ABSTRACT
Plant glutamate receptor homologs (GLRs), which function as key calcium channels, play pivotal roles in various developmental processes as well as stress responses. The moss Physcomitrium patens, a representative of the earliest land plant lineage, possess multiple pathways of hormone signaling for coordinating growth and adaptation responses. However, it is not clear whether GLRs are connected to hormone-mediated growth control in the moss. In this study, we report that one of the two GLRs in P. patens, PpGLR1, involves in abscisic acid (ABA)-mediated growth regulation. ABA represses the growth of wild-type moss, and intriguingly, the PpGLR1 transcript levels are significantly increased in response to ABA treatment, based on both gene expression and the PpGLR1pro::GUS reporter results. Furthermore, the growth of Ppglr1 knockout moss mutants is hypersensitive to ABA treatment. These results suggest that PpGLR1 plays a critical role in ABA-mediated growth regulation, which provide useful information for our further investigation of the regulatory mechanism between Ca2+ signal and ABA in moss growth control.
Introduction
As one of the most primitive and simple non-vascular plants, bryophytes occupy a unique evolutionary position as the intermediate between green algae and vascular plants. The bryophyte P. patens belongs to the Funariaceae and has been used as a typical representative of early terrestrial plants in the past 50 years.Citation1–3 As the first genome-sequenced non-vascular plant, the availability of the whole genome sequence,Citation4 together with efficient gene manipulating techniques, makes P. patens a suitable species for addressing key questions in relation to plant development and stress responses.
Being the earlier land plants in terrestrial conditions, P. patens has to maintain normal growth and cope with complicated environmental changes. Multiple signaling pathways are required to ensure normal plant developmental and adaptation processes,Citation1–3 among which calcium acts as a versatile signaling molecule due to its instant response to endogenous and external stimuli.Citation5,Citation6 Under the stimuli, calcium channels distributed on the cytoplasmic membrane or endomembrane system can be transiently activated, resulting in a fluctuation of cytosolic-free calcium ions, known as Ca2+ signature, and then a further plant growth regulation process.Citation7
Based on sequence homology search of animal calcium channels in the plant genomes, putative candidates of plant calcium channels were identified, mainly including cyclic nucleotide-gated ion channels (CNGCs), glutamate receptor-like channels (GLRs), and calcium permeable stress-gated cation channels/reduced hyperosmolality-induced [Ca2+]i increase channels (CSCs/OSCAs) and so on.Citation8–11 Subsequent studies have shown that these genes function in a variety of Ca2+-dependent physiological or pathological processes.Citation6,Citation7,Citation12,Citation13
Since the discovery of putative GLRs in the model plant Arabidopsis in 1998,Citation8 studies have demonstrated that GLRs involve in various physiological processes in different plant species.Citation7,Citation14 With the advances of studies in molecular genetics, cell biology, and plant physiology, the functions and molecular regulatory mechanisms of plant GLRs have attracted extensive attention, with their roles covering normal developmental programs, such as C/N metabolism, root growth and development, seed germination, stomatal movement, and pollen tube morphogenesis and fertilization,Citation14–21 as well as stress responses.Citation22–26 The report that two GLRs in the moss P. patens, PpGLR1 and PpGLR2, function in sperm tropism and reproduction greatly expands our understanding about the roles of GLRs in the early land plant species.Citation14 However, whether PpGLRs are implicated in the other process is largely unknown. In this study, we report that PpGLR1 is connected to the growth regulation performed by plant hormone abscisic acid (ABA).
Materials and methods
Plant materials and growth conditions
In this study, the P. patens (Hedwig) ecotype ‘Gransden 2004’ is used as the wild-type (WT) moss, and the P. patens glr1 mutant lines were generated by CRISPR/Cas9-mediated gene editing approach in this study. The tissue of WT P. patens and the Ppglr1 mutant was cultured on BCDA (for protonemata) or BCD (for gametophytes) medium supplemented with 0.5% (w/v) glucose and grown at 25°C under 120 μmol m−2 s−1 light with a long-day photoperiod (16-h light/8-h dark). For growth phenotype analysis, the tips of WT gametophytes with uniform size were cultured on BCD medium supplemented with indicated concentrations of the hormone for 7 weeks. The same stage P. patens samples were harvested for reverse transcription-PCR (RT-PCR) analysis. For the expression analysis of PpGLR1 and PpGLR2 in young tissue, gametophore tissues of WT were homogenized gently, and the obtained protonemata was cultured on BCDA medium for two weeks with a second time homogenization in the middle. The protonemata was then transferred to cellophane-overlayed BCD medium for gametophore growth and collected at indicated time points for the expression analysis.
Gene expression analysis
Total RNA of the P. patens tissue was extracted using Spectrum Plant Total RNA Kit (Sigma) and reverse transcribed using iScript cDNA Synthesis Kit (Bio-Rad) after the treatment with DNase I (Ambion). For semi-quantitative RT-PCR analysis of PpGLR1 and PpGLR2 expression in gametophytes, PCR amplifications were performed with 31 cycles for PpGLR1 and 28 cycles for PpGLR2 and Tubulin respectively, using the following gene-specific primers: PpGLR1, 5’-GACCCCAAATTTTCGCAGGCACTC-3’ and 5’-GAGCTGCAGCAGGTAGTCCCGCAC-3’; PpGLR2, 5’-GGACAAAGACTCAGATTTTCGGC-3’ and 5’-GAAGGATCCCGTTTGGTAGCCGATAG-3’; and Tubulin, 5’-GAGTTCACGGAAGCGGAGAG-3’ and 5’-TCCTCCAGATCCTCCTCATA-3’. qRT-PCR was conducted with SYBR Green PCR Master Mix (Bio-Rad) using the CFX96 Real-Time PCR System (Bio-Rad), and relative expression level was normalized to that of Tubulin. Primers used for PpGLR1 and tubulin genes are as follows: PpGLR1, 5’-TTCTTCACGGGTCTAGTAGTGTGG-3’ and 5’-GAGCTGCAGCAGGTAGTCCCGCAC-3’; and tubulin, 5’-GAGTTCACGGAAGCGGAGAG-3’ and 5’-TCCTCCAGATCCTCCTCATA-3’. For the expression analysis, three biological replicates were conducted, and the data presented are from one experimental repetition with three technical repeats.
Generation of the Ppglr1 mutant and P. patens transgenic plants
The Ppglr1 mutant generation and related protoplast preparation and stable transformation were performed using standard PEG-mediated approach. To generate the Ppglr1 mutant lines, we subjected the first exon of PpGLR1 to a search of CRISPR RNA (ATTGTCGCTGGAGGGCTAAC) with the webtool CRISPOR V1 to the P. patens genome Phytozome V9 http://crispor.tefor.net/crispor.py). The sgRNA constructed a 20 bp CRISPR RNA of PpGLR1 fused with 83 bp of the S. pyogenes tracrRNA scaffold under the U6 promoter control as described previously.Citation27 Transformants of the Ppglr1 mutant candidates were selected on BCD medium supplemented with 50 mg/L G418, and confirmed by sequencing of the target PpGLR1 fragment using primers 5’-ACTGAATCGTGGGGTTCGAC-3’ and 5’-GCCCAGATGTAGCACCAACT-3’. To conduct the PpGLR1pro::GUS reporter assay, a 2-kb fragment of the PpGLR1 promoter was amplified from WT genomic DNA with specific primers PpGLR1pro-F 5’-CGGTACCAGGGTGCTAAAGATCACTTTATTTGG-3’ and PpGLR1pro-R 5’-GGGCGCGCCACTTTCCTTGGTACTGTTTACTTCTAAACC-3’, and cloned into pTFH15.3-GUS vector with restriction enzyme sites of KpnI and AscI then performed protoplast transformation. To select transgenic plants of PpGLR1pro::GUS, transformed protoplasts were selected on BCD medium supplemented with 50 mg/L G418. After a period of 6-week regeneration, the candidate lines were examined for GUS signal.
Histochemical GUS activity analysis
GUS activity was examined according to the procedure described.Citation28 Briefly, the PpGLR1pro::GUS transgenic lines were incubated in GUS staining buffer at 37°C in the dark overnight and cleaned with 70% (v/v) ethanol to remove chlorophyll. Samples from control or hormone-treated medium were stained and decolorized at the same time for result comparison. Photographs were taken using a Zeiss Axio Zoom V16 equipped with a Zeiss Axio CamICc digital camera.
Statistical analysis
Student’s t-test (one-tailed distribution) was used to determine the significance of the data.
Results
To examine the effects of plant hormone ABA on the growth of P. patens, we cultured WT P. patens under indicated amounts of ABA and analyzed the growth phenotypes. Compared with control, ABA inhibited P. patens growth in a dose-dependent manner, as shown by the significantly decreased plant size (as shown by the area of gametophyte) and fresh weight (). Compared with ABA treatments, GA treatment caused no visible differences in plant size (Supplementary Figure S1). We next examined whether the two PpGLRs were connected to the hormone-modulated growth control in P. patens, and we detected the expression levels of PpGLR1 and PpGLR2 in the above hormone-treated samples by semi-quantitative RT-PCR analysis. The results showed that the expression levels of PpGLR1 were obviously increased by ABA treatments (). In contrast, the expression levels of PpGLR2 showed no clear changes in response to either of the hormone treatment ( and Supplementary Figure S2), which may imply its more specific roles in the sexual reproduction as reported previously.Citation14 Consistent with the observed ABA-induced PpGLR1 transcript increase pattern (), qRT-PCR results showed a dose-dependent increase of ABA effects on PpGLR1 expression levels (). In line with the above results, the transcript amounts of LATE EMBRYOGENESIS ABUNDANT (PpLEA), a gene known to be involved in ABA response, were greatly increased in the ABA treated samples (), supporting the reliability of our experimental conditions.
Figure 1. The expression analysis of two PpGLRs under different concentrations of ABA in P. patens. (a) Phenotypes of wild-type (WT) P. patens cultured on BCD medium supplemented with indicated concentrations of ABA. Three biological replicates were done, and representative pictures are shown. (b and c) Statistical analyses of the area and fresh weight of the gametophyte under indicated amounts of hormone. Three biological replicates were done, and data shown are mean of thirty samples from one replicate and error bars indicate SD. (d) semi-quantitative RT-PCR analysis of the expression of PpGLR1 and PpGLR2 under indicated concentration of hormone treatment. Tubulin was used as a loading control. (e) qRT-PCR analysis of PpGLR1 and PpLEA expression under different concentrations of ABA. Three biological replicates were done and similar gene expression trends are shown. Data from three technical repeats are shown, and error bars represent SD. In B, C, and E, ** indicates significant differences between control and hormone-treated sample (P < .01).
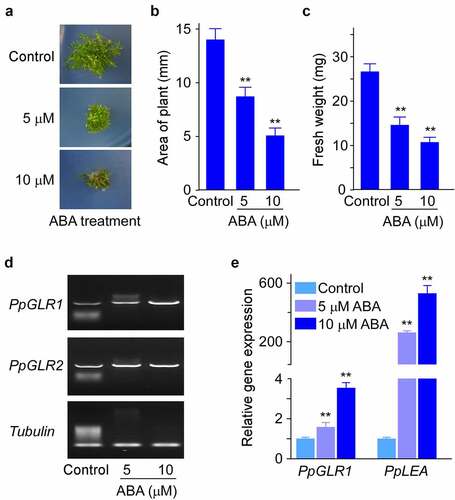
We further characterized the expression sites of PpGLR1 using the PpGLR1pro::GUS transgenic plants. We cloned a 2-kb promoter sequence of PpGLR1, fused it with the GUS reporter gene, and then generated the PpGLR1pro::GUS transgenic plants in P. patens. Histochemical GUS activity analysis showed that PpGLR1 transcript was mainly detected in the phyllids of the gametophytes under normal culture conditions (). In response to 5 or 10 µM ABA treatment, PpGLR1 transcript was highly up-regulated, in the same expression sites as the control (). Compared with the control, no obvious changes of PpGLR1pro::GUS activity were observed under GA treatments (Supplementary Figure S3). Thus, both gene expression and the PpGLR1pro::GUS reporter analysis results demonstrate that the transcript amounts of PpGLR1 increase obviously in response to ABA. The expression level of PpGLR1 increases while the growth of P. patent is repressed by ABA treatments, suggesting that PpGLR1 plays a role in ABA-regulated growth control in P. patens.
Figure 2. PpGLR1pro::GUS reporter analysis of the PpGLR1 expression under ABA treatments. Gametophytes of the PpGLR1pro::GUS transgenic lines were cultured under the control and indicated concentration of the hormone for fifteen days and collected for the analysis. Representative pictures are shown.
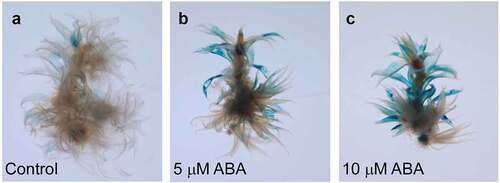
Furthermore, to know more about the physiological roles of PpGLR1 in P. patens, we examined the transcript levels of PpGLR1 in protonema. WT P. patens tissue was collected respectively at four time points (0 d, 3 d, 7 d, and 10 d) after transfer to the new medium, and the expression level of PpGLR1 was analyzed by semi-quantitative RT-PCR. The results showed that PpGLR1 exhibited a first increase and then a decrease trend during the time points detected (Supplementary Figure S4), which may suggest a regulatory role of PpGLR1 in protonema growth regulation of P. patens.
To assess the role of PpGLR1 in ABA-mediated P. patens growth control, we generated Ppglr1 knockout mutants using CRISPR/Cas9 method and tested their phenotypes under ABA treatment. The Ppglr1 mutants contain an 8 bp nucleotide deletion (), and this would lead to the premature termination of the PpGLR1 protein translation. WT and Ppglr1 mutants were grown on BCD medium supplemented with 0 or 5 µM ABA for 7 weeks for phenotype analysis. Under normal conditions, the Ppglr1 mutants were similar to WT in terms of plant size and fresh weight (). In contrast, under ABA treatment, the mutants were smaller and browser than wild type (), with the plant size and fresh weight being reduced to 75% and 45% of the WT, respectively (). These results indicate a correlation between the PpGLR1 expression level and ABA sensitivity in terms of P. patent growth phenotype.
Figure 3. The growth of Ppglr1 mutant was hypersensitive to ABA. (a) The gene structure of PpGLR1 (top) and the sequencing results of the PpGLR1 gene in the Ppglr1 mutant showing an 8 bp deletion which would lead to the premature termination of the PpGLR1 protein translation (bottom). (b) Phenotypes of WT and the Ppglr1 mutant lines under the control and ABA treatment. Gametophytes of WT and Ppglr1 mutants were cultured on BCD medium supplemented with 0 or 5 µM ABA for 7 weeks. Three independent experiments were performed, and representative images are shown. (c and d) Statistical analysis of the diameter and fresh weight of the gametophyte from WT and Ppglr1 mutants under indicated amounts of ABA. Data from thirty samples were analyzed under each treatment, and error bars indicate SD. ** indicates significant differences between WT and Ppglr1 mutant under the same condition (P < .01).
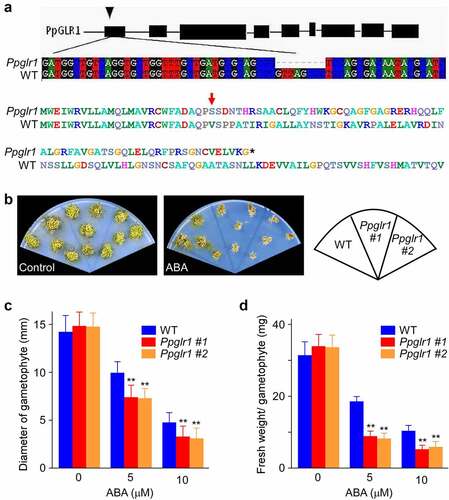
Discussion
Calcium channel-mediated calcium signal plays pivotal roles in plant growth and development.Citation5–7 Increasing evidence has demonstrated that GLR channels function via generating and transducing specific cytosolic calcium signals in different plant species.Citation28,Citation29 A recent study in P. patens showed that two PpGLRs served as key regulators of Ca2+ signal in sperm chemotaxis and transcriptional regulation,Citation14 which extends our knowledge about GLR function to the extremophile land plant. In the current work, through a combination of CRISPR/Cas9-mediated gene editing approach, phenotypic observation, and gene expression analysis, we demonstrate that one of the two PpGLRs, PpGLR1, plays an important role in phytohormone ABA-modulated growth control in the moss.
Compared with the large GLR gene family with big members in seed plants, the genome of the early land plant P. patens contains only two GLRs, PpGLR1 and PpGLR2.Citation29,Citation30 These two PpGLRs therefore may execute relatively conserved function compared to the counterparts in seed plants that have various specialized function. We show that the transcript of PpGLR1 but not PpGLR2 responds to and accumulates under ABA treatment ( and Supplementary Figure S2). Our results, together with the previous finding that PpGLR1 and PpGLR2 showed different spatiotemporal expression pattern,Citation14 indicate that the two PpGLRs have distinct function. The hypersensitive growth phenotype of the Ppglr1 mutant under ABA treatment () suggests that PpGLR1 is a regulator in ABA-mediated developmental process in the moss P. patens. Interestingly, studies have demonstrated that repressing the function of several Arabidopsis GLRs impaired cytosolic Ca2+ concentration and ABA sensitivity,Citation20,Citation31,Citation32 and these Arabidopsis GLRs all belong to subfamily III, one of the three subfamilies divided in Arabidopsis. From a phylogenetic point of view, two PpGLRs are close to the most ancient GLR subfamily III (Clade III) from land plants, particularly in the conserved amino-terminal domain (ATD).Citation29,Citation30,Citation33 Recently, several studies have reported that altering the expression level of GLR changed ABA sensitivity in Arabidopsis.Citation20,Citation31,Citation32 These studies are in line with the results shown in P. patents (, b-d), which together suggests that the regulatory connection between GLR-involved Ca2+ signals and phytohormone ABA-mediated growth exists in moss, the early land plants, and is conserved between P. patens and Arabidopsis. Moreover, our current research might also have indicated an important evolutionary conservation of GLR functional role in plants.
In this study, we show that the Ppglr1 knockout moss mutant is hypersensitive to ABA in growth (), and consistent with our results, a recent report showed that overexpression of Arabidopsis GLR3.7 alleviated ABA sensitivity in germination.Citation32 However, our observation that the transcript of PpGLR1 increased under ABA treatment () is inconsistent with their report showing that ABA treatment suppressed the expression of GLR3.7.Citation32 This divergence could be attributable to differences in the treatment conditions and the GLR member studied. In addition, considering the universal roles of ABA signaling in a variety of stress responses, such as salinity, drought, temperature, and so on, and the cross regulation of plant GLRs in these adversity physiology against environmental menace, we speculate that PpGLRs, especially PpGLR1, may undertake more potential functions responding to adverse environmental stimuli. More related studies in the future would help us better understand the regulatory mechanism between GLR and ABA-mediated physiological process.
Supplemental Material
Download Zip (1.2 MB)Disclosure statement
No potential conflict of interest was reported by the author(s).
Supplementary material
Supplemental data for this article can be accessed online at https://doi.org/10.1080/15592324.2022.2145057
Additional information
Funding
References
- Cove D. The moss physcomitrella patens. Annu Rev Genet. 2005;39(1):339–6. doi:10.1146/annurev.genet.39.073003.110214. PMID: 16285864
- Falz AL, Müller-Schüssele SJ. Physcomitrella as a model system for plant cell biology and organelle-organelle communication. Curr Opin Plant Biol. 2019;52:7–13. PMID:31254720. doi:10.1016/j.pbi.2019.05.007.
- Rensing SA, Goffinet B, Meyberg R, Wu SZ, Bezanilla M. The moss Physcomitrium (Physcomitrella) patens: a model organism for non-seed plants. Plant Cell. 2020;32(5):1361–1376. doi:10.1105/tpc.19.00828. PMID:32152187
- Rensing SA, Lang D, Zimmer AD, Terry A, Salamov A, Shapiro H, Nishiyama T, Perroud P-F, Lindquist EA, Kamisugi Y, et al. The Physcomitrella genome reveals evolutionary insights into the conquest of land by plants. Science. 2008;319(5859):64–69. PMID:18079367. doi:10.1126/science.1150646.
- Kudla J, Becker D, Grill E, Hedrich R, Hippler M, Kummer U, Parniske M, Romeis T, Schumacher K. Advances and current challenges in calcium signaling. New Phytol. 2018;218(2):414–431. doi:10.1111/nph.14966. PMID:29332310
- Tian W, Wang C, Gao QF, Li LG, Luan S. Calcium spikes, waves and oscillations in plant development and biotic interactions. Nat Plants. 2020;6(7):750–759. doi:10.1038/s41477-020-0667-6. PMID:32601423
- Demidchik V, Shabala S, Isayenkov S, Cuin TA, Pottosin I. Calcium transport across plant membranes: mechanisms and functions. New Phytol. 2018;220(1):49–69. doi:10.1111/nph.15266. PMID:29916203
- Lam HM, Chiu J, Hsieh MH, Meisel L, Oliveira IC, Shin M, Coruzzi G. Glutamate-receptor genes in plants. Nature. 1998;396(6707):125–126. doi:10.1038/24066. PMID:9823891
- Leng Q, Mercier RW, Yao W, Berkowitz GA. Cloning and first functional characterization of a plant cyclic nucleotide-gated cation channel. Plant Physiol. 1999;121(3):753–761. doi:10.1104/pp.121.3.753. PMID:10557223
- Hou CC, Tian W, Kleist T, He K, Garcia V, Bai FL, Hao YL, Luan S, Li LG. DUF221 proteins are a family of osmosensitive calcium-permeable cation channels conserved across eukaryotes. Cell Res. 2014;24(5):632–635. doi:10.1038/cr.2014.14. PMID: 24503647
- Yuan F, Yang HM, Xue Y, Kong DD, Ye R, Li CJ, Zhang J, Theprungsirikul L, Shrift T, Krichilsky B, et al. OSCA1 mediates osmotic-stress-evoked Ca2+ increases vital for osmosensing in Arabidopsis. Nature. 2014;514(7522):367–371. doi:10.1038/nature13593. PMID: 25162526
- Frachisse JM, Thomine S, Allain JM. Calcium and plasma membrane force-gated ion channels behind development. Curr Opin Plant Biol. 2020;53:57–64. PMID:31783322. doi:10.1016/j.pbi.2019.10.006.
- Jarratt-Barnham E, Wang L, Ning Y, Davies JM. The complex story of plant cyclic nucleotide-gated channels. Int J Mol Sci. 2021;22(2):874. doi:10.3390/ijms22020874. PMID:33467208
- Ortiz-Ramírez C, Michard E, Simon AA, Damineli DSC, Hernández-Coronado M, Becker JD, Feijó JA. GLUTAMATE RECEPTOR-LIKE channels are essential for chemotaxis and reproduction in mosses. Nature. 2017;549(7670):91–95. doi:10.1038/nature23478. PMID:28737761
- Kang J, Turano FJ. The putative glutamate receptor 1.1 (AtGLR1.1) functions as a regulator of carbon and nitrogen metabolism in Arabidopsis thaliana. Proc Natl Acad Sci USA. 2003;100(11):6872–6877. doi:10.1073/pnas.1030961100. PMID:12738881
- Cho D, Kim SA, Murata Y, Lee S, Jae SK, Nam HG, Kwak JM. De-regulated expression of the plant glutamate receptor homolog AtGLR3.1 impairs long-term Ca 2+-programmed stomatal closure. Plant J. 2009;58(3):437–449. doi:10.1111/j.1365-313X.2009.03789.x. PMID:19143998
- Michard E, Lima PT, Borges F, Silva AC, Portes MT, Carvalho JE, Gilliham M, Liu L-H, Obermeyer G, Feijó JA, et al. Glutamate receptor–like genes form Ca 2+channels in pollen tubes and are regulated by pistil d-serine. Science. 2011;332(6028):434–437. PMID:21415319. doi:10.1126/science.1201101.
- Vincill ED, Clarin AE, Molenda JN, Spalding EP. Interacting glutamate receptor-like proteins in phloem regulate lateral root initiation in Arabidopsis. Plant Cell. 2013;25(4):1304–1313. doi:10.1105/tpc.113.110668. PMID:23590882
- Kong D, Ju C, Parihar A, Kim S, Cho D, Kwak JM. Arabidopsis glutamate receptor homolog AtGLR3.5 modulates cytosolic Ca2+ level to counteract effect of abscisic acid in seed germination. Plant Physiol. 2015;167(4):1630–1642. doi:10.1104/pp.114.251298. PMID:25681329
- Kong D, Hu H-C, Okuma E, Lee Y, Lee HS, Munemasa S, Cho D, Ju C, Pedoeim L, Rodriguez B, Wang J, Im W, Murata Y, Pei ZM, Kwak JM. L-Met activates Arabidopsis GLR Ca2+ channels upstream of ROS production and regulates stomatal movement. Cell Rep. 2016;17(10):2553–2561. PMID:27926860. doi:10.1016/j.celrep.2016.11.015.
- Wudick MM, Portes MT, Michard E, Rosas-Santiago P, Lizzio MA, Nunes CO, Campos C, Santa Cruz Damineli D, Carvalho JC, Lima PT, et al. CORNICHON sorting and regulation of GLR channels underlie pollen tube Ca 2+homeostasis. Science. 2018;360(6388):533–536. PMID:29724955. doi:10.1126/science.aar6464.
- Meyerhoff O, Muller K, Roelfsema MRG, Latz A, Lacombe B, Hedrich R, Dietrich P, Becker D. AtGLR3.4, a glutamate receptor channel-like gene is sensitive to touch and cold. Planta. 2005;222(3):418–427. doi:10.1007/s00425-005-1551-3. PMID:15864638
- Li F, Wang J, Ma CL, Zhao YX, Wang YC, Hasi A, Qi Z. Glutamate receptor-like channel3.3 is involved in mediating glutathione-triggered cytosolic calcium transients, transcriptional changes, and innate immunity responses in Arabidopsis. Plant Physiol. 2013;162(3):1497–1509. doi:10.1104/pp.113.217208. PMID:23656893
- Mousavi SAR, Chauvin A, Pascaud F, Kellenberger S, Farmer EE. GLUTAMATE RECEPTOR-LIKE genes mediate leaf-to-leaf wound signaling. Nature. 2013;500(7463):422–426. doi:10.1038/nature12478. PMID:23969459
- Toyota M, Spencer D, Sawai-Toyota S, Jiaqi W, Zhang T, Koo AJ, Howe GA, Gilroy S. Glutamate triggers long-distance, calcium-based plant defense signaling. Science. 2018;361(6407):1112–1115. doi:10.1126/science.aat7744. PMID:30213912
- Li M, Wang F, Li S, Yu G, Wang L, Li Q, Zhu X, Li Z, Yuan L, Liu P. Importers drive leaf-to-leaf jasmonic acid transmission in wound-induced systemic immunity. Mol Plants. 2020;13(10):1485–1498. PMID:32889174. 10.1016/j.molp.2020.08.017.
- Chen L, Bao F, Tang S, Zuo E, Lv Q, Zhang D, Hu Y, Wang X, He Y. PpAKR1A, a novel Aldo-Keto reductase from Physcomitrella patens, plays a positive role in salt stress. Int J Mol Sci. 2019;20(22):5723. doi:10.3390/ijms20225723. PMID: 31739643
- Jefferson RA, Kavanagh TA, Bevan MW. GUS fusions: b-glucuronidase as a sensitive and versatile gene fusion marker in higher plants. EMBO J. 1987;6(13):3901–3907. doi:10.1002/j.1460-2075.1987.tb02730.x. PMID: 3327686
- Forde BG, Roberts MR. Glutamate receptor-like channels in plants: a role as amino acid sensors in plant defence? F1000Prime Rep. 2014;6:37. PMID:24991414. doi:10.12703/P6-37.
- Wudick MM, Michard E, Oliveira C, Feijó JA. Comparing plant and animal glutamate receptors: common traits but different fates? J Exp Bot. 2018;17(17):4151–4163. doi:10.1093/jxb/ery153. PMID:29684179
- Cheng Y, Zhang X, Sun T, Tian Q, Zhang WH. Glutamate receptor homolog3.4 is involved in regulation of seed germination under salt stress in Arabidopsis. Plant & Cell Physiol. 2018;59(5):978–988. doi:10.1093/pcp/pcy034. PMID:29432559
- Chen PY, Hsu CY, Lee CE, Chang IF. Arabidopsis glutamate receptor GLR3.7 is involved in abscisic acid response. Plant Signal Behav. 2021;12(12):1997513. doi:10.1080/15592324.2021.1997513. PMID:34763610
- De Bortoli S, Teardo E, Szabò I, Morosinotto T, Alboresi A. Evolutionary insight into the ionotropic glutamate receptor superfamily of photosynthetic organisms. Biophys Chem. 2016;218:14–26. PMID:27586818. doi:10.1016/j.bpc.2016.07.004.