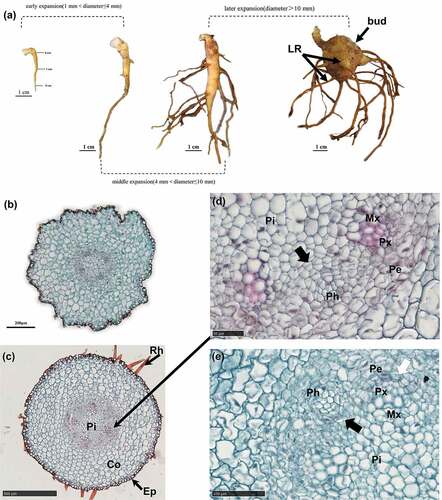
Anatomical changes during AR formation and development
There was protuberance on the bud tip, which broke downwards through the bud epidermis and then became an AR (). Three files of meristematic cells that were more stained than the surrounding cells were found in the axillary meristem (). The cells differentiated into an adventitious root primordium (ARP) with a distinct root cap (CP) and quiescent center (QC) of differentiation (). The ARP broke through the epidermis of the axillary buds to form an AR (). The whole structure is an AR with a bud (). Moreover, the AR continued elongating downwards where the area where long vessel elements (VEs) were connected with the short VEs inside the axillary bud (). The bud developed slowly while AR exhibited rapid development to the tuberous root ().
Anatomical changes during AR development
Five stages were divided according to the diameter changes of the AR development.
Primary growth stage (Φ ˂1 mm)
Primary growth occurred in the AR with a root diameter of less than 1 mm (). Notably, the primary structure showed a tetrarch-heptarch stele with a parenchymatous pith at the center (), whereas the primary phloem occupied the indentations between xylem arms.
Initial swelling (Φ = 1 mm)
Secondary growth firstly had occurred to 0 cm, then 1 cm, finally 2 cm at root vertical axis. The procambium located inside the primary phloem differentiated into a cambium segment via periclinal division. The other cambium segment developed from the pericycle at the exterior of the protoxylem poles (). Both cambium segments initiated secondary growth, which resulted in a root diameter of about 1 mm. Notably, secondary growth began when the diameter of the AR reached 1 mm, consistent with the findings by Wison and Lowe Citation12 in sweetpotato. The root had no obvious morphology.
Early expansion (1 mm˂Φ≤ 4 mm)
Both cambium segments exhibited centrifugal differentiation to numerous parenchyma cells, with evidence of starch accumulation (). Furthermore, the phloem was markedly enlarged, and the AR diameter varied from 1 to 4 mm (). In addition, LRs appeared on the AR (). The primary growth stage was very brief, whereas secondary growth started almost immediately after AR emergence. Although the increase in the root diameter of the ARs was not apparent, the first three stages occurred at 0 cm of the AR.
Figure 4. Early and middle expansion.
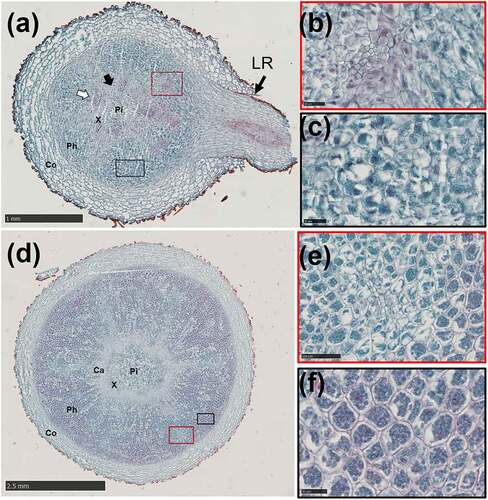
Middle expansion (4 mm ˂Φ≤ 10 mm)
Both cambium segments merged into a ring (), and there were meristematic cells around the primary sieve elements (SEs) (). Activities of the cambium and meristematic cells led to a proliferation of the starch-storing parenchyma cells (SPCs), which was followed by starch accumulation and a further increase in cell size (). Proliferation and volume increase in the SPCs led to phloem enlargement () phenomenon that subsequently caused a uniform increase in root diameter to about 10 mm.
Later expansion (Φ> 10 mm)
The cambium ring showed further differentiation of the SPCs, to form xylem and phloem on the inside and outside, respectively. The SPCs outside the cambium showed tangential elongation whereas the parenchyma cells inside revealed radial elongation. Moreover, meristematic cells surrounding the vessel elements (VEs) differentiated radially into SPCs, which subsequently produced by anticlinal division separated the primary VEs first (), and later secondary VEs (). Thereafter, SPCs produced by periclinal division were added to the pith, and the vessels were arranged in a U-shape (). The proliferation of the SPCs, coupled with volume enlargement by both the cambium and meristematic cells, pushed the cambium to the outside, resulting in further expansion of the xylem (). Some cambium segments, without meristematic cells around the VEs, divided slowly resulting in a polygonal appearance of the cambium ring (). This cambium segment might be trapped in the xylem (). However, the cambium segment with actively dividing meristematic cells around the VEs may separate from the cambium () resulting in a scattered vascular bundle. The phloem was significantly enlarged ()
Figure 5. Later expansion.
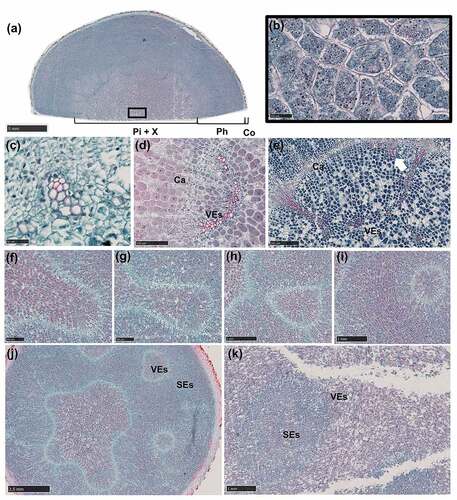
Anatomical changes during LR formation and development
LRs appeared on the AR at 16 D. LRs initiation occurred when periclinal divisions of pericycle cells in front of the primary xylem polar generated lateral root primordium (LRP) (). The LRP began to resemble the root tip passing through the cortex (), then the LR emerged from the AR (). However, only a handful of LRs showed a significant increase in root diameter at 61 D. Notably, the primary LR had a pith with a similar primary structure to that of the AR (), while xylem poles either had a triarch or tetrarch structure. However, as the LR grew, the metaxylem differentiated to the pith (). In addition, the cambium segment that developed from the procambium showed centripetal division thereby forming secondary VEs (). The high lignification in the vascular cylinder replaced the pith, while LR’s expansion pattern was similar to that observed in the middle of the AR. The high SPC differentiation in the phloem produced by meristematic cells surrounding the primary SEs () and the cambium (), contributed to the increase in the root diameter (). The non-swollen LRs at 152 D showed secondary growth as shown in () with no starch accumulation.
Figure 6. Anatomical changes in the lateral root of A. carmichaelii.
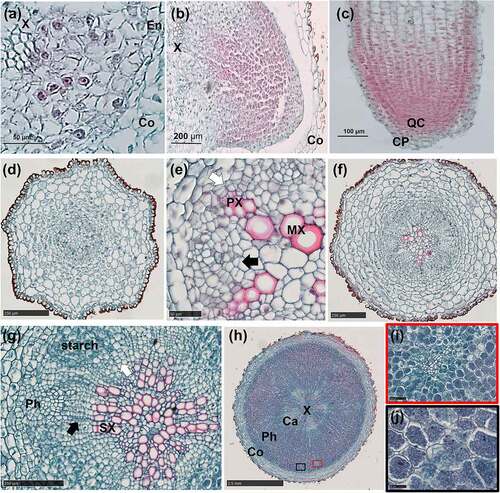
Discussion
The AR of A. carmichaelii has a different origin from its LRs.
summarizes the characters of AR and LR in A. carmichaelii.
Table 1. Characters of adventitious root (AR) and lateral root (LR)
Roots are important parts of plants, owing to the fact that they anchor them into the soil and transport water and minerals. The types of roots include primary roots, lateral roots and adventitious roots. Primary roots are derived from the embryonic radicle within a seed, lateral roots branch out from roots. The location of primary roots and lateral roots is determined while adventitious roots are not. The origin of AR is a complex and species-related process.Citation13 Previous studies have shown that ARs could form from aerial organs and initiate from different tissues, such as the pericycle,Citation14,Citation15 the cambium,Citation16,Citation17 and the parenchyma.Citation18–20 In contrast, LRs originate from the pericycle in a conservative manner.Citation21,Citation22 In this study, the daughter root is regarded as an AR that naturally initiates from the axillary meristem within the axillary bud on the rhizome of the mother root rather than an LR which is suggested by other researchers.Citation7,Citation23,Citation24 In addition, LRs branch out from an AR, which originate from the pericycle within the AR. Therefore, the origin and formation of AR are different from that of other species, and that of the LR shows a conservative manner.
The development of AR and LR is similar
Our results further revealed a similar pattern in the development of ARs and LRs. Notably, the activities of the cambium and meristematic cells and starch accumulation and high lignification are expansion factors, which subsequently contributed to an increase in the diameter of both AR and LR. Results of the present study showed that starch had accumulated as the AR and LR developed for storage. Interestingly, non-swollen LR did not show starch accumulation, suggesting that starch accumulation determines root expansion. Swelling of the phloem in both AR and LR is possibly associated with a source to sink tissues of storage reserves, which usually include starchCitation24–28 and soluble sugars.Citation29–32 We hypothesize that sources such as sucroseCitation33 are delivered through the SEs and subsequently unloaded to the amyloid within phloem’s parenchyma cells. The amyloid synthesizes starch for storage which contributes to the expansion of the phloem in the AR and LR. Qin and LiCitation34 reported similar development is performed in AR as well as primary root of A. carmichaelii; It suggests that the development manners of different roots in A. carmichaelii are conserved. In A. carmichaelii, the primary LR showed a triarch to tetrarch stele with high lignification which limits further expansion. On the other hand, AR showed a tetrarch to heptarch stele with few lignification, which subsequently developed into a tuberous root (TR),Citation35,Citation36 consistent with the studies in sweetpotato.Citation12,Citation37–39 This may be due to the fact that a polyarch stele would produce more cambium segments which are involved in the secondary growth. The increased girth of the cambium increases the root diameter. TogariCitation40 reported that lignification inhibits TR formation. In addition, transcriptional profiling found the down-regulation of lignin biosynthesis and up-regulation of starch, during the TR initiation phase in sweetpotato.Citation41 Furthermore, exogenous application of gibberellin was shown to increase lignin deposition and inhibit TR formation,Citation42,Citation43 suggesting that lignification negatively affects TR development. To date, no studies have reported the effect of lignification on TR development. The AR and LR have different functions. The major role of the LR is to transport water and minerals, so the lignification within the LR is higher. However, the AR occurs to store nutrients, so the lignification is fewer. The thickened part of the LR appears when the stored nutrients are beyond the capacity of the AR. Previous studies have suggested that xylem expansion causes TR formation in root and tuber crops, such as sweetpotato,Citation12 cassavaCitation15,Citation44 and radish.Citation45 Moreover, meristematic cells around VEs, which contribute to xylem expansion, have also been reported in TR formation.Citation12,Citation39,Citation46 In potato tuber, its expansion is mainly caused by the pith following perimedullary zone.Citation47,Citation48 The development of the AR in A. carmichaelii shows similarities with the development of the AR in the sweetpotato. Results from studies investigating the development of the AR in sweetpotato have provided relevant clues for further exploration of the underlying molecular mechanisms of AR development in A. carmichaelii.
Diverse cambium in the AR
The abnormal development of the AR was first reported by Kumazawa.Citation8 Several scholars have attempted to use the root microstructure to identify different Aconitum species. For example, Tong et al.Citation49 classified them into three types according to the arrangement of vascular bundles in the root, namely Type I (a single siphonostele), Type II (a polystele) and Type III (a number of schizostylis) categories. In this study, type I and II are observed and described in serial sections. In addition, Liu et al.Citation50 reported that cross-sections obtained from different segments showed different microscopic structures. It suggests that it would be inappropriate to use the cross-section of AR to identify species. Furthermore, our results demonstrated that the meristematic cells around the VEs also play a role in AR expansion, with their absence found to lead to the formation of scattered vascular bundles.
The role of vegetative propagation of A. carmichaelii
Axillary buds in the rhizome at the axis of the mother root sprout which subsequently elongate laterally to develop a compound vegetative propagule, the root tuberCitation51 in A. carmichaelii. The root tuber consists of an apical bud and an enlarged AR. The root tuber is hidden underground to cope with adverse conditions and stores water and nutrients to develop into a new plant in the next spring. This mode of vegetative propagation provides multiple options for the reproduction of A. carmichaelii in addition to sexual reproduction. This reproductive mode, which differentiates from an axillary bud downward to an AR, and upwards to a bud, is commonly found in many species in subgenus Aconitum.
Species of subgenus Aconitum has clonal growth with root tuber and sexual growth with seed. Both growths compete for nutrients provided by the mother root. Tang et al.Citation7 reported that the clonal growth of A. kusnezoffii developed to 59 D, after which the sexual growth began to compete for the nutrients with flower bud appearance. In this study, we found that the clonal growth of A. carmichaelii had been basically completed at 152 D. During this time, no flower buds had been produced. A. carmichaelii tends to allocate more biomass to clonal growth and less to sexual growth. The vegetative reproduction of A. carmichaelii is promoted over sexual reproduction. A. carmichaelii is an octoploid species which is grown in the Sichuan province of ChinaCitation52,Citation53 whereas A. kusnezoffii exhibits either diploids or tetraploids.Citation54–56 Previous studies show that polyploidy and vegetative reproduction are positively correlated across the angiosperms.Citation57,Citation58 A recent study suggests that polyploidy promotes vegetative reproduction.Citation59 Therefore, the increased vegetative reproduction of A. carmichaelii could be related to polyploidization.
Conclusion
In summary, the study focused on clonal growth in A. carmichaelii by anatomical and morphological changes. AR has a similar development pattern to LR in A. carmichaelii. However, LR’s high stele lignification inhibits further expansion. Moreover, AR development is attributed to the activities of the cambium and meristematic cells, as well as starch accumulation, a polyarchy stele, and stele lignification. These results provide clues to the next step in uncovering potential molecular regulatory mechanisms of AR development in A. carmichaelii. The clonal growth provide new insights into the formation of root tuber in plants.
Author contributions
Jing Gao and Ran Liu designed and wrote the manuscript. Min Luo designed the figures. Guangzhi Wang reviewed and edited the manuscript.
Acknowledgments
We sincerely apologize to those authors whose works we could not cite in this review due to space limitations.
Disclosure statement
No potential conflict of interest was reported by the author(s).
Additional information
Funding
References
- Klimesˇova´ J, de Bello F. CLO-PLA the database of clonal and bud bank traits of Central European flora. J Veg Sci. 2009;20(3):511–10. doi:10.1111/j.1654-1103.2009.01050.x.
- He Y-N, S-P O, Xiong X, Pan Y, Pei J, Xu R-C, Geng F-N, HAN L, ZHANG D-K, YANG M, et al. Stems and leaves of Aconitum carmichaelii Debx. As potential herbal resources for treating rheumatoid arthritis: chemical analysis, toxicity and activity evaluation. Chin J Nat Med. 2018;16(9):644–652. doi:10.1016/s1875-5364(18)30104-3.
- Jaiswal Y, Liang Z, Ho A, Wong L, Yong P, Chen H, Zhao Z. Distribution of toxic alkaloids in tissues from three herbal medicine Aconitum species using laser micro-dissection, UHPLC–QTOF MS and LC–MS/MS techniques. Phytochemistry. 2014;107:155–174. doi:10.1016/j.phytochem.2014.07.026.
- Wu P, Sun X, Sun F, Dai M, Huang Z, Yu Z, Cao Y. Shennong Materia Medica. Nanning (China): Guangxi Science and Technology Press , 2016. [in Chinese].
- Su J. The new revision of the materia medica. Shanghai (China): Shanghai Science and Technology Press; 1957. [in Chinese].
- Chinese Pharmacopoeia Commission. The pharmacopoeia of the people’s Republic of China: 2020 edition. part one.Vol. 40. Beijing (China): China Medical Science and Technology Press; 2020. pp. 200. [in Chinese].
- Tang M, Zhao W, Xing M, Zhao J, Jiang Z, You J, Ni B, Ni Y, Liu C, Li J. Resource allocation strategies among vegetative growth, sexual reproduction, asexual reproduction and defense during growing season of Aconitum kusnezoffii Reichb. Plant J. 2021;105(4):957–977. doi:10.1111/tpj.15080.
- Kumazawa M. Developmental history of the abnormal structure in the geophilous organ of aconitum. The Botanical Magazine. 1937;51(612):914–925. doi:10.15281/jplantres1887.51.914.
- Dong Z-M, Z-L L. The development anatomy of the monkshood-tuber of tuber of Aconitum kusnezoffii. Aca Botanica Sinica. 1990;32:7–12. [in Chinese].
- Yan J-J. Studies on developmental anatomical structure and seasonal alkaloids change of Aconitum carmichaelii [MSc thesis]. Xianyang (China): Northwest A&F University, 2008. [in Chinese].
- Qin M. Sudies on the formation of prepared daughter root of common monkshood. China J Chinese Materia Med . 1991;16:526–528. [in Chinese].
- Wison LA, Lowe SB. The anatomy of the root system in West Indian sweetpotato. Ann Bot. 1973;37(3):633–643. doi:10.1093/oxfordjournals.aob.a084729.
- Lakehala A, Bellinia C. Control of adventitious root formation: insights into synergistic and antagonistic hormonal interactions. Physiol Plant. 2019;165(1):90–100. doi:10.1111/ppl.12823.
- Sukumar P, Maloney GS, Muday GK. Localized induction of the ATP-binding cassette B19 auxin transporter enhances adventitious root formation in Arabidopsis. Plant Physiol. 2013;162(3):1392–1405. doi:10.1104/pp.113.217174.
- Chaweewan Y, Taylor N. Anatomical assessment of root formation and tuberization in cassava (Manihot esculenta Crantz). Trop Plant Biol. 2015;8(1–2):1–8. doi:10.1007/s12042-014-9145-5.
- Fandino ACA, Kim H, Rademaker JD, Lee J-Y. Reprogramming of the cambium regulators during adventitious root development upon wounding of storage tap roots in radish (Raphanus sativus L.). Biol Open. 2019:8. doi:10.1242/bio.039677.
- Naija S, Elloumi N, Jbir N, Ammar S, Kevers C. Anatomical and biochemical changes during adventitious rooting of apple rootstocks MM 106 cultured in vitro. C R Biol. 2008;331(7):518–525. doi:10.1016/j.crvi.2008.04.002.
- Welander M, Geier T, Smolka A, Ahlman A, Fan J, Zhu L-H. Origin, timing, and gene expression profile of adventitious rooting in Arabidopsis hypocotyls and stems. Am J Bot. 2014;101(2):255–266. doi:10.3732/ajb.1300258.
- Ma J, Aloni R, Villordon A, Labonte D, Kfir Y, Zemach H, Schwartz A, Althan L, Firon N. Adventitious root primordia formation and development in stem nodes of ‘Georgia Jet‘ sweetpotato, Ipomoea batatas. Am J Bot. 2015;102(7):1040–1049. doi:10.3732/ajb.1400505.
- Owusu Adjei M, Xiang Y, He Y, Zhou X, Mao M, Liu J, Hu H, Luo J, Zhang H, Feng L. Adventitious root primordia formation and development in the stem of Ananas comosus var. bracteatus slip. Bracteatus Slip Plant Signal Behav. 2021;16(11):1949147. doi:10.1080/15592324.2021.1949147.
- Casero PJ, Casimiro I, Lloret PG. Lateral root initiation by asymmetrical transverse divisions of pericycle cells in four plant species: Raphanus sativus, Helianthus annuus, Zea mays, and Daucus carota. Protoplasma. 1995;188:49–58. doi:10.1007/BF01276795.
- Malamy JE, Benfey PN. Organization and cell differentiation in lateral roots of Arabidopsis thaliana. Development. 1997;124(1):33–44. doi:10.1242/dev.124.1.33.
- Yang X, Dai J, Guo D, Zhao S, Huang Y, Chen X, Huang Q. Changes in the properties of Radix aconiti lateralis preparata (Fuzi, processed aconite roots) starch during processing. J Food Sci Technol. 2019;56(1):24–29. doi:10.1007/s13197-018-3430-5.
- Xia Y, Gao W, Jiang Q, Li X, Huang L, Xiao P. Comparison of the physicochemical and functional properties of Aconitum carmichaelii and Aconiti lateralis preparata starches. Starch/Sta¨rke. 2011;63(12):765–770. doi:10.1002/star.201100062.
- Carvalho LJ, Filho JF, Anderson JV, Figueiredo PG, Chen S. Storage root of cassava morphological types, anatomy, formation, growth, development and harvest time. Cassava Viduranga Waisundara Intech Open. 2018. doi:10.5772/intechopen.71347.
- Kitahara K, Nakamura Y, Otani M, Hamada T, Nakayachi O, Takahata Y. Carbohydrate components in sweetpotato storage roots: their diversities and genetic improvement. Breed Sci. 2017;67(1):62–72. doi:10.1270/jsbbs.16135.
- Tang JR, Lu YC, Gao ZJ, Song WL, Wei KH, Zhao Y, Tang QY, Li XJ, Chen JW, Zhang GH. Comparative transcriptome analysis reveals a gene expression profile that contributes to rhizome swelling in panax japonicus var. major. Plant Bio Int J Deal Asp Plant Bio. 2019;154(4):515–523. doi:10.1080/11263504.2019.1651774.
- Wang X, Chang L, Tong Z, Wang D, Yin Q, Wang D, Jin X, Yang Q, Wang L, Sun Y, et al. Proteomics profiling reveals carbohydrate metabolic enzymes and 14-3-3 proteins play important roles for starch accumulation during cassava root tuberization. Sci Rep. 2016;6(1):19643. doi:10.1038/srep19643.
- Aráoz MVC, González AMK, Mercado MI, Ponessa GI, Grau A, Catalán CAN. Ontogeny and total sugar content of yacon tuberous roots and other three Smallanthus species (Heliantheae, Asteraceae), insights on the development of a semi-domesticated crop. Gen Res Crop Evolution. 2013;61(1):163–172. doi:10.1007/s10722-013-0022-0.
- Bombo AB, Oliveira T, Oliveira A, Appezzato-da-Glória VLGR, Appezzato-da-Glo´ria B. Anatomy and essential oil composition of the underground systems of three species of aldama la llave (Asteraceae). J Torrey Bota Soc. 2014;141(2):115–125. doi:10.3159/torrey-d-12-00053.1.
- Silva E, Hayashi AH, Appezzato-da-Glo´ria B. Anatomy of vegetative organs in Aldama tenuifolia and A. kunthiana (Asteraceae: heliantheae). Brazilian J Botany. 2014;37(4):505–517. doi:10.1007/s40415-014-0101-2.
- Zhang N, Zhao J, Lens F, Visser J, Menamo T, Fang W, Xiao D, Bucher J, Basnet RK, Lin K, et al. Morphology, carbohydrate composition and vernalization response in a genetically diverse collection of asian and European turnips (Brassica rapa subsp. rapa). PLoS One. 2014;9(12):e114241. doi:10.1371/journal.pone.0114241.
- Ruan Y-L. Sucrose metabolism: gateway to diverse carbon use and sugar signaling. Annu Rev Plant Biol. 2014;65(1):33–67. doi:10.1146/annurev-arplant-050213-040251.
- Qin M, Li W. Study on development anatomy of root of Aconitum carmichaelii Debx. J China Phar Uni. 1996;027:761–763. [in Chinese].
- Pal T, Malhotra N, Chanumolu SK, Chauhan RS. Next-generation sequencing (NGS) transcriptomes reveal association of multiple genes and pathways contributing to secondary metabolites accumulation in tuberous roots of Aconitum heterophyllum Wall. Planta. 2015;242(1):239–258. doi:10.1007/s00425-015-2304-6.
- Kawasaki R, Motoya W, Atsumi T, Mouri C, Kakiuchi N, Mikage M. The relationship between growth of the aerial part and alkaloid content variation in cultivated Aconitum carmichaelii Debeaux. J Nat Med. 2011;65(1):111–115. doi:10.1007/s11418-010-0466-x.
- Belehu T, Hammes P, Robbertse P. The origin and structure of adventitious roots in sweet potato (Ipomoea batatas). Australian J Bot. 2004;52(4):551–558. doi:10.1071/bt03152.
- Singh V, Zemach H, Shabtai S, Aloni R, Yang J, Zhang P, Sergeeva L, Ligterink W, Firon N. Proximal and distal parts of sweetpotato adventitious roots display differences in root architecture, lignin, and starch metabolism and their developmental fates. Front Plant Sci. 2020;11:609923. doi:10.3389/fpls.2020.609923.
- Villordon AQ, Bonte DRL, Firon N, Kfir Y, Pressman E, Schwartz A. Characterization of adventitious root development in sweetpotato. Hort Science. 2009;44(3):651–655. doi:10.21273/hortsci.44.3.651.
- Togari Y. A study of tuberous root formation in sweet potato. Bul Nat Agr Expt Sta Tokyo. 1950;68:1–96.
- Firon N, LaBonte D, Villordon A, Kfir Y, Solis J, Lapis E, Perlman T, Doron-Faigenboim A, Hetzroni A, Althan L. Transcriptional profiling of sweetpotato (Ipomoea batatas) roots indicates down-regulation of lignin biosynthesis and up-regulation of starch biosynthesis at an early stage of storage root formation. BMC Genomics. 2013;14(1):460. doi:10.1186/1471-2164-14-460.
- Singh V, Sergeeva L, Ligterink W, Aloni R, Zemach H, Doron-Faigenboim A, Yang J, Zhang P, Shabtai S, Firon N. Gibberellin promotes sweetpotato root vascular lignification and reduces storage-root formation. Front Plant Sci. 2019;10:1320. doi:10.3389/fpls.2019.01320.
- Wang G-L, Que F, Xu Z-S, Wang F, Xiong A-S. Exogenous gibberellin enhances secondary xylem development and lignification in carrot taproot. Protoplasma. 2017;254(2):839–848. doi:10.1007/s00709-016-0995-6.
- Figueiredo PG, Moraes-Dallaqua M, Bicudo SJ, Tanamati FY, Aguiar EB. Development of tuberous cassava roots under different tillage systems: descriptive anatomy. Plant Prod Sci. 2015;18(3):241–245. doi:10.1626/pps.18.241.
- Zaki HEM, Takahata Y, Yokoi S. Analysis of the morphological and anatomical characteristics of roots in three radish (Raphanus sativus) cultivars that differ in root shape. J Horti Sci Biotec. 2015;87(2):172–178. doi:10.1080/14620316.2012.11512849.
- Li M, Yang Y, Li X, Gu L, Wang F, Feng F, Tian Y, Wang F, Wang X, Lin W, et al. Analysis of integrated multiple ‘omics’ datasets reveals the mechanisms of initiation and determination in the formation of tuberous roots in Rehmannia glutinosa. J Exp Bot. 2015;66(19):5837–5851. doi:10.1093/jxb/erv288.
- Vreugdenhil XXD, Lammeren A, Lammeren AAMV. Cell division and cell enlargement during potato tuber. J Exp Bot. 1998;49(320):573–582. doi:10.1093/jxb/49.320.573.
- Zierer W, Rüscher D, Sonnewald U, Sonnewald S. Tuber and tuberous root development. Annu Rev Plant Biol. 2021;72(1):551–580. doi:10.1146/annurev-arplant-080720-084456.
- Tong -Y-Y, Xiao P-G, Lou Z-C. A general survey of morphological and histological characters of the Chinese medicinal aconite root. Acta Pharmaceutica Sinica. 1984;19:701–705. [in Chinese].
- Liu -C-C, Cheng M-E, Peng H-S, Duan H-Y, Huang L-Q. Identification of four Aconitum species used as “Caowu” in herbal markets by 3d reconstruction and microstructural comparison. Microsc Res Techniq. 2015;78(5):425–432. doi:10.1002/jemt.22491.
- Ott JP, Klimesova J, Hartnett DC. The ecology and significance of below-ground bud banks in plants. Ann Bot. 2019;123(7):1099–1118. doi:10.1093/aob/mcz051.
- Shang X-M, Z-L L. Study on the chromosomes of ten domestic Aconitum Species. J Sys Evo. 1984;22:378–385. [in Chinese].
- Yu M, Cao -L-L, Yang Y-X, Guan -L-L, Gou -L-L, Shu X-Y, Huang J, Liu D, Zhang H, Hou D-B, et al. Genetic diversity and marker–trait association analysis for agronomic traits in Aconitum carmichaelii Debeaux. Biotech Equip. 2017;31(5):905–911. doi:10.1080/13102818.2017.1355747.
- Jin W, Chen C, Wang E-B. A karytaxonomical study on 6 species of Aconitum in Liaoning area. Bull Bot Res. 1998;18:163–172. [in Chinese].
- Song Y, Qiao Y-G, Wu Y-X. clustering analysis of karyotype resemblance near coefficient of 16 Aconitum L. speicies. ACTA AGRESTIA SINICA. 2012; 20. [in Chinese].
- Yang Q-E. Cytology of 12 species in Aconitum L. and of 18 species in Delphinium L. of the tribe Delphineae (Ranunculaceae) from China. Acta Phytotaxonomica Sinica. 2001;39:502–514.
- Gustafsson A. Polyploidy, life-form and vegetative reproduction. Hereditas. 1948;34(1–2):1–22. doi:10.1111/j.1601-5223.1948.tb02824.x.
- Eckert CG, Lui K, Bronson K, Corradin P, Bruneau A. Population genetic consequences of extreme variation in sexual and clonal reproduction in an aquatic plant. Mol Ecol. 2003;12(2):331–344. doi:10.1046/j.1365-294x.2003.01737.x.
- Van Drunen WE, Husband BC. Evolutionary associations between polyploidy, clonal reproduction, and perenniality in the angiosperms. New Phytol. 2019;224(3):1266–1277. doi:10.1111/nph.15999.