ABSTRACT
Abscisic acid (ABA) is a plant hormone that induces seed dormancy during seed development and inhibits seed germination after imbibition. Although ABA is synthesized in the seed coat (testa), endosperm, and embryo, the physiological roles of the hormone derived from each tissue are not fully understood. We found that the gene encoding an Arabidopsis ABA importer, NPF5.1, was expressed in the seed coat during seed development. Dry seeds of loss-of-function npf5.1 mutants contained significantly higher levels of dihydrophaseic acid (DPA), an inactive ABA metabolite, than the wild type. The npf5.1 mutant also had a slight increase in ABA content. An increase in DPA was prominent in the fraction containing the seed coat and endosperm. Seed germination of the npf5.1 mutant was similar to the wild type in the presence of ABA or the gibberellin biosynthesis inhibitor paclobutrazol. However, a mutation in NPF5.1 suppressed the paclobutrazol-resistant germination of npf4.6, a mutant impaired in an ABA importer expressed in the embryo. These results suggest that ABA uptake into the seed coat mediated by NPF5.1 is important for ABA homeostasis during seed development and for regulating seed germination.
Introduction
The plant hormone abscisic acid (ABA) regulates several physiological processes throughout the plant life cycle.Citation1–3 During vegetative stages, ABA promotes stomatal closure to limit water loss. In addition, ABA regulates the expression of genes that are involved in plant responses to abiotic and biotic stresses. ABA is also required for the induction and maintenance of seed dormancy. These physiological responses are often associated with the changes in endogenous ABA levels. Therefore, it is important to understand how the hormone concentration is regulated through biosynthesis, catabolism (inactivation), and transport.
Recent studies have demonstrated that ABA movement within plants is actively regulated by transmembrane transporters.Citation4–6 Three types of transporter families, namely, the ATP-binding cassette (ABC), NITRATE TRANSPORTER 1/PEPTIDE TRANSPORTER FAMILY (NPF), and Detoxification Efflux Carriers (DTX)/Multidrug and Toxic Compound Extrusion (MATE) proteins, are reported to be involved in ABA transport. In Arabidopsis, ABA synthesized in vascular tissues is released into the apoplastic space by the exporter protein ABCG25 and ultimately reaches guard cells, where the hormone is taken up by importer proteins ABCG40 and NPF4.6.Citation7–9 The amount of ABA transported from vascular tissues to guard cells is regulated by several transporters, such as ABCG17, ABCG18, DTX50, and NPF5.1.Citation8,Citation10,Citation11 In Arabidopsis seeds, ABCG25 and ABCG31 export ABA from the endosperm, whereas ABCG30 and ABCG40 import ABA into embryos.Citation12 These findings indicate that the movement of ABA within plants is regulated in a very complex manner.
In this study, we found that Arabidopsis NPF5.1, which encodes an ABA importer, was expressed specifically in the seed coat. Interestingly, mutants defective in NPF5.1 (npf5.1) accumulated significantly higher levels of dihydrophaseic acid (DPA), an inactive ABA metabolite, in the seed coat and/or endosperm compared to the wild type. Possible functions of NPF5.1 in ABA homeostasis and the regulation of seed germination are discussed.
Materials and methods
Plant materials and growth conditions
Arabidopsis [Arabidopsis thaliana (L.) Heynh.] accession Columbia-0 was used as the wild type. Mutants npf4.6–1 (ait1-1) (SALK_146143), npf5.1–1 (SALK_085919), and npf5.1–2 (SALK_000464) were isolated in previous studies.Citation8,Citation13 Transgenic plants expressing the GUS gene under the control of the NPF5.1 promoter (pNPF5.1:GUS) were generated as described previously.Citation8 Seeds were sterilized with 70% (v/v) ethanol and then with 5% (v/v) NaClO (0.25% active chlorine) containing 1% (w/v) Tween 20. After washing with sterilized water, seeds were sown on plates containing half-strength MS medium and 0.8% (w/v) agar and incubated at 4°C for 3 days in the dark. Plates were then incubated at 22°C under continuous light (50 μ moles/m2/s) for 7 days. Seedlings were transplanted to soil containing vermiculite and Metro-mix 350 (Sun Gro Horticulture) at a 3:1 ratio for seed propagation.
GUS staining
For GUS staining, developing seeds (15 days after flowering) were isolated from the silique envelopes. Seeds were incubated overnight in a solution composed of 50 mM sodium phosphate buffer (pH 7.2), 10 mM EDTA, 0.05% (v/v) Triton X-100, 0.5 mM potassium ferrocyanide, 0.5 mM potassium ferricyanide and 1 mM X-Gluc and then observed using a stereo microscope (Leica M205, Leica).
Germination assays
Surface sterilized seeds were sown on 0.8% (w/v) agar plates containing half-strength MS medium. Stock solutions of (±)-ABA (Sigma-Aldrich) and paclobutrazol (Wako) to be added to the media were prepared in DMSO at 1000× concentrations; DMSO was added to the control medium. Plates were incubated at 4°C in the dark for 3 days and then at 22°C under continuous light. Germination, defined as cotyledon greening, was scored after 4 days (for control and paclobutrazol treatments) or 7 days (for ABA treatment).
Measurements of ABA and ABA metabolites
ABA and ABA metabolites were extracted and purified as described previously.Citation14 ABA and ABA metabolites were quantified using a quadrupole/time-of-flight tandem mass spectrometer (Triple TOF 5600, SCIEX) and a Nexera HPLC system (SHIMADZU) equipped with a ZORBAX Eclipse XDB-C18 column (2.1 × 50 mm, Agilent). Compounds were separated by LC with a binary solvent system of water containing 0.01% (v/v) acetic acid (A) and acetonitrile containing 0.05% (v/v) acetic acid (B) at a flow rate of 0.4 ml/min. The experimental protocol was as follows: constant 3% solvent B from 0 to 0.5 min followed by a linear gradient of solvent B from 3 to 40% over 8.5 min. Retention times of the compounds were 3.24 min for dihydrophaseic acid (DPA) and d3-DPA, 4.52 min for phaseic acid (PA) and d3-PA, 5.93 min for ABA and d6-ABA, and 4.53 min for ABA-glucose ester (ABA-GE) and d5-ABA-GE. MS/MS conditions were as follows: ion spray voltage (kV) = −3.5, desolvation temperature (°C) = 600, declustering potential (V) = −100 (DPA and d3-DPA), −90 (PA and d3-PA, ABA and d6-ABA), −120 (ABA-GE and d5-ABA-GE), collision energy (V) = −20 (DPA and d3-DPA), −16 (PA and d3-PA), −15 (ABA and d6-ABA), −16 (ABA-GE and d5-ABA-GE), scan range (m/z) = 100–500, MS/MS transition (m/z) = 281/171 (DPA), 284/174 (d3-DPA), 279/139 (PA), 282/142 (d3-PA), 263–153 (ABA), 269–159 (d6-ABA), 425–263 (ABA-GE), 430/268 (d5-ABA-GE). Data were analyzed using SCIEX OS-Q 2.0 software (SCIEX).
Results and discussions
We previously reported that the transporter encoded by Arabidopsis NPF5.1 (At2g40460) mediates cellular ABA uptake.Citation8 NPF5.1 is expressed in vascular tissues and leaf mesophyll and epidermal cells during vegetative stages. Mutants defective in NPF5.1 (npf5.1) exhibited higher leaf surface temperatures than in the wild type, suggesting that the protein negatively regulates stomatal closure by limiting the amount of ABA transported from vascular tissues, where the hormone is synthesized, to guard cells. The Arabidopsis eFP Browser (http://bar.utoronto.ca/efp/cgi-bin/efpWeb.cgi)Citation15,Citation16 indicated that NPF5.1 is also expressed in seeds, more specifically in the seed coat, during later stages of seed development. We confirmed the seed-coat specific expression of NPF5.1 at 15 days after flowering by GUS staining of pNPF5.1:GUS seeds (). We hypothesized that NPF5.1 might regulate some important aspects of seed physiology as an ABA transporter.
Figure 1. Expression of NPF5.1 in seeds. Seeds of pNPF5.1:GUS plants at 15 days after flowering were stained for GUS activity. Bar = 0.5 mm.
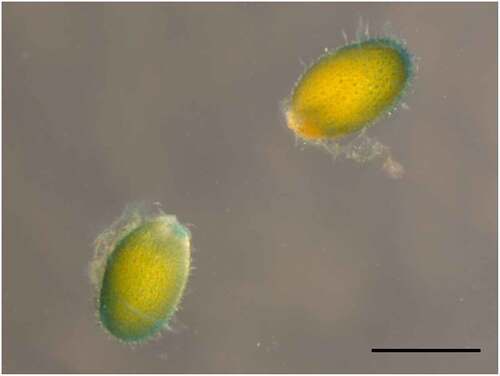
We next examined whether the loss of NPF5.1 functions affects seed germination (). Germination levels of seeds from both npf5.1–1 and npf5.1–2 alleles and the wild type were almost 100% within 4 days after stratification. Although several ABA-deficient and -insensitive mutants have been reported to germinate better than the wild type in the presence of gibberellin (GA) biosynthesis inhibitors such as paclobutrazol,Citation17,Citation18 germination of npf5.1 was inhibited to levels similar to the wild type. The sensitivity of npf5.1 to exogenously applied ABA was also comparable to that of the wild type. In contrast, npf4.6 germinated better than the wild type in the presence of ABA, as we reported previously.Citation13 In addition, npf4.6 was less sensitive to paclobutrazol compared to the wild type. As NPF4.6 is expressed in the embryo,Citation13 ABA uptake into the embryo may contribute significantly to seed sensitivity to exogenously applied ABA and paclobutrazol. Interestingly, a mutation in NPF5.1 suppressed the paclobutrazol-resistant germination phenotype in the npf4.6 mutant background. This observation may occur because ABA uptake into the seed coat mediated by NPF5.1 somehow affected ABA accumulation within the seeds.
Figure 2. Germination of wild type (WT), npf5.1–1, npf5.1–2, npf4.6–1 (npf4.6), and npf4.6–1 npf5.1–1 (npf4.6 npf5.1) on the control medium (Control) or on media containing 50 μM paclobutrazol (Pac) or 0.5 μM ABA. Different letters indicate significant differences (P < .05) by Tukey’s multiple comparison test determined for each group.
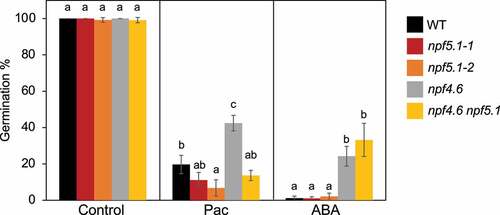
Endogenous levels of ABA and its metabolites in whole dry seeds were analyzed to determine the impact of the npf5.1 mutations on the homeostasis of the hormone (). npf5.1 contained slightly (about 1.3 times) but significantly greater amounts of ABA and ABA-GE than the wild type. In npf4.6, the two compounds were detected at the levels comparable to npf5.1. Additive effects of the npf5.1 and npf4.6 mutations on ABA and ABA-GE content were not observed for unknown reasons. To our surprise, DPA levels were markedly (3–4 times) increased in npf5.1 compared to the wild type. The difference in the DPA content between the two npf5.1 alleles was possibly due to different severities of the mutations: npf5.1–1 contains T-DNA in the second intron, whereas T-DNA is inserted in the 3’ UTR in npf5.1–2. DPA was detected at similar levels in the wild type and npf4.6, indicating that different mechanisms may have caused the increase in ABA and ABA-GE levels observed in npf5.1 and npf4.6.
Figure 3. Endogenous levels of ABA, phaseic acid (PA), dihydrophaseic acid (DPA) and ABA-glucose ester (ABA-GE) in mature dry seeds of wild type (WT), npf5.1–1, npf5.1–2, npf4.6–1 (npf4.6), and npf4.6–1 npf5.1–1 (npf4.6 npf5.1). Different letters indicate significant differences (P < .01) determined by Tukey’s multiple comparison test.
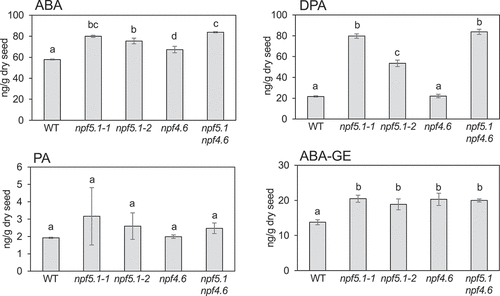
Finally, we determined where the accumulation of ABA and DPA occurred in seeds. Mature seeds were dissected into the embryo and the remaining tissues containing the seed coat and endosperm within 1 h of imbibition, and ABA and DPA were quantified separately from the two fractions (). In npf5.1, slight increases in the ABA levels compared to wild type were observed in the embryos and the testa/endosperm fraction. DPA was accumulated to much higher levels (about 4 times relative to the wild type) in the testa/endosperm fraction of npf5.1. In contrast, the compound present in embryos was only slightly (about 1.3 times) higher in npf5.1 than the wild type.
Figure 4. Comparison of ABA and dihydrophaseic acid (DPA) levels in embryos and the fraction containing seed coats and endosperms (testa/endosperm) between wild type (WT) and npf5.1 (npf5.1–1). Significant differences were determined by Student’s t-test.
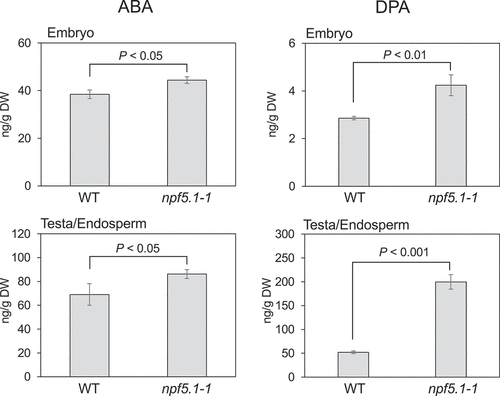
ABA is synthesized in the seed coat, endosperm, and embryo during Arabidopsis seed development.Citation19,Citation20 Crosses between wild-type and ABA-deficient mutants have shown that ABA synthesized in maternal tissues, including the seed coat, is transported to embryos.Citation14,Citation21 Given that NPF5.1 is expressed in the seed coat (), we hypothesized that the NPF5.1 transporter protein plays a role in retaining ABA within seed coats. Loss of NPF5.1 functions would, thus, first result in an increase in the amount of ABA transported from the seed coat to the neighboring tissue, namely the endosperm. One of the genes (CYP707A2) encoding ABA 8’-hydroxylases, the rate-limiting enzyme of ABA catabolism in Arabidopsis, is highly expressed in the endosperm.Citation22 Therefore, ABA transported from the seed coat to the endosperm may be easily converted successively to 8’-hydroxylated ABA, PA, and finally to DPA in npf5.1. Although the increase in ABA levels observed in npf5.1 was not prominent, possibly due to a higher turnover rate, the mutation was sufficient to affect seed germination performances, at least in the npf4.6 background (). Different effects of npf4.6 and npf5.1 mutations on seed germinability and endogenous levels of ABA and its metabolites () support the idea that NPF4.6 and NPF5.1 function at distinct sites (the embryo and seed coat, respectively) and regulate different physiological processes. Quantification of ABA and DPA also suggests that an excess amount of either or both compounds accumulated in the endosperm of npf5.1 is transported to the embryo (). Several ABA transporters that function in seeds have been identified;Citation12 however, it is not fully understood how ABA and ABA metabolites move within seed tissues. Further identification of transporters and the visualization of ABA-related compounds at the tissue or cellular levels are required to answer this question.
Acknowledgments
We thank Dr. Suzanne R. Abrams for providing us with the internal standards of ABA metabolites. We also thank Ms. Tomoe Nose and Masako Tanaka for their technical assistance.
Disclosure statement
No potential conflict of interest was reported by the author(s).
Additional information
Funding
References
- Zeevaart JAD, Creelman RA. Metabolism and physiology of abscisic acid. Annu Rev Plant Physiol Plant Mol Biol. 1988;39(1):439–5. doi:10.1146/annurev.pp.39.060188.002255.
- Nambara E, Marion-Poll A. Abscisic acid biosynthesis and catabolism. Annu Rev Plant Biol. 2005;56(1):165–185. doi:10.1146/annurev.arplant.56.032604.144046. Epub 2005/ 05/03. PubMed PMID: 15862093.
- Finkelstein R. Abscisic Acid synthesis and response. Arabidopsis Book. 2013;11:e0166. doi:10.1199/tab.0166. PubMed PMID: 24273463; PubMed Central PMCID: PMC3833200.
- Anfang M, Shani E. Transport mechanisms of plant hormones. Curr Opin Plant Biol. 2021;63:102055. doi:10.1016/j.pbi.2021.102055. Epub 20210605. PubMed PMID: 34102450.
- Kuromori T, Seo M, Shinozaki K. ABA transport and plant water stress responses. Trends Plant Sci. 2018;23(6):513–522. doi:10.1016/j.tplants.2018.04.001. Epub 2018/ 05/08. PubMed PMID: 29731225.
- Seo M, Marion-Poll A. Abscisic acid metabolism and transport. Adv Bot Res. 2019;92:1–49. doi:10.1016/bs.abr.2019.04.004. PubMed PMID: WOS: 000539033800002.
- Kang J, Hwang JU, Lee M, Kim YY, Assmann SM, Martinoia E, Lee Y. PDR-type ABC transporter mediates cellular uptake of the phytohormone abscisic acid. Proc Natl Acad Sci U S A. 2010;107(5):2355–2360. doi:10.1073/pnas.0909222107. Epub 2010/ 02/06. PubMed PMID: 20133880; PubMed Central PMCID: PMC2836657.
- Shimizu T, Kanno Y, Suzuki H, Watanabe S, Seo M. Arabidopsis NPF4.6 and NPF5.1 control leaf stomatal aperture by regulating abscisic acid transport. Genes (Basel). 2021;12(6):885. doi:10.3390/genes12060885. Epub 20210608. PubMed PMID: 34201150; PubMed Central PMCID: PMCPMC8227765.
- Kuromori T, Miyaji T, Yabuuchi H, Shimizu H, Sugimoto E, Kamiya A, Moriyama Y, Shinozaki K. ABC transporter AtABCG25 is involved in abscisic acid transport and responses. Proc Natl Acad Sci U S A. 2010;107(5):2361–2366. doi:10.1073/pnas.0912516107. Epub 2010/ 02/06. PubMed PMID: 20133881; PubMed Central PMCID: PMC2836683.
- Zhang Y, Kilambi HV, Liu J, Bar H, Lazary S, Egbaria A, Ripper D, Charrier L, Belew ZM, Wulff N, et al. ABA homeostasis and long-distance translocation are redundantly regulated by ABCG ABA importers. Sci Adv. 2021;7(43):eabf6069. doi:10.1126/sciadv.abf6069. Epub 20211020. PubMed PMID: 34669479; PubMed Central PMCID: PMCPMC8528425.
- Zhang H, Zhu H, Pan Y, Yu Y, Luan S, Li L. A DTX/MATE-type transporter facilitates abscisic acid efflux and modulates ABA sensitivity and drought tolerance in arabidopsis. Mol Plant. 2014;7(10):1522–1532. doi:10.1093/mp/ssu063. PubMed PMID: 24851876
- Kang J, Yim S, Choi H, Kim A, Lee KP, Lopez-Molina L, Martinoia E, Lee Y. Abscisic acid transporters cooperate to control seed germination. Nat Commun. 2015;6(1):8113. doi:10.1038/ncomms9113. PubMed PMID: 26334616; PubMed Central PMCID: PMC4569717
- Kanno Y, Hanada A, Chiba Y, Ichikawa T, Nakazawa M, Matsui M, Koshiba T, Kamiya Y, Seo M. Identification of an abscisic acid transporter by functional screening using the receptor complex as a sensor. Proc Natl Acad Sci U S A. 2012;109(24):9653–9658. doi:10.1073/pnas.1203567109. Epub 2012/ 05/31. PubMed PMID: 22645333; PubMed Central PMCID: PMC3386071.
- Kanno Y, Jikumaru Y, Hanada A, Nambara E, Abrams SR, Kamiya Y, Seo M. Comprehensive hormone profiling in developing Arabidopsis seeds: examination of the site of ABA biosynthesis, ABA transport and hormone interactions. Plant Cell Physiol. 2010;51(12):1988–2001. doi:10.1093/pcp/pcq158. PubMed PMID: 20959378
- Winter D, Vinegar B, Nahal H, Ammar R, Wilson GV, and Provart NJ . An ”electronic fluorescent pictograph” browser for exploring and analyzing large-scale biological data sets. PloS One. 2007;2(8):e718. doi:10.1371/journal.pone.0000718. PubMed PMID: 17684564; PubMed Central PMCID: PMC1934936
- Bassel GW, Fung P, Chow TF, Foong JA, Provart NJ, Cutler SR. Elucidating the germination transcriptional program using small molecules. Plant Physiol. 2008;147(1):143–155. doi:10.1104/pp.107.110841. Epub 2008/ 03/25. PubMed PMID: 18359847; PubMed Central PMCID: PMC2330302.
- Leon-Kloosterziel KM, Gil MA, Ruijs GJ, Jacobsen SE, Olszewski NE, Schwartz SH, Zeevaart JAD, Koornneef M. Isolation and characterization of abscisic acid-deficient Arabidopsis mutants at two new loci. Plant J. 1996;10(4):655–661. doi:10.1046/j.1365-313X.1996.10040655.x. Epub 1996/ 10/01. PubMed PMID: 8893542.
- Nambara E, Akazawa T, McCourt P. Effects of the gibberellin biosynthetic inhibitor uniconazol on mutants of Arabidopsis. Plant Physiol. 1991;97(2):736–738. doi:10.1104/pp.97.2.736. Epub 1991/ 10/01. PubMed PMID: 16668460; PubMed Central PMCID: PMC1081068.
- Lefebvre V, North H, Frey A, Sotta B, Seo M, Okamoto M, Nambara, E, and Marion-Poll, A . Functional analysis of Arabidopsis NCED6 and NCED9 genes indicates that ABA synthesized in the endosperm is involved in the induction of seed dormancy. Plant J. 2006;45(3):309–319. doi:10.1111/j.1365-313X.2005.02622.x. Epub 2006/ 01/18. Epub 2006/ 01/18.
- Frey A, Effroy D, Lefebvre V, Seo M, Perreau F, Berger A, Sechet J, To A, North HM, and Marion-Poll A, et al. Epoxycarotenoid cleavage by NCED5 fine-tunes ABA accumulation and affects seed dormancy and drought tolerance with other NCED family members. Plant J. 2012;70(3):501–512. doi:10.1111/j.1365-313X.2011.04887.x. PubMed PMID: 22171989.
- Karssen CM, Brinkhorstvanderswan DLC, Breekland AE, Koornneef M. Induction of dormancy during seed development by endogenous abscisic-acid - studies on abscisic-acid deficient genotypes of Arabidopsis-Thaliana (L) Heynh. Planta. 1983;157(2):158–165. doi:10.1007/BF00393650. PubMed PMID: ISI:A1983QJ30600009.
- Okamoto M, Kuwahara A, Seo M, Kushiro T, Asami T, Hirai N, Kamiya, Y, Koshiba, T, and Nambara, E . CYP707A1 and CYP707A2, which encode abscisic acid 8’-hydroxylases, are indispensable for proper control of seed dormancy and germination in Arabidopsis. Plant Physiol. 2006;141(1):97–107. doi:10.1104/pp.106.079475. Epub 2006/ 03/18. Epub 2006/ 03/18.